2
Digital Impressions
Brian J. Goodacre, Charles J. Goodacre, Sarah E. Goodacre, and Gary D. Hack
2.1 Introduction
All of us, whether we like it or not, have been thrust into the digital age. We have moved from the rotary telephone to the cell phone, from television sets that were eighteen inches deep to televisions that are now less than an inch thick. Moreover, global positioning systems have replaced paper maps, and communicating by the written hand has morphed into email or texting. Computer power has exploded to the extent that the computing capability of our current cell phones is greater than that of all of the computing power used on the first space flights to the moon by National Aeronautics and Space Administration (Kaku 2011). Today’s students have been raised in this digital world.
Digital technology has also profoundly impacted the dental profession. Significant progress has been made in computerized digital technologies, such as digital cast scanners, intraoral digital impression‐capture devices, cone beam computed tomography, three‐dimensional (3D) printers, laser sintering units, and milling machines (Beuer et al. 2008). It is predicted that in the not‐too‐distant future, the art of making conventional impressions will become nonessential. Just as students today have grown up knowing the internet, the new generation of dentists entering dental schools will learn to practice dentistry using intraoral scanners to make their dental impressions.
Consider the fact that dental X‐ray film has been replaced with digital radiography and cone beam technology as detailed in this chapter. Photographic film, for patient documentation, has evolved into the digital memory card. The manually written appointment book has been transformed into practice management software, and dental advertising has moved from paper to the Web. Digital impressions or optical scans are beginning to overtake the use of conventional dental impressions. Whether we realize it or not, much of what is received from the laboratory is fabricated using digital dentistry. Major changes are now taking place in dental laboratories as a result of these new digital technologies. Laboratories are digitally scanning dies, stone casts, and conventional impressions and designing restorations digitally on computers. Dental laboratories are eliminating wax and wax spatulas. The technology is such that now anything you might expect a dental laboratory to produce can be done digitally and therefore more consistently, quickly, and inexpensively (Touchstone et al. 2010; Van Noort 2012).
It is not only interesting to know where we are going, but it is important to understand from where we have come. Digital imaging for making dental impressions is not novel. The concept began in 1980 with Dr. Werner Mörmann of Zurich, Switzerland. Dr. Mörmann, in collaboration with Dr. Marco Brandestini, began experiments in the machining of dental restorations a century after the introduction of fired porcelain into restorative dentistry. By December of 1980, Dr. Mörmann obtained the first patent in dental scanning. Two years later, in 1982, the first handheld intraoral 3D scanner was developed, but it required further development. By 1983, using the Principle of Active Triangulation, the first optical impression of an inlay was successfully fabricated chairside, and in 1985, the first functional 3D camera for intraoral imaging was introduced. This first camera CEREC technology (Sirona Bersheim, Germany) used an infrared light emitting diode as the light source (Mörmann et al. 1987). This was called the RedCam, which was replaced in 2009 with the BlueCam that scanned using a blue light with 25 μm precision. Today, we have real‐time, full‐color, and high‐definition video scanning with the introduction of Sirona’s OmniCam (2012) and, more recently, the Primescan (2019).
While the concept of imaging/scanning teeth digitally has not yet exceeded conventional impression making in dentistry, it is anticipated that digital scanning of teeth will continue to grow until it does eventually become the predominant method of acquiring an impression. Computer‐Assisted Design/Computer‐Assisted Manufacturing (CAD/CAM) systems can now not only acquire digital impressions/scans, but some systems allow chairside milling of dental restorations using in‐office milling units. The rapid expansion and incorporation of CAD/CAM into the field of dentistry has been documented since the beginning of the 1990s (Miyazaki et al. 2009).
The development of CAD/CAM is based on three elements: data acquisition, data processing, and manufacturing. These elements can now be used to produce a digital cast that can be used to design and fabricate restorations such as crowns and both fixed and removable partial denture frameworks. A further development in CAD/CAM technology is the transition from closed to open access systems, which allows access to a much wider range of manufacturing techniques such that the most appropriate manufacturing processes and associated materials can be selected (van Noort 2012). A scanned image of a prepared tooth or digital impression can greatly exceed the value of a conventional impression, as will be discussed. This chapter will focus primarily on data acquisition with some reference to processing and manufacturing.
2.2 Benefits of Digital Impressions
Changing from the existing conventional/analog impression technique to a digital impression can be an intimidating undertaking, and therefore, it is important to understand what benefits this change will provide and how it compares to the traditional process. In every facet, technology makes our lives easier, better, and more efficient. Digital impressions offer the same benefits to dental workflows.
To achieve excellence in dentistry, it is important to be able to visualize quality, and there are limitations when using conventional elastomeric impressions. When examining conventional impressions, a negative image of a tooth preparation is observed. It is difficult, if not sometimes impossible, to critically evaluate the quality of a preparation simply by looking at its negative appearance. When using elastomeric materials, it is only possible to accurately evaluate certain characteristics such as the finish line and the presence of voids or tears. But some unanswerable questions remain. For instance, did the tray flex or distort, did the tray adhesive perform adequately, or did the impression material pull away from the tray? It is difficult for the clinician to critically evaluate all aspects of the preparation until the impression is poured in stone and a positive cast is produced.
With a conventional impression, failure to record all the surfaces requires making another analog impression. With a digital impression, it is possible to simply trim away the area that is missing and rescan that area. Reich et al. (2021) compared the “cut out‐rescan” procedure of three different intraoral scanners and found there is not a significant influence on the accuracy of complete‐arch scans. This same procedure can be used if the finish line is not completely captured by “locking” the surface. This process prevents new scanned data from being added to the existing scan. For example, if there is a missing portion of the finish line, the entire surface can be locked and then the missing finish line trimmed from the scan. The retraction cord can be placed in that location alone, removed, and the missing portion rescanned. While this procedure was shown to be accurate with complete‐arch scans, it is unknown if it is accurate at the level of a finish line. However, based on the results of Reich et al. (2021), it would appear to fall within a clinically acceptable level, but further research is needed for validation.
The digital impression has significantly improved the ability to immediately evaluate tooth preparations. Having the capability of acquiring a color 3D scan of the prepared tooth and visualizing it on a computer monitor eliminates problems associated with conventional impressions. When evaluating a conventional impression, there is dependence on the intensity of light and degree of magnification. When viewing a digital impression on a computer screen, a true representation of the preparation is seen along with the surrounding structures, and not simply a negative representation as produced with a conventional impression.
With digital impressions, the size of the image seen on the computer screen is significantly larger than what can be seen intraorally, even with loupes, and thereby the visualization process is enhanced (Figure 2.1). This visualization enables dentists to see, in exquisite detail, any imperfections in their preparations, while patients are still in the chair and anesthetized. The quality of the preparation/scan can be easily analyzed compared with evaluating a conventional impression or visualizing difficult‐to‐see areas intraorally. Factors such as preparation total occlusal convergence, quality of finish lines, undercuts, inter‐occlusal clearance, and path of draw/placement can be corrected, if necessary, and a new digital impression made within seconds. In essence, the dentist is receiving immediate feedback on their preparation, and corrections can be made quickly, accurately, and efficiently. No additional appointments are required to remake impressions and the patient is spared the inconvenience of additional anesthesia, cord placement, and re‐provisionalization.

Figure 2.1 Use of the zoom feature to evaluate intraoral scan.
Some intraoral scanners can assist the clinician in shade selection. Scanners with this feature require frequent color calibration using custom color calibration blocks. With proper calibration, intraoral scanners have been a proven and reliable visual method of determining shade and are comparable to spectrophotometers (Liberato et al. 2019). However, some studies have reported concerns with the ability of intraoral scanners to reproduce an accurate shade (Ebeid et al. 2020; Yoon et al. 2018). While a consensus has not been reached, intraoral scanners may be adjunctive instruments for shade selection.
Additional benefits of digital impressions include improved patient comfort, efficiency, simplified procedures, and better communication with both the laboratory technician and patient (Mangano et al. 2017).
2.3 Limitations of Digital Impressions
Intraoral scanners are not perfect, and they certainly have limitations. It is important that we understand these limitations to know when conventional/analog impression techniques should be performed. Intraoral scanners, as the name implies, scan the surfaces of teeth. Intraoral scanners use various types of technology to capture and record digital impressions. Regardless of the technology, the user is limited by the ability of the scanner to have direct visual access to the critical areas needing to be captured. Understandably, one limitation is the difficulty of intraoral scanners to record subgingival finish lines. Another limitation is the inherent learning curve associated with a new technique. It has been reported that clinicians with less experience and enthusiasm for technology may find using an intraoral scanner and associated software more difficult (Kim et al. 2016; Lim et al. 2018). A reality with technology is the associated costs; intraoral scanners are no exception. Purchase price and maintenance fees can be costly, and as technology is constantly evolving, a scanner can quickly become outdated. Frequent software updates should be considered a benefit, but occasionally, they are accompanied by layout changes, requiring constant training. Software updates commonly fix known issues and have been shown to affect the accuracy of the scanner (Ender et al. 2016). While all impression techniques, both analog and digital, have limitations, it is important to consider the above factors to minimize their effect on daily use.
2.4 Clinical Considerations
2.4.1 Technology of Intraoral Scanners
Since the introduction of intraoral scanning in the 1980s, there has been constant innovation. The first scanners recorded still images and required intraoral scanning spray. The process has evolved into modern‐day color video‐capturing without the need for scanning spray. These advancements are only possible due to improved scanning technology. This section of the chapter will describe two of the most commonly used scanning technologies currently available on the market, allowing for a better understanding of their strengths and weaknesses. These two primary technologies are active triangulation and confocal microscopy. Other scanning technologies include optical coherence tomography, accordion fringe interferometry, and active wavefront sampling; however, they will not be discussed in further detail at this time (Logozzo et al. 2014)
Active triangulation works by projecting light on tooth surfaces. The light may be white, red, or blue and can include special light pattern projections. Light then bounces off the surfaces of the tooth and is captured by a camera sensor. If the light is located further away from the camera, it is recorded in a different location within the camera’s sensor. This allows the scanner to determine different surface contours. The name triangulation was derived because the camera sensor, light source, and the light contacting the tooth surfaces form a triangle. The length of one side of the triangle, the distance between the camera and the light source are known. The angle between the light source and the angle between the camera are also known. From this point, it becomes a standard trigonometry equation to calculate the exact surface location (França et al. 2005; Richert et al. 2017). All of the surface data is then compiled on a computer and, using surface‐matching algorithms, aligned to stitch multiple smaller images into a larger 3D image. One critical aspect of triangulation is the need for a uniform reflective surface. As different materials create different reflective properties, some triangulation technologies require applying a reflective surface powder (i.e. titanium dioxide) to the tooth surface. However, more recent scanners using triangulation use improved techniques such as stream projection or dual camera optical triangulation, which do not require surface powder application. Examples of intraoral scanners that use this technology include: Cerec BlueCam®, Carestream CS 3600, Medit i500, and Planmeca PlanScan (Logozzo et al. 2014; Kim et al. 2018; Kim et al. 2021).
Confocal microscopy works by projecting light that is focused to a specific focal length. This focus allows the light to hit the surface of an object and reflect back to an image sensor, and an image is captured of the in‐focus surface. The specific distance to the object can be calculated because the specific focal length of the lens is known (Richert et al. 2017). The focal length can be fixed or variable using a motor to allow simultaneous image‐capturing, resulting in a 3D surface. The Trios scanner, for example, varies its focal plane at a specific frequency to allow the scanner head to remain at a constant distance to the object while the scanner can acquire multiple images at different focal lengths creating a 3D surface. This technology does require additional components such as a pattern or color wheel that contributes to a larger scanner head and more moving parts than can be found in some of the other scanning technologies. The addition of moving parts also creates vibrations that could cause scanning errors that have to be eliminated by counterweight movement to offset any vibration, adding to the complexity and increasing the number of components that could need servicing (Fisker et al. 2010). This technology does not require any scanning powder to be applied to the surface of the tooth (Logozzo et al. 2014). Manufacturers will use this technology in different ways to achieve additional benefits. For example, iTero uses a parallel confocal imaging technique that uses a red laser light source, while Trios uses light oscillation patterns that are projected on the object. Examples of intraoral scanners that use this technology include: Align Technology iTero Element and 3 shape Trios 3 (Logozzo et al. 2014; Kim et al. 2018; Amornvit et al. 2021; Kim et al. 2021).
Some systems use a combination of triangulation and confocal microscopy technology to combine the benefits while reducing the limitations. For example, the Primescan by Dentsply/Sirona projects a dynamic pattern using a light source without a moving pattern wheel. The projected light pattern has a variable depth of focus that is varied during the projection or imaging of the object (Tewes & Berner 2020). Simplifying the scanning process without additional moving parts is thought to improve reliability by minimizing future maintenance.
2.4.2 Clinical Scanning Techniques
The resolution of an intraoral scanner is determined by the size of the triangles that make up the surface mesh of a scanned image. As the resolution of the scanner increases, the size of the triangles decreases and therefore the mesh is made up of more triangles. The number of triangles can be directly related to the overall accuracy of what is scanned (Nedelcu et al. 2018) (Figure 2.2).

Figure 2.2 Triangular mesh created by scanner. Note areas of smaller and larger triangles.
Intraoral scanners scan the surfaces of teeth and, based on the unique contours of the teeth, can stitch multiple small images together into a larger image, for example, a complete‐arch scan. The key to success is recording the complex contours of teeth, from the cusp tips to the central fossa. Each tooth is unique like a fingerprint. When scanning, it is important to remember the variation in the complex anatomy of various teeth. For example, molars and premolars have complex occlusal morphology while incisors have fewer complex surfaces. This difference in morphology can affect the image stitching capability of the scanners when transitioning from posterior teeth to anterior teeth. Due to this change in tooth contours, specific scan paths or strategies have been suggested.
When initializing an intraoral scan, it is suggested that the scanning must begin with the occlusal surfaces of the teeth within the desired region. The occlusal surfaces then become the baseline reference from which the buccal and lingual surfaces can then be stitched onto and increase the size of the scanned area. Mueller and colleagues (2016) evaluated three different scan strategies and determined that for the tested scanner, scanning the occlusal−palatal surfaces first followed by the buccal surfaces resulted in clinically acceptable values for the complete‐arch scans with higher precision than other scan strategies. When transitioning from the complex contours of the posterior teeth to the anterior teeth, there is a greater chance of having stitching errors (Figure 2.3a,b). Due to this possibility, different scanning strategies have been suggested such as rotating the scanner from the lingual surfaces to the facial surfaces of the anterior teeth to reduce the chance of stitching errors (Ender et al. 2016) (Figure 2.4).
An additional suggestion when transitioning from scanning the occlusal surfaces to the buccal or lingual surfaces is to only rotate the scanner 45° from the occlusal surface. In this way, the scanner can acquire some previously captured data and some newly captured data and minimize the chance of a stitching error. A stitching error is more commonly found when the scanner is rotated 90° from the occlusal surface because of the reduced overlap of previously and newly scanned data (Figure 2.5).

Figure 2.3 (a) Example of stitching error that occurred when transitioning from posterior to anterior; (b) careful examination of the intraoral scan is required to identify stitching errors. Visualization of errors is improved by turning off the color.

Figure 2.4 Example of anterior stitching error caused by improper scan strategy and difficulty of the scanner to capture the narrow facial−lingual dimension of anterior teeth.
The importance of scanning strategies cannot be overstated, and, once again, the importance of understanding the specific scanner used and the scan strategies suggested by the manufacturer is emphasized. If these suggested scan strategies and principles are not followed, there is a much greater chance of incorporating errors into the scan that may or may not be identified. Some examples of suggested scan strategies are shown below (Figure 2.6).

Figure 2.5 When transitioning from occlusal to buccal or lingual surface, it is suggested to rotate the scanner 45° to ensure adequate overlap of scanned data (green circle). If rotation approaches 90°, reduced overlap (red circle) can lead to stitching errors at this location.

Figure 2.6 (a) Maxillary complete‐arch scan strategy; (b) mandibular complete‐arch scan strategy; (c) maxillary quadrant scan strategy; (d) mandibular quadrant scan strategy.
2.4.3 Scanning Environment
Additional considerations when scanning include preparation of the scanning environment to optimize success. It is suggested that scanning must begin with a surface that is clean and free of debris. As is desired with conventional impressions, the surface can be cleaned using an air/water syringe or mechanically cleaned using cleaning/disinfecting agents. Following cleaning, any liquid on the surface of the teeth should be removed, because saliva or plain water remaining on the surface have been shown to affect accuracy (Chen et al. 2021). Additionally, ambient light has been shown to influence both accuracy and scanning time of intraoral scanners. However, there is currently not a consensus on the ideal ambient light condition as different scanners perform better under varying conditions (Revilla‐Leon et al. 2020a,b,c; Wesemann et al. 2020; Koseoglu et al. 2021). Ambient light appears to play a more significant role in the ability of intraoral scanners to accurately record a tooth shade (Revilla‐Leon et al. 2020a,b,c)
Adequate cheek and tongue retraction improves the quality of the scanned data. Some individuals choose to use cheek retraction or just utilize the size of the scanner to achieve this retraction. It is suggested to try multiple retraction techniques to determine the preferred method for each clinician. Additional factors that affect scanning accuracy include restrictive patient opening and patient movement as they can affect the ability to completely scan a patient. While the ideal scanning environment for all intraoral scanners is unknown, it is best to ensure the environment is clean, dry, and soft tissues are properly retracted.
2.5 Accuracy of Intraoral Scanners Compared with Conventional Impressions
All of the currently available conventional impression materials exhibit a small degree of dimensional change that builds distortion and inaccuracy into the final restoration. Digital impressions eliminate the possibility of dimensional change that is inherent in all conventional impression materials. Voids, tears, and pulls that are routinely experienced with elastomeric materials are no longer an issue with digital scans. In addition, the use of dental stones to create physical dental casts introduces additional inaccuracies due to expansion of stone and the possible movement or shifting of the individual dies when sectioned from the working cast. With digital scans, the number of steps is reduced and therefore the number of possible inaccuracies are reduced. Scans can be evaluated immediately for accuracy and restorations designed from the digital scans, avoiding the inaccuracies mentioned above.
Research on the accuracy of digital impressions focuses on the trueness and precision of a scan. These two elements together determine the accuracy of a digital impression. Trueness is the closeness between what is scanned to the exact value of that surface. In other words, how close is the average value of the results to the exact measured value. Precision is the reproducibility of getting that same result each time you scan. The combination of both trueness and precision determines the overall accuracy of what is scanned (Figure 2.7). Renne et al. (2017) evaluated the accuracy of seven different digital scanners in an in vitro analysis. They compared both laboratory and intraoral scanners and determined that scanners differ regarding speed, trueness, and precision. Additionally, they reported that some scanners performed better with complete‐arch scans while others performed best when scanning quadrants. Overall, there are many studies that support the claim that intraoral scanners have accuracy comparable to conventional impressions (Ender et al. 2016a,b; Syrek et al. 2010; Nedelcu & Persson 2014).

Figure 2.7 Intraoral scanners require trueness and precision for overall accuracy.
2.6 Accuracy of Complete Arch vs. Quadrant Scans
The decision to scan only a quadrant or extend scanning to include the complete arch is an important consideration. As more surface data is captured, more potential stitching errors could be incorporated (Gan et al. 2016). The concept of “less is more” holds true with digital scanners as quadrant scans have been shown to be more accurate than complete‐arch scans (Park et al. 2019; Ender et al. 2019; Moon & Lee 2020
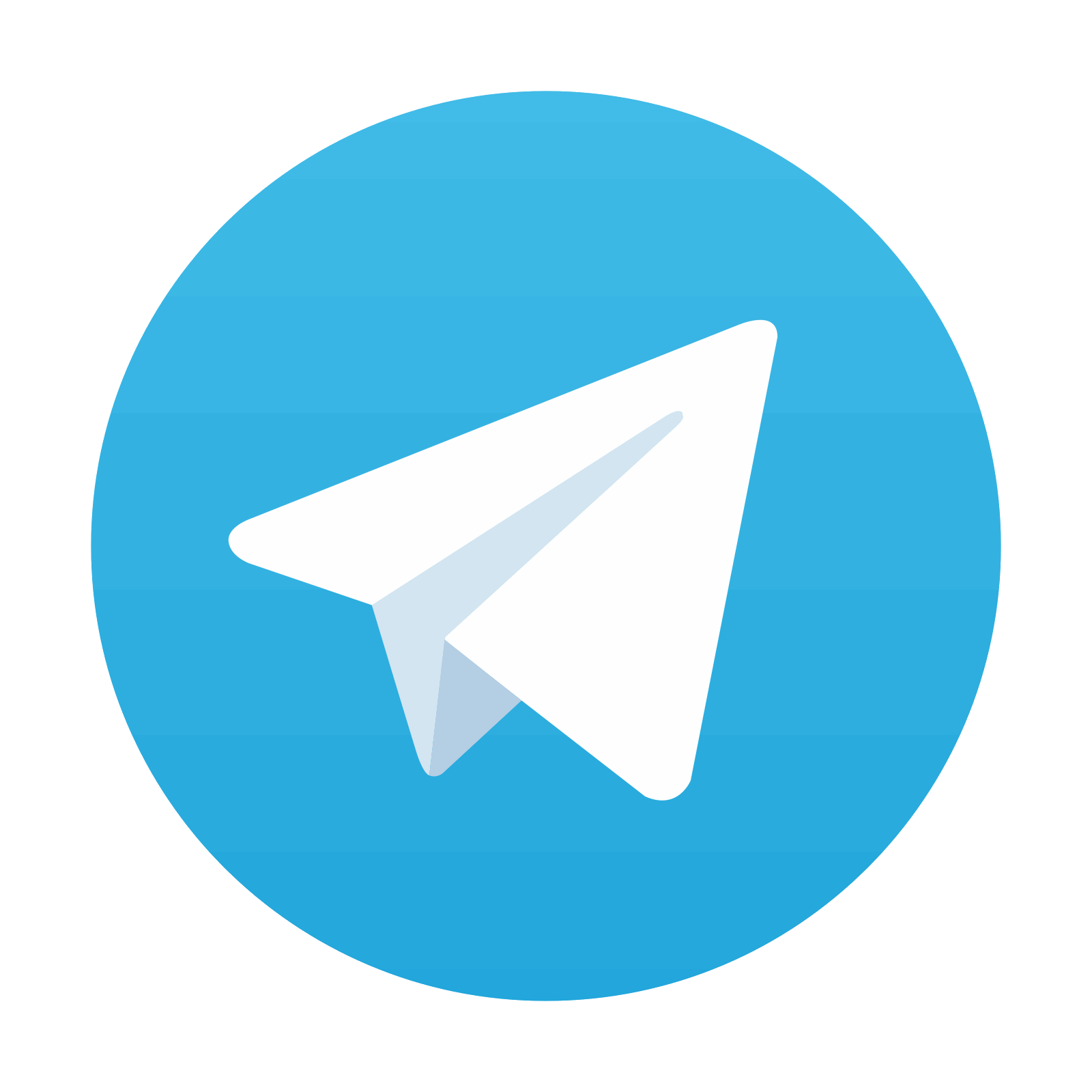
Stay updated, free dental videos. Join our Telegram channel

VIDEdental - Online dental courses
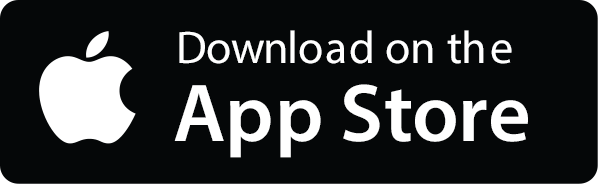
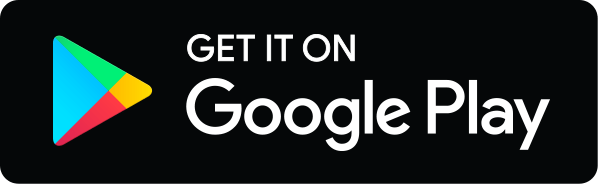