Microbiological tests
pSS
sSS
References
Tongue smear
33 %
76 %
Sota-Rojas et al. (1998)
Tongue swab culture
52 %
76 %
Sota-Rojas et al. (1998)
Saliva culture/oral rinse
76 %
79 %
Sota-Rojas et al. (1998)
81 %
67 %
Kindelan et al. (1998)
65 %
60 %
Almståhl et al. (1999)
72 %
48.1 %
Leung et al. (2007)a
Supragingival plaque
84 %
55.6 %
Leung et al. (2007)a
Numbers of CFU/ml
Tongue/palate swab culture
3.1 × 106 (mean)
1.2 × 105
Rhodus et al. (1997)
Saliva culture
419/μl (mean)
739/μl
Sota-Rojas et al. (1998)
>104 (35.7 %)
>104 (39.1 %)
Ergun et al. (2010)
Oral rinse
2100 (median)
1710
Kindelan et al. (1998)a
380 (median)
500
Almståhl et al. (1999)b
1025.5 (mean)
155
Leung et al. (2007)a
Supragingival plaque
1.8 × 106/g (mean)
0.4 × 106
Leung et al. (2007)a
The microbial samples proving the presence of Candida species on the oral mucosa have usually been obtained by smears or culture swabs taken from the dorsum of the tongue, the buccal or palatal mucosa, the right tonsillar area and/or the fitting surface of the denture (Almståhl and Wikström 1999; Leung et al. 2008; MacFarlane and Mason 1974; MacFarlane 1984; Pedersen et al. 2002a, b; Rhodus et al. 1997; Soto-Rojas et al. 1998; Tapper-Jones et al. 1980). Results revealed that patients with SS have a significant higher mucosal colonisation of C. albicans than healthy subjects (Leung et al. 2008; MacFarlane 1984; Radfar et al. 2003; Rhodus et al. 1997; Soto-Rojas et al. 1998; Tapper-Jones et al. 1980; Yan et al. 2011) and pSS compared with patients with oral lichen planus (Pedersen et al. 2002a, b). Patients with sSS harboured higher numbers of C. albicans (CFU 3.1 × 106) than patients with pSS (CFU 1.2 × 105), which was attributed to the presence of an additional inflammatory disease in sSS as well as to low saliva flow rates (Rhodus et al. 1997). The presence and density of C. albicans were also inversely correlated to saliva flow rates (Hernandez and Daniels 1989; Radfar et al. 2003; Rhodus et al. 1997; Tapper-Jones et al. 1980). Hernandez and Daniels (1989) found that patients with SS and with chronic erythematous candidiasis were older and had long duration of oral symptoms, more inflammation in their labial salivary glands and lower stimulated parotid flow rates than SS patients without this oral lesion. In contradiction to oral rinses, not all mucosal cultures correspond to the clinical signs and symptoms. Thus, MacFarlane (1984) found that 73 % of the patients with pSS had clinical signs of oral candidiasis, although cultures obtained from the dorsal part of the tongue only were positive in 52 % of the cases. This difference between clinical signs and results of Candida cultures may reflect difficulties in obtaining representative material from the dry mucosa (Lundström and Lindström 1995; Soto-Rojas et al. 1998). Furthermore, patients with Candida infection do not necessarily exhibit oral lesions which can be attributed to an asymptomatic carrier status or early candidiasis without clinical apparent lesions or a less virulent strain of Candida. Regarding site specificity, it is noteworthy that C. albicans was found twice as frequent in the supragingival plaque than on the tongue in patients with pSS, but could not be detected in the gingival crevicular region using the paper point technique (Almståhl et al. 2001b).
9.2.3 Other Microorganisms
A sparse number of studies have examined the bacteria in oral mucosal cultures of patients with SS. MacFarlane and Mason (1974) found significantly higher numbers of Staphylococcus aureus and coliform bacilli in SS patients without clinical signs of inflammation than in healthy subjects. Veillonella species, Neisseria pharyngis, Micrococcus mucilaginosus, S. salivarius and S. aureus have also been isolated in higher numbers from the tongue, palate, throat and dentures of patients than in healthy subjects (MacFarlane 1984). However, Almståhl and Wikström (1999) found no differences in the numbers of S. aureus and enterics between patients with pSS and healthy subjects, and the numbers of S. salivarius, Neisseria pharyngis and Veillonella species were lower in pSS patients. Regarding site specificity, a higher density of streptococci, S. salivarius, Fusobacterium nucleatum, Prevotella intermedia, Prevotella nigrescens and S. aureus has been demonstrated on the dorsal part of the tongue than on the buccal mucosa and in the vestibulum (Almståhl et al. 2001b). Species usually associated with gingivitis such as F. nucleatum, P. intermedia and P. nigrescens were found in slightly lower levels in the gingival crevice region of the patients with pSS, but in higher levels of the sSS patients, than in control subjects (Almståhl and Wikström 1999). In this regard, it is noteworthy that the susceptibility to gingivitis and periodontitis has not been found increased in patients with pSS compared to healthy controls (Boutsi et al. 2000; Kuru et al. 2002; Pedersen et al. 1999b; Schiødt et al. 2001; Tseng et al. 1990), which is supported by the rare detection of Porphyromonas gingivalis and Actinobacillus actinomycetemcomitans in the gingival crevice region of patients with pSS (Almståhl et al. 2001b). However, increased levels of antibodies to A. actinomycetemcomitans and Porphyromonas gingivalis, but not to P. intermedia, in addition to an increased incidence of periodontal disease have been reported in patients with SS (Çelenligil et al 1998; Ergun et al. 2010), but they did not discriminate between pSS and sSS, and it is likely that patients with sSS display an increased risk of periodontal diseases due to its concomitant presence with rheumatoid arthritis (for further details on rheumatoid arthritis and periodontitis, see Chap. 4).
9.3 Oral Microbiota in Patients Receiving Chemotherapy
During cancer chemotherapy, there is a significantly increased risk of oral infections due to the immunosuppressive effect and the direct cytotoxic effect of the drugs on oral epithelial barrier function. Studies have shown that chemotherapy may induce temporary salivary gland hypofunction, although there is some controversy whether it is caused by the chemotherapy per se or by other factors, e.g. concomitant intake of xerogenic medication like antiemetics (Jensen et al. 2010). Salivary secretion may even be lower in individuals with cancer prior to the initiation of treatment (Harrison et al. 1998; Napeñas et al. 2013). Chemotherapy can induce compositional changes in saliva. Decreased saliva flow rates combined with the finding of slightly increased salivary sodium and chloride concentrations as well as decreased inorganic phosphate concentration suggest that salivary gland acinar secretion and duct modification mechanisms are impaired by cancer chemotherapy (Jensen et al. 2008a). The concentration and output of secretory IgA has been found to decrease both during and following chemotherapy (Harrison et al. 1998; Jensen et al. 2008a; Laine et al. 1992; Main et al. 1984; Meurman et al. 1997a) and the concentration of lysozyme to decrease after chemotherapy (Meurman et al. 1997a). The salivary peroxidase system is impaired during chemotherapy due to a lower concentration of thiocyanate and its oxidised form hypothiocyanite with antibacterial properties (Mansson-Rahemtulla et al. 1992). Findings on saliva pH and buffer capacity are inconsistent and have been shown to decrease, be unchanged or with regard to buffer capacity even be increased in response to cancer chemotherapy (Avsar et al. 2007; Jensen et al. 2008a; Nemeth et al. 2014; Pajari et al. 1989; Schum et al. 1979). Thus, salivary gland hypofunction, changed composition and reduced output of antimicrobial substances may impair the oral host defence against microorganisms, thus individuals in cancer chemotherapy may be more susceptible to oral infections.
9.3.1 Dental Caries
Increased amount of dental bacterial plaque, gingival inflammation and increased salivary counts of caries-related bacteria, mutans streptococci and lactobacilli have been found during and after chemotherapy (Avsar et al. 2007; Jensen et al. 2008b). However, other studies have shown that the salivary concentrations of S. mutans and lactobacilli may decrease during chemotherapy in spite of salivary gland hypofunction. This could be ascribed to the concomitant use of antibiotics, antifungals and/or chlorhexidine mouth rinses during chemotherapy as well as cytotoxic effect of the chemotherapy itself (Meurman et al 1997b; O’Sullivan et al. 1993). S. mutans has been found to be sensitive to daunorubicin, a cytotoxic antibiotic used in chemotherapeutic regimens (O’Sullivan et al. 1993). Along this line, a study showed that salivary S. mutans counts decreased, whereas lactobacilli counts increased during chemotherapy (Meurman et al. 1997b). Other studies have not revealed any changes in the composition of the oral microbiota (Bergmann 1991; O’Sullivan et al. 1993; Wahlin and Holm 1988), but an initial doubling of the concentration of microorganisms concomitant with a transient decrease of stimulated whole saliva flow rate during chemotherapy (Bergmann 1991). A study of the supra- and subgingival dental plaque in adult acute leukaemia patients during chemotherapy found that the percentage of total viable counts of S. mutans in supragingival dental plaque increased and the percentage in subgingival dental plaque decreased (Reynolds et al. 1989). However, the percentage of viridans streptococci (S. mutans not specified) has also been shown to be lower in the supragingival dental plaque of children with acute leukaemia during chemotherapy than in healthy individuals (Sixou et al. 1998).
As chemotherapy is a time-limited treatment and caries is a process that progresses relatively slowly, it may be debatable whether it is possible to assess an increased progression rate of caries during chemotherapy. One study found that 5 years after chemotherapy, salivary counts of S. mutans and lactobacilli were on the same low levels as baseline values before chemotherapy (Meurman et al. 1997b). Another study found no significant correlation between salivary immunoglobulin levels in stimulated whole saliva and S. mutans or Lactobacillus counts in long-term (6 months to 10 years) event-free paediatric patients treated for childhood malignancies by chemotherapy (Dens et al. 1995). The salivary immunoglobulin level was within normal limits, but there was a negative correlation between secretory IgA concentration and caries prevalence (DMFT/dmft), although only significant in some age groups. In bone marrow transplant patients, a significant decrease in stimulated whole saliva flow rate, lower buffer capacity and a change in the oral microbiota towards higher salivary counts of S. mutans and Lactobacillus have been observed during and after chemotherapy and transplantation (Dahllof et al. 1997; Dens et al. 1996). However, stimulated saliva flow rates reached normal values 1 year after cancer treatment, and no significant differences in caries prevalence were found between bone marrow-transplanted children receiving chemotherapy and healthy children 4 years following treatment, but all participants also underwent preventive dental care (Dahllof et al. 1997). In other paediatric populations, significantly more caries, poor oral hygiene and significantly lower stimulated whole saliva flow rate have been found following childhood chemotherapy (Alberth et al. 2004; Avsar et al. 2007; Nemeth et al. 2014; Pajari et al. 1995).
9.3.2 Oral Candidiasis during andAfter Chemotherapy
The oral yeast counts and especially the prevalence of Candida species may increase significantly from a prevalence of about 50 % in the normal population to 73 % in cancer patients during chemotherapy, and the weighted prevalence of clinical oral fungal infection has been estimated to be 38 % (Lalla et al. 2010). C. albicans is the predominant yeast in the oral microbiota during chemotherapy and accounts for up to 88 % of the salivary yeasts (Samaranayake et al. 1984). Other potential virulent Candida species may colonise the oral cavity during cancer treatment with weighted prevalence of 16.6 % for Candida tropicalis, 5.5 % for Candida glabrata and 3 % for Candida krusei (data pooled for chemotherapy and radiation therapy) (Lalla et al. 2010).
Clinical candidiasis and angular cheilitis have been found to correlate to higher oral yeast counts and low saliva flow rates (Wahlin and Holm 1988; Wahlin 1991). A follow-up study found that salivary yeast counts remained high in spite of normal saliva flow rates 5 years after chemotherapy for lymphoma (Meurman et al. 1997b). The salivary concentrations of s-IgA, IgG, IgM and lysozyme in stimulated whole saliva were concomitantly found to be significantly decreased as compared to baseline values (Meurman et al. 1997a). These findings suggest that the disease itself or the chemotherapy may affect the body defences against Candida in the long term.
9.4 Oral Microbiota in Patients Receiving Radiation Therapy
Radiotherapy (RT) of tumours in the head and neck region often includes the major and minor salivary glands in the radiation field depending on the anatomical location and the extension of the tumour. RT can cause severe salivary gland hypofunction (Jensen et al. 2003, 2010). The severity of salivary gland hypofunction depends on the volume of salivary gland tissue included in the radiation field and on the total radiation dose (Vissink et al. 2010). RT targets cells with a rapid mitotic turnover like tumour cells and damages the DNA thereby leading to cell death. Acinar salivary gland cells are radiosensitive in spite of their slow mitotic turnover (Berthrong 1986), and the serous cells appear to be more sensitive to radiation than the mucous ones (Kashima et al. 1965). Radiation damage to the salivary glands may be seen as early as 1 week after initiation of RT (Dreizen et al. 1977) and results in both acute and long-term effects characterised by reduced saliva flow rates, high saliva viscosity and changes of saliva composition. During RT, saliva flow rates decrease and may even reach immeasurable levels (Vissink et al. 2010). With decreasing flow rates, the salivary pH drops and the buffer capacity decreases both during and after RT (Jensen et al. 2003; Valdez et al. 1993). RT also affects the salivary antimicrobial components. The salivary concentrations of IgA and IgG, lactoferrin, lysozyme and peroxidase have been shown to increase during RT due to acute tissue destruction, but after RT they decrease due to reduced functioning of the glands (Brown et al. 1976; Jensen et al. 2003; Makkonen et al. 1986). In the long term, recovery of salivary gland function is dependent on the total radiation dose that the tissue has received. Thus, saliva flow rates may remain severely decreased, and the compositional changes may persist in response to the decrease in saliva flow rates (Jensen et al. 2003, 2010). The standard therapeutic radiation dose for head and neck carcinoma amounts to a total dose of 60–70 Gy. The major salivary glands may have the potential to gradually recover within 1–2 years if gland-sparing radiation regimens have been applied, for example, intensity-modulated radiation therapy, and if it has been achievable to keep the radiation dose to the gland tissue below thresholds of ~26 Gy to the parotid gland and ~39 Gy to the submandibular gland (Murdoch-Kinch et al. 2008; Vissink et al. 2010). A compensatory increase in saliva flow rate from salivary glands not included in the radiation field may be seen (Eisbruch et al. 2001).
9.4.1 Dental Caries
Irradiation-induced salivary gland hypofunction is associated with a shift of the normal oral microbiota increasing the risk to develop rampant dental caries (Vissink et al. 2003). Higher levels of S. mutans and Lactobacillus species are often observed in the oral cavity during and after RT compared to preradiation levels (Brown et al. 1975, 1978; Keene and Fleming 1987; Llory et al. 1972; Schwarz et al. 1999; Vuotila et al. 2002). Oral colonisation with S. mutans has been found lower and stimulated saliva flow rates higher at the end of treatment in patients receiving unilateral RT as compared to bilaterally irradiated patients (Beer et al. 2002). In oral rinses, it has been demonstrated that the predominant acid-producing species of the oral microbiota may change from S. sanguis, S. mitis and S. salivarius before RT to S. mitis, S. salivarius and lactobacilli with a concomitant decrease in saliva flow rates after RT (Tong et al. 2003). The acid-sensitive S. sanguis appears to be inhibited by the more acidic oral environment after RT (Tong et al. 2003). The decrease in the presence of S. sanguis after RT has been shown in other studies (Brown et al. 1975, 1978). Vuotila et al. (2002) found unchanged levels of S. mutans after RT as compared to preradiation levels. Interestingly, a study found no difference in the microbial diversity, composition or number of colony-forming bacterial units (mutans streptococci and lactobacilli), nor did they find a difference in the stimulated whole saliva flow rate, saliva pH and buffering capacity when comparing caries-free and caries-active irradiated nasopharyngeal patients (Zhang et al. 2015).
RT patients suffering from impaired saliva secretion are to be considered a high-risk group regarding dental caries as the oral environment favours acidogenic and acidophilic species.
9.4.2 Oral Candidiasis
The number of yeasts increases in the oral cavity of cancer patients with hyposalivation due to RT, and oropharyngeal candidiasis is a frequent complication during and after RT for head and neck cancer (Lalla et al. 2010). The weighted prevalence of oral candidiasis during head and neck RT has been estimated to be 37 % (Lalla et al. 2010). The increase in the oral yeast colonisation is observed during RT, and the colonisation level remains elevated after RT. The weighted prevalence of oral fungal colonisation during radiation therapy has been estimated to be 75 % (Lalla et al. 2010). C. albicans is the predominant yeast species associated with oral candidiasis in RT patients (Redding et al. 1999; Thaweboon et al. 2008). However, other Candida species are frequently isolated from the oral cavity in RT and may cause oral candidiasis, e.g. C. glabrata, C. tropicalis, C. parapsilosis and C. krusei (Bulacio et al. 2012; de Freitas et al. 2013; Lalla et al. 2010; Schelenz et al. 2011). It has been shown that the increase in C. albicans in oral rinses is positively related to the radiation dose and the volume of parotid gland tissue included in the radiation field (Rossie et al. 1987). A direct correlation between the increase in C. albicans in saliva and reduced saliva flow rates during RT has also been shown (Epstein et al. 1998; Karbach et al. 2012). The increased colonisation of oral yeasts in RT patients in combination with salivary gland hypofunction emphasises the importance of optimal oral hygiene in RT patients due to the risk of developing oropharyngeal candidiasis (Lalla et al. 2010).
9.5 Medication-Induced Salivary Gland Dysfunction and Oral Microbiota
The most common cause of salivary gland dysfunction is the intake of prescribed medications (Handelman et al. 1986; Närhi et al. 1992; Smidt et al. 2010, 2011; Villa et al. 2015; Österberg et al. 1984). Xerostomia is a common complaint, especially in elderly people above the age of 65 years, and is often reported as an adverse effect of medications. The prevalence is ranging from 11 to 72 % (Smidt et al. 2011; Desoutter et al. 2012). Xerostomia is defined as the subjective feeling of oral dryness (Fox et al. 1987; Shetty et al. 2012), and salivary gland dysfunction denotes changes in the quantity and/or quality of saliva (Villa et al. 2015). More than 75 % of adults aged 65 and older take at least one prescription medication (Chrischilles et al. 1992; Smidt et al. 2010). Xerostomia has been associated with 80 % of the most commonly prescribed medications, and several of them have adverse effects directly on the mechanisms responsible for saliva secretion (Smith and Burtner 1994; Sreebny 2010; Smidt et al. 2011; Villa et al. 2015; Aliko et al. 2015). Regardless of the type of medication, saliva flow rates have been shown to decrease as the number of medications increases also known as polypharmacy (Thorselius et al. 1988; Närhi et al. 1992; Smidt et al. 2010). Xerostomia is not only associated with a decrease in the salivary flow rate, it may also be attributed to a change in quality of the saliva (Crogan 2011; Ekström et al. 2012; Tabak 1995). The duration of medication intake also affects the saliva flow rates and the prevalence of xerostomia (Navazesh et al. 1996; Thomson et al. 2006). Navazesh et al. (1996) found that unstimulated and stimulated whole saliva flow rates were significantly lower in adults who had been taking medication for more than 2 years as compared to those who had been taking medication for less than 2 years.
The mechanisms by which medications can influence salivary secretion and cause salivary gland dysfunction are complex, and this is also reflected in the variation and severity of oral complications. It is believed that medications can interact with the salivary secretory reflex at several sites (Ekström et al. 2012; Proctor 2015; Sreebny 2010; Villa et al. 2015). Thus some medications act at the level of the central nervous system such as antidepressants (both tricyclic and non-tricyclic), opioids, sedatives, anxiolytics and decongestants (pseudoephedrine). Also antihypertensives acting on central alpha-2 adrenergic receptors (e.g. clonidine) have been shown to reduce salivary secretion in humans (Proctor 2015; Sreebny 2010). Other medications like anticholinergics for overactive bladder, antiemetics, tricyclic antidepressants, serotonin reuptake inhibitors, certain neuroleptics, antihistamines and antihypertensives (alpha-1 adrenergic and beta-adrenergic blocking agents) are acting at the peripheral level of the neuro-glandular junction interfering with cholinergic muscarinic (M3), adrenergic, peptidergic and/or histaminergic receptor systems (for reviews Proctor 2015; Sreebny 2010). Antidepressants, i.e. inhibitors of serotonin and noradrenaline transporters responsible for reuptake, appear to cause salivary gland dysfunction through activation of alpha-2 adrenergic receptors by elevating endogenous levels of noradrenaline. Both centrally and peripherally acting medications include tricyclic antidepressants, the serotonin reuptake inhibitors, some neuroleptics and antihistamines (Clemmesen 1988; Del Vigna de Almeida et al. 2008; Hunter and Wilson 1995; Proctor 2015; Sreebny 2010). Furthermore, some medications like diuretics indirectly influence the salivary secretion by affecting the electrolytes and water homeostasis (Nederfors et al. 1989). Finally, a large number of factors influence the effect of medications on salivary secretion, such as the dose and the absorption and excretion rates of the drug as well as drug interactions (Del Vigna de Almeida et al. 2008).
9.5.1 Dental Caries
A relatively limited number of studies have investigated the association between medication-induced salivary gland dysfunction and changes in the oral microbiota. A cross-sectional study demonstrated that root caries was more prevalent in those taking antihypertensives than in control subjects (Streckfus et al. 1990). Also the whole saliva flow rates have been found lower and the levels of mutans streptococci and lactobacilli higher in patients taking antihypertensives compared to control subjects (Nonzee et al. 2012). A study on 848 community-dwelling elderly people in South Australia found only a moderate association between medication intake and root caries experience (Thomson et al. 1995). However, a more detailed analysis on the various drugs revealed that patients who took antidepressants and antiulcer agents had a significantly higher root caries index and a 5-year follow-up study including 528 community-dwelling elderly South Australians did not reveal a strong association between intake of medication and caries, apart from intake of antiasthmatics. Along this line, Ryberg et al. (1991) found that long-term treatment with β2-adrenoceptor agonists in patients with asthma was associated with an impaired saliva secretion, which was followed by an increased incidence of dental caries and higher number of DMS (Decayed-Missing-Surfaces) after 4 years of follow-up. These findings were supported by Alaki et al. (2013), who also found higher levels of mutans streptococci and lactobacilli in asthmatic patients taking antiasthmatics more than three times a day compared with other asthmatic patients. A recent study showed that patients on diuretics had a higher prevalence of xerostomia, periodontitis, dental caries and mucosal lesions than control subjects (Prasanthi et al. 2014
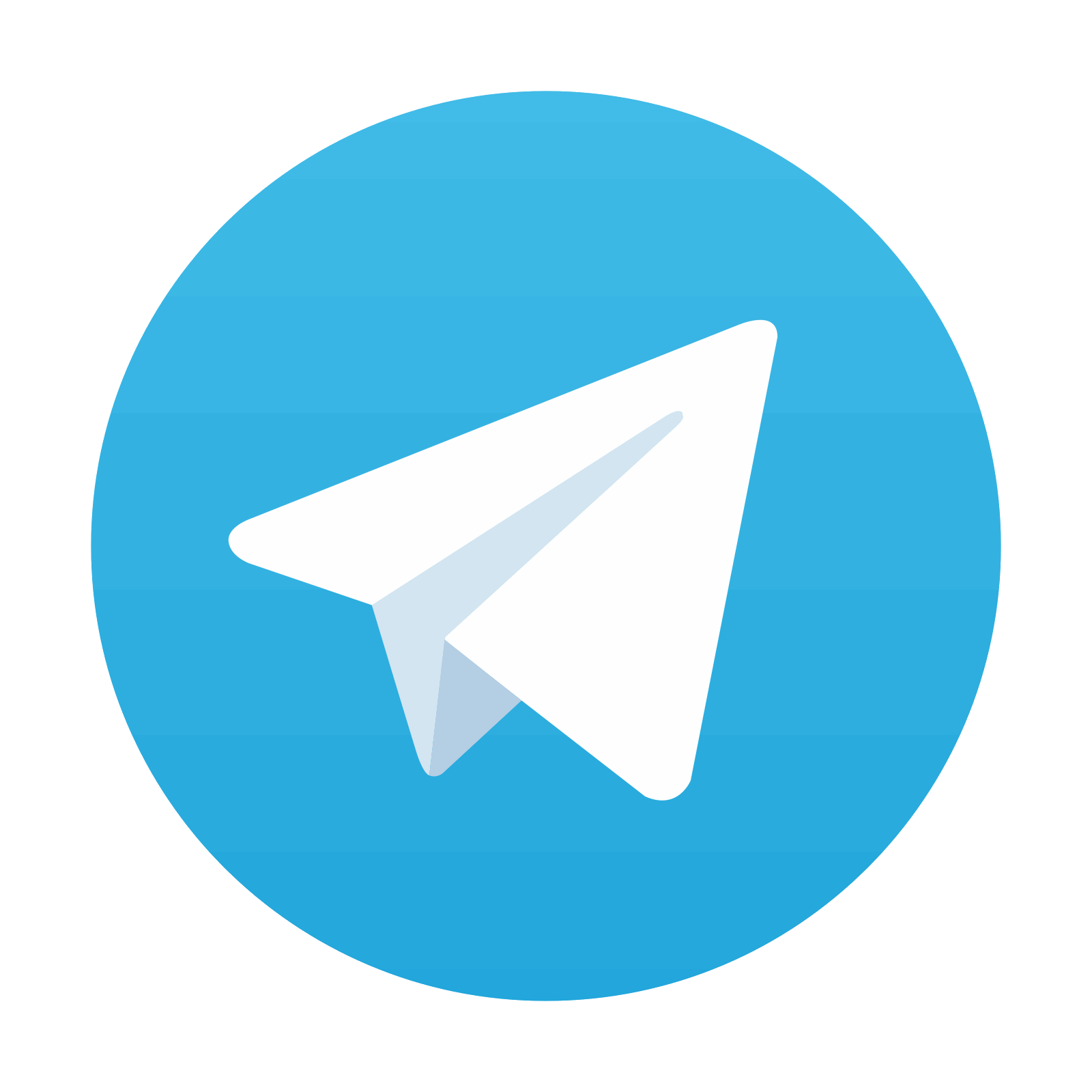
Stay updated, free dental videos. Join our Telegram channel

VIDEdental - Online dental courses
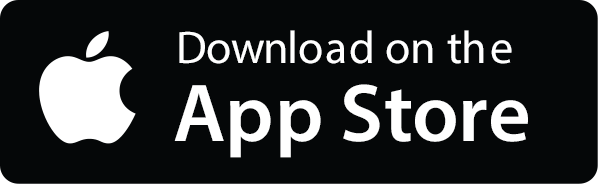
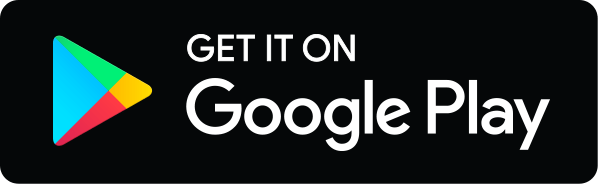