Fig. 3.1
Schematic cartoon of an atherosclerotic lesion and events in the process of atherogenesis (Adapted and reprinted from (Schenkein and Loos 2013))
Ultimately, a disintegrated endothelial lining (6) can rupture, with exposition of the underlying atherosclerotic plaque. This generates thrombin from prothrombin, which in turn enzymatically generates fibrin from fibrinogen, initiating the clotting cascade and resulting in thrombosis and subsequently to a myocardial infarction, ischemic stroke, acute peripheral artery disease, or cardiac death.
This slow and chronic process over years of atherogenesis may be mediated by repeated bacteremia, a pro-inflammatory state and activated inflammatory cells, from infectious and inflammatory processes elsewhere (Andersen and Jess 2014; Koenig 2013). In this respect, Crohn’s disease and ulcerative colitis, chronic periodontitis, but also rheumatoid arthritis have been implicated (Andersen and Jess 2014; Garcia-Gomez et al. 2014; Kholy et al. 2015).
Bacteria (transmigrated from the subgingival biofilm into the periodontal lesion or in the circulation itself) can activate platelets (7; out of scale within Fig. 3.1) and can trigger platelet–leukocyte complexes. These can form aggregates on the dysfunctional endothelium, in particular due to the adhesion molecules that are abundantly expressed, resulting in the initiation of (micro) thrombus formation.
Simultaneously, the liver (8) is stimulated and upregulated due to inflammatory signals (IL-6), to overproduce clotting factors, resulting in a prothrombotic state. Also increased production of acute phase reactants, including CRP, which will increase the pro-inflammatory state, are produced in the liver (8; again out of scale within Fig. 3.1).
Within the progressive atherosclerotic lesion, decreased collagen production and activation of MMPs (9) is occurring, resulting in reduction of the smooth muscle cell content and increased degradation of remaining collagen that borders the fibrous cap, weakening the strength of the vessel, leading to fissuring of the atheroma.
In a severely progressed stage (10), the atheroma comprises of a large necrotic core which is exposed to the vasculature within the lesion, leading to contact with platelets and initiation of coagulation and plaque rupture in so-called vulnerable lesions.
3.3 Bacteremia and Consequences for the Cardiovascular System
It has been known for many years that oral bacteria can enter the bloodstream. In particular, in cases of severe gingivitis and periodontitis, the inner epithelial lining of the periodontal pockets is ulcerated and shows loss of epithelial lining integrity (Schenkein and Loos 2013; Loos 2005; Tonetti and Graziani 2014). This ulcerated pocket epithelium may add up to 8–20 cm2 (Loos 2005; Nesse et al. 2008). It is called periodontal inflamed surface area and forms an easy porte d’entrée for the bacteria, bacterial toxins, and other antigenic components residing in the subgingival area (Loos 2005; Nesse et al. 2008). Many of the subgingival bacteria are gram negative, and in particular, the endotoxin lipopolysaccharide (LPS) from these species stimulates the host immune system. This latter biologic event can be at the basis of the pro-inflammatory state seen in both periodontitis and cardiovascular disease (see below) (Schenkein and Loos 2013; Tonetti and Graziani 2014). Also the daily episodes of dissemination of bacteria from the periodontal lesions into the bloodstream can favor atheroma formation and can induce activated platelets and platelet–leukocyte complexes, leading to the prothrombotic state (see below).
It is now well documented that bacteria and endotoxins from periodontal lesions enter the bloodstream (Schenkein and Loos 2013; Tonetti and Graziani 2014; Reyes et al. 2013). This has been shown when patients chew, brush their teeth, but also after dental procedures. However, the long-standing periodontal lesions and the chronic daily short-lived bacteremias are mostly responsible for the negative effects. The possibilities for oral and periodontal bacteria to cause harm for the cardiovascular system and to be involved in atherogenesis and atherosclerosis are summarized in Table 3.1.
Table 3.1
Lines of evidence for the role of bacteria and specifically periodontal pathogens and atherosclerosis (Tonetti and Graziani 2014)
Evidence for the role of periodontal pathogens in atherosclerosis
|
---|
DNA of periodontopathogens has been localized within atherosclerotic plaques
|
Periopathogens are able to invade endothelial cells, in particular P. gingivalis
|
Periopathogens and other oral bacteria may trigger platelet aggregation
|
P. gingivalis can accelerate the transition of macrophages to foam cells
|
Periopathogens can activate the host immune system and cause a pro-inflammatory state
|
Interestingly, some indirect evidence for a role of bacteria in atherogenesis or in atherosclerotic lesions in general is the finding of a large variety of bacterial signatures in atherosclerotic biopsies (Ott et al. 2006). Specifically, in addition to a high variety of species, also P. gingivalis, A. actinomycetemcomitans, and other periodontal pathogens have been identified (Table 3.1). In periodontitis, the prevalence of bacteremias and endotoxinemias is higher than in gingivitis or periodontal healthy subjects as evidenced by higher microbial diversities in atherosclerotic biopsies from periodontal patients. Also, there are correlations between the presence of bacterial antigens and molecular signatures in the atherothrombotic lesions and the severity of periodontitis. Moreover, there are correlations reported between the composition of the subgingival microflora and the bacterial species in vascular biopsies. Even, interestingly, there is suggestion that some bacterial species are still viable.
Taken together, there is biological evidence that bacteria and their toxic components, such as LPS, may easily gain access to the vascular system in cases of periodontitis. And biological experiments have demonstrated that this phenomenon plays a role in atherogenesis and exacerbation of atherosclerotic lesions.
3.4 Immunologic Reactions
The increased bacteremias and dissemination of endotoxins (LPS) and many other microbiological antigens stimulate the immune response of the host (Schenkein and Loos 2013). Consequently, an activated innate immunity may result in hyperactive neutrophils, higher levels of complement, increased levels of acute phase reactants (see below), and a general exacerbation of reactivity.
Also the adaptive immunity is activated. The latter is an intriguing finding and the consequences are not well understood. It is found that there is an increased titer of antibodies to oral and periodontal bacteria in the circulation in patients with periodontitis. These antibodies first of all can enter atherosclerotic lesions where such oral species are nested. This can exacerbate such atherosclerotic lesions and could increase the endothelial dysfunction and increase the risk of rupture of the lesion with thrombus formation as a result.
Furthermore, enhanced T- and B-cell activation may give rise to autoimmunity. Autoantibodies may be generated through the molecular mimicry between bacterial and human heat shock proteins (HSP). These cross-reactive antibodies in the first place generated against circulating microorganisms may react, for example, with HSPs on endothelial cells. These become dysfunctional and again this can enhance the chance for rupture of the endothelial lining giving rise to thrombus formation with the chance of ischemia. Anti-HSP antibodies against the periodontal pathogens P. gingivalis, F. nucleatum, T. forsythia, and A. actinomycetemcomitans have been found. Also the activated T and B cells against other antigens of these bacteria have been found. Further, other autoantibodies include anticardiolipin and anti-low-density lipoproteins (anti-LDL-type cholesterol) (Schenkein and Loos 2013; Schenkein et al. 2004).
3.5 Pro-inflammatory State
It has been widely accepted that low-grade systemic inflammation contributes to an elevated risk for CAD and ischemic stroke (Friedewald et al. 2009; Andersen and Jess 2014; Garcia-Gomez et al. 2014; Pearson et al. 2003; Koenig 2013; Emerging Risk Factors Collaboration et al. 2010; Ridker 2009). In the last two decades, C-reactive protein (CRP) has been proposed as an important inflammatory biomarker related to acute atherosclerotic events. CRP levels exhibit a continuous association with the risk of CAD, ischemic stroke, and vascular mortality (Emerging Risk Factors Collaboration et al. 2010). Interestingly, lowering of CRP levels after statin therapy is associated with a decrease in cardiovascular disease event rate, equivalent to what was observed for patients who achieved lowered LDL cholesterol treatment goals (Ridker et al. 2005).
CRP is an “acute-phase reactant,” a molecule produced mainly in the liver, in response to inflammatory signals, in particular in reaction to elevated levels of interleukin-6 (IL-6). The acute-phase reactants such as CRP have pro-inflammatory properties. The exact function(s) of CRP, despite its years of discovery as biomarker, is not yet completely uncovered. CRP is a pentatrexin molecule; it functions as soluble pattern recognition molecule, and one of the most important roles for this protein is host defense primarily against pathogenic bacteria. It can function as an opsonin for pathogens through activation of the complement pathway and through binding of Fc-gamma receptors. CRP and other pentatrexins can also recognize membrane phospholipids and nuclear components exposed on or released by damaged endothelial cells. In this way, it is proposed to be important for clearance of damaged cells and tissues. Because CRP is a strong acute-phase reactant, it is widely used as a diagnostic marker for acute inflammation and/or infection, with levels ranging 10–200 mg/l or even higher.
The levels of CRP related to chronic inflammation and proposed as cardiovascular risk biomarker are relatively low and not exceeding 10 mg/L. These levels have been found by applying a high sensitivity test using nephelometry and therefore often referred to as hsCRP. Levels of hsCRP are classified in three categories with regard to risk for cardiovascular events (Table 3.2) (Pearson et al. 2003).
Table 3.2
Risk levels for acute cardiovascular events based on plasma hsCRP levels
Risk for acute cardiovascular events
|
Levels of hsCRP
|
---|---|
Low
|
<1 mg/L
|
Intermediate
|
1–3 mg/L
|
High
|
>3 mg/L
|
In periodontitis, consistently elevated levels of hsCRP have been found (Paraskevas et al. 2008). Meta-analysis of published case-control studies have shown that in periodontitis often CRP levels are in the range of intermediate to high CVD risk, while the values in controls are in the low- or intermediate-risk category. The latter authors reported that the weighted mean difference between patients and controls was highly significant (1.56 mg/L). Moreover, it has been shown that hsCRP behaves in a dose-dependent manner: severe periodontitis in general shows higher blood plasma levels of hsCRP than moderate or mild periodontitis, while healthy controls show even lower values.
Furthermore, a meta-analysis on periodontitis treatment studies (n = 23) including 1647 patients have shown that hsCRP levels are significantly reduced after periodontal therapy (overall a mean reduction of 0.5 mg/L) (Teeuw et al. 2014). The reduction was specifically significant in a subgroup of periodontitis patients having comorbidities such as diabetes, atherosclerosis, or rheumatoid arthritis. The reduction of hsCRP in periodontitis treatment studies is clinically relevant since the hsCRP levels exhibit a continuous association with the risk of CAD, ischemic stroke, and vascular mortality (Emerging Risk Factors Collaboration et al. 2010); the hsCRP levels after periodontal treatment can often place patients in a low CVD risk category (Table 3.2). These treatment studies with favorable results suggest further that periodontitis is causally related to elevated CRP.
Next to hsCRP are other markers of inflammation in the circulation of patients with periodontitis. Also these can make periodontitis patients more susceptible for an atherosclerotic event as they have atherogenic potential (Schenkein and Loos 2013). These include other acute-phase reactants and immune mediators: interleukin (IL)-1, IL-4, IL-6, IL-18, haptoglobin, serum amyloid A, alpha 1 anti-chymotrypsin, tumor necrosis factor-alpha, metalloproteinase (MMP)-9, platelet-activating factor (PAF), and PAF acetylhydrolase.
The pro-inflammatory state as characterized by the elevated levels of hsCRP, and other biomarkers mentioned above, could enter the blood circulation in essentially two ways:
1.
The spill over from periodontal lesions: There is substantial literature providing indications that inflammatory cytokines and other biomarkers are produced in the periodontal lesion; from here they are “dumped” or “spilled” into the blood circulation. These molecules could impact organs and tissues, such as blood vessels with/without atherosclerotic lesions and the liver.
2.
The activated liver: The liver in turn initiates an acute-phase response. The liver produces higher levels of CRP, and other acute-phase reactants, but also higher levels of complement molecules and prothrombotic molecules, such as fibrinogen, von Willebrand factor, and plasminogen.
In summary, through the clearly proven pro-inflammatory state in periodontitis, there is a highly plausible biologic mechanism why periodontitis is linked to atherosclerosis and acute atherosclerotic events. Excessive local production of pro-inflammatory cytokines, as well as higher levels of these and acute-phase reactants produced in the liver, can induce or exacerbate inflammatory changes in the endothelium and atherosclerotic lesions (Fig. 3.1).
3.6 Prothrombotic State
In recent years, there is a growing body of evidence that in periodontitis both a hypercoagulable state and hypofibrinolysis exist (Schenkein and Loos 2013; Tonetti and Graziani 2014). Collectively, we call this a prothrombotic state, i.e., an individual may form faster than normal a (small) thrombus and/or this is less efficiently removed. It is hypothesized that this state in periodontitis could also be one of the mechanisms as to how periodontitis is associated with ischemic atherosclerotic events. In Box 3.2, we summarize the normal blood clotting events.
Box 3.2. The Normal Blood Clotting Events
In response to vascular injury, a sequence of coordinated events ensures blood fluidity while preventing blood loss. Adhesion, activation, and aggregation of platelets are all steps in primary hemostasis. Under static or low shear conditions of flow, platelets circulate in the inactivated discocyte form. Upon vascular or tissue injury, collagen matrix exposure and local adenosine diphosphate (ADP) production result in agonist–receptor interactions (GPIb-von Willebrand factor, GPVI-collagen, P2Y12-ADP), which conclude with formation of platelet aggregates.
Formation of the platelet hemostatic plug is followed by true blood clot formation (activation of coagulation) and, finally, by clot dissolution (fibrinolysis). Activation of coagulation is strongly dependent on upregulation of tissue factor (TF) and leads to thrombin generation. TF is not normally present in the circulation. Normal hemostasis arises when the blood vessels are disrupted allowing blood to contact extravascular cells expressing TF. Thrombin is active in the conversion of soluble fibrinogen to insoluble fibrin (deposited in the formed blood clot) and is a potent platelet activator. There are three natural anticoagulant mechanisms acting together to prevent thrombosis: (I) the heparin–antithrombin system, (II) the protein C pathway, and (III) the tissue factor pathway inhibitor system.
Fibrinolysis is a natural response to coagulation, leading to fibrin clot breakdown. During fibrinolysis, plasmin is generated from plasminogen (an inactive proenzyme) under the influence of tissue plasminogen activator (tPA) or urokinase plasminogen activator (uPA); this reaction is inhibited by plasminogen activator inhibitor 1 and 2 (PAI-1, PAI-2) and α2-antiplasmin. The formed plasmin cleaves fibrin into soluble degradation products.
In periodontitis, the normal hemostasis state and events may be disturbed. In Table 3.3, we summarized the findings. One of the very first parameters found to be elevated in periodontitis was fibrinogen (Kweider et al. 1993). Fibrinogen is a member of the acute-phase protein family. Its concentration rises in acute and chronic inflammation mainly as result of increased production by hepatocytes. Increased concentrations of fibrinogen are associated with the development of atherothrombotic disease. Several cohort and population-based studies have documented an increased level of plasma fibrinogen in periodontitis patients compared to periodontally healthy individuals (Kweider et al. 1993; Papapanagiotou et al. 2009; Wu et al. 2000). Studies on the effects of periodontal therapy on fibrinogen levels (reviewed in (Teeuw et al. 2014; D’Aiuto et al. 2013)) yielded variable results; some studies reported a significant reduction in the fibrinogen levels in the patient groups that received active periodontal treatment; others measured no significant changes post-therapy.
Table 3.3
Changes or abnormalities in hemostasis parameters observed in periodontitis
Hemostasis parameters
|
Situation in periodontitis
|
---|---|
Biomarkers of coagulation
|
|
Fibrinogen
|
↑
|
Fragment 1 + 2
|
↑
|
von Willebrand factor
|
↑
|
P-selectin
|
↑
|
Abnormalities of platelets
|
|
Numbers in the circulation
|
↑
|
Size (mean platelet volume)
|
↑
|
Activation state
|
↑
|
Reactivity
|
↑
|
Abnormalities of fibrinolysis
|
|
tPA
|
↓
|
PAI-1
|
↑
|
D-dimer
|
↑
|
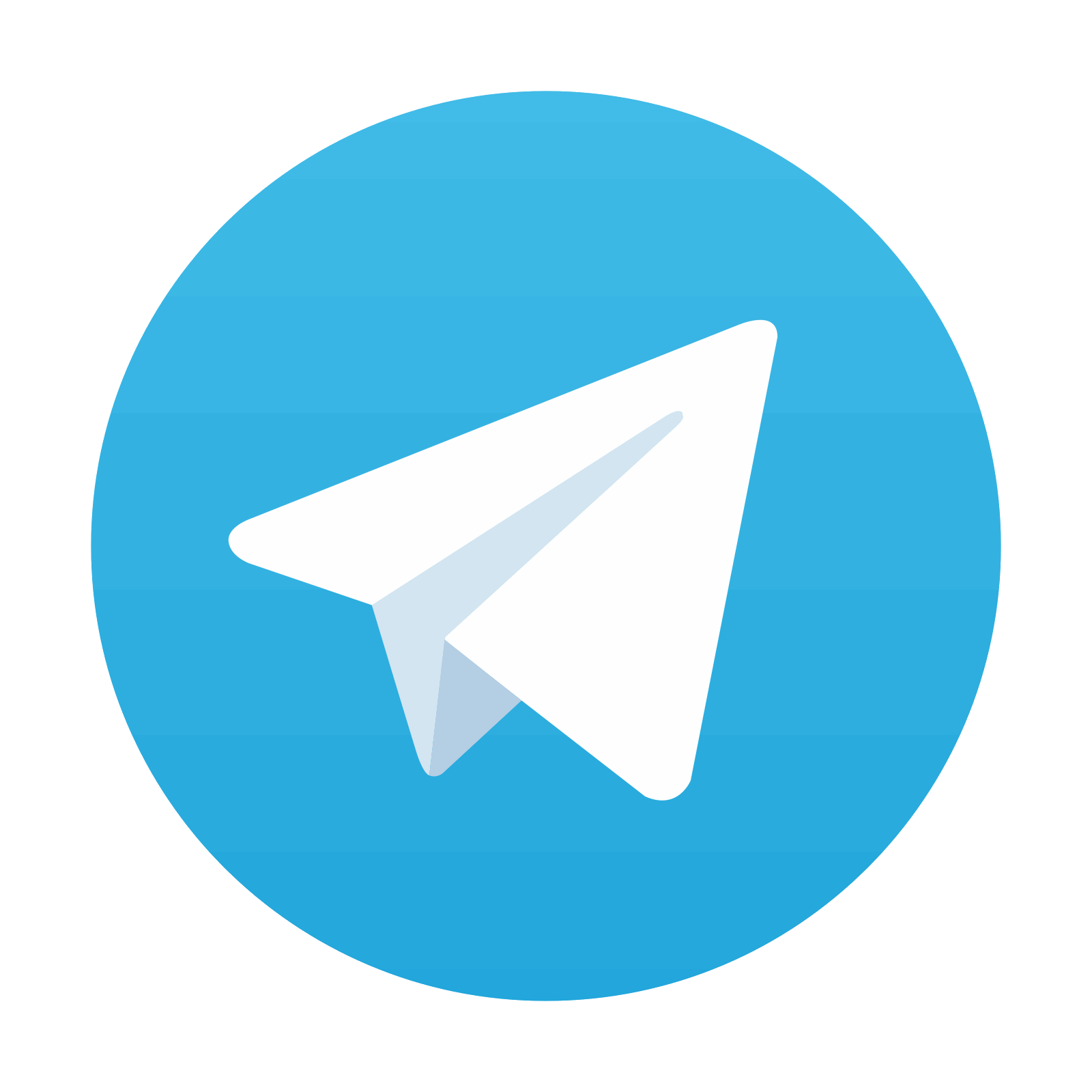
Stay updated, free dental videos. Join our Telegram channel

VIDEdental - Online dental courses
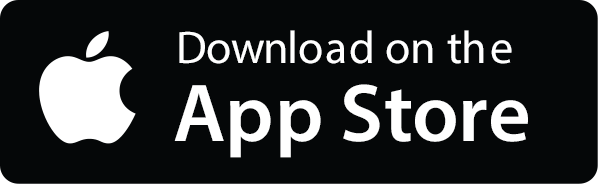
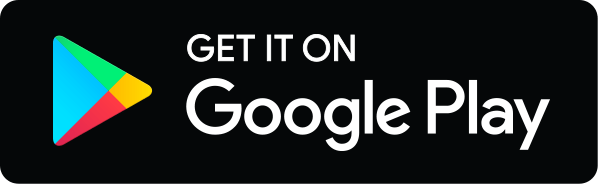