13
Clinical Applications of Digital Dental Technology in Orthodontics
Jose A. Bosio
13.1 Introduction
Dental tooth movement has evolved from “gold bands and wires to plastic,” and about 120 years later, the profession of orthodontics has become intimately intertwined and dependable on digital technology. This chapter will present the interdependence of digital technology tools in all aspects of orthodontic diagnosis and treatment planning and physical digitally created tools used as auxiliary to the orthodontic dental movement. Furthermore, this chapter presents the interdependence of orthodontics and digital technology to conduct business in the twenty‐first century.
In the past, physical paper charts and folders were used to hold patient personal health information (PHI). In contrast, the ability and speed with which information can be searched and retrieved currently and the knowledge gained by the computer evolution has propelled to newer heights, not only orthodontics, but in all health care professions. Technology has allowed orthodontic private practices to grow their business and to see more new patients as a consequence of the time saved. Increase in patient volume associated with improved technology to deliver orthodontic care led to an exponential increase in time required for computer screen dedication. Thus, orthodontic professionals’ debate if the tradeoff is worth it. Nonetheless, it is assumed that the greater majority of orthodontic professionals currently prefers newer technology trends to the difficulty of practicing orthodontics in the early‐ and mid‐1900s.
13.2 History
The orthodontic profession was established in the late 1800s when Edward Hartley Angle published his first classification of malocclusion and decided to dedicate the rest of his dental career to the new rising specialty of orthodontics (Peck 2009a–c). Since then, monumental changes have occurred in the specialty, starting from utilization of gold bands and wires, creation of the edgewise appliance, incorporation of cephalometrics, utilization for extraoral and intraoral removable and fixed appliances, such as headgears, activators, rapid palatal expanders, and temporary anchorage devices (TADs), invention of straight wire appliances, innovations in wire alloy metallurgy, creation of plastic and ceramic brackets, establishment of clear aligners as new modality of orthodontic dental treatment last, but not least, digital printing individual prescription braces.
The internet advent (World Wide Web – WWW) created by Tim Berners‐Lee in 1992 helped to accelerate these changes once the educational world expanded and became intertwined. New online platform designs spiked the ability to instantly reproduce images created thousands of miles away. As interactions and technologies changed everywhere, the dental industry was no exception. The catalyst for change in this industry has been the digital platforms developed across the globe (van Noort 2012).
The graphic below helps to briefly describe how the orthodontic profession evolved, and how the digital technology came to affect how orthodontics is practiced nowadays (Figure 13.1).

Figure 13.1 Chronological history of orthodontics and digital orthodontics.
13.2.1 Diagnosis and Treatment Plan
It is common knowledge that documenting changes to facial and dental characteristics is the hallmark of orthodontics. Thus, one of the greatest impact digital technology created in orthodontics is in the diagnosis and treatment plan phases. Since its inception, Dr. Edward H. Angle recognized that ideally documenting cases during the initial phases of treatment not only would help in finding all possible clinical solutions, but would also indicate visual changes to orthodontic cases, which, in turn, would support initial treatment decisions, or teach lessons to prevent perpetuation of errors on clinical results (Angle 1903). Copy of the teeth had its own evolution, from dental impression with vulcanite to digital acquisition with intraoral scanners. Imaging of internal structures also evolved from two‐dimensional (2D) radiographic films to three‐dimensional (3D) cone beam computer tomography (CBCT) imaging of all structures in the head and neck area. Photographic imaging of the face also evolved from 2D extraoral pictures to 3D scanning of all facial structures, capturing details of facial structures never seen before.
However, the higher the quality, the more technological resources became necessary to store these digital files. The quest for the best, the greatest, or a unique diagnostic tool will likely never stop, since companies and inventors will continue producing new “gizmos” to visualize structures, and concomitantly push to sell to eager buyers. On the other hand, professionals must make decisions on what they believe will improve the quality of orthodontic treatments, where to invest their assets, and what technologies to acquire for their practice. The contrast between the old and the new will continue pushing technology to advance, and the market will dictate what is worth developing and what is not. However, once a diagnostic tool is purchased, it must be used to pay for itself, which in essence, the buyers will likely find a way to use to produce images, or to verify certain characteristic measured by the diagnostic tool purchased, despite the support from scientific evidences or the possible need for such exams.
13.3 Imaging
Among many tools used for orthodontic diagnosis, imaging is the one with the ability to depict internal or external structures. For matter of description, the imaging section was divided into “penetrating images,” which in most cases are X‐rays, but can also be ionizing energy, and “surface imaging” (intra or extraoral surfaces).
13.3.1 Penetrating Imaging
13.3.1.1 Intraoral Radiographic Films
Intraoral radiographic films were first taken of a living person in the United States in 1896 by C. Edmund Kells; however, dentists began to use X‐rays regularly in the 1950s (Riaud 2014). Despite the advancements in dental radiology, it was not until the late 1990s that dentists started to utilize digital dental equipment. Digital radiograph is the X‐ray imaging that uses digital X‐ray sensors instead of the old traditional photographic films. The method using photographic films required them to be processed in a dark room or box, and usually required more time for processing. The benefits gained by collecting digital images were the reduction of ionizing radiation delivery, the ability of visualizing films immediately after acquiring, retaking if wrong acquiring techniques were applied, minimal digital micro‐storage technology allowing saving on small space‐saving drives, and immediate retrieval when necessary to visualize in office or to transfer to other professionals in other locations (Bosio et al. 2017a,b, Dentistry 2021). Currently, the standard of care of visualization of specific dental structure problem is the intraoral radiographic films.
The most important types of intraoral X‐rays for orthodontics are: (i) Periapical X‐ray – which shows the entire tooth or group of teeth, and the surrounding structures, and are best used to determine bone loss, periodontal disease, and endodontic lesions such as abscess and cysts (Figure 13.2a,b); (ii) Bitewing X‐ray – which is an taken with the patient biting down and it shows the dental crown up to the level of the supporting bone. This imaging technique is a good diagnostic tool to show interdental decay and marginal integrity of tooth restorations (Figure 13.3). To diagnoses dental disease, dentists need to have high‐quality images and the best tool provided by digital technology is certainly the ability to zoom into the images where better details can be visualized on a computer screen (Figure 13.3).

Figure 13.2 (a) Digital maxillary periapical X‐ray. (b) Digital mandibular periapical X‐ray.

Figure 13.3 Digital maxillary and mandibular bitewing X‐ray.

Figure 13.4 Digital panoramic X‐ray.
13.3.1.2 Panoramic X‐ray
Analogical panoramic radiographic film, also known as pantomography, was created during the early 1960s (Hallikainen 1996). The panoramic radiograph tool is deemed to have greater value as a screening tool rather than for diagnostic purposes (Quintero et al. 1999). Its shortcomings as a diagnostic tool relate to the reliability and accuracy of size, location, and form of the image created, especially because the image is created by a focal trough or region of focus on a generic jaw form and size (Quintero et al. 1999). The image also presents significant amount of distortion and lacks fine anatomic detail and the presence of overlapping anatomical structures. Nonetheless, the ability of working as a primary source for clinical situations, such as, but not limited to, visualization of both maxillary and mandibular jaws on the same X‐ray, presence of asymmetric condyles, midpalatal suture (MPS) (Kanas 2018), dental eruption pattern, dental impactions, maxillary sinus lesions (Bosio et al. 2009), supernumerary teeth, periodontal health, and dental caries has depicted its use as the standard of care in orthodontics (Figure 13.4).
The controversies in orthodontics around panoramic X‐rays is significant. Although every professional understands the limitations around the panoramic X‐ray, its distortion and limited diagnostic value, everyone also recognizes the ability to visualize conditions not seen clinically. Despite the flaws, it is intriguing to understand why the panoramic X‐ray has become the minimum standard diagnostic tool for the orthodontic profession according to the American Board of Orthodontics. However, the resistance to accept attempts to establish standardized diagnostic features from this source in lieu of more current CBCT imaging sources, prevents the overall public from benefiting from broader knowledge.
For example, a master’s thesis study conducted by Kanas in 2018 aimed to validate a system for individual assessment of MPS maturation based on panoramic radiographic films, and to test the reliability of correctly predicting the right grading system over time (Kanas 2018). Using 173 panoramic X‐rays, the authors extracted images from the MPS and maxillary central incisors to create four stages of the MPS maturation system (Figure 13.5). The description of the stages can be seen in Table 13.1. Intra‐examiner and inter‐examiner reliability consistently increased over time as examiners practiced the method, as expected. This study was possible only because the panoramic images could be zoomed in digitally. Thus, along with other orthodontic studies in which maxillary canines’ inclinations, third molar impactions, periodontal defects, and other features seen in panoramic digital films were studied, digital panoramic images enable professionals to conduct better orthodontic diagnosis and treatment plans.
As a primary tool for screening, the digital panoramic radiograph enhances the possibility of zooming actions into specific areas of the film for better visualization. Once the preliminary observation is conducted, referral for more specific exams, such as periapical X‐ray films or CBCT can be made.
13.3.1.3 Lateral Cephalometric Radiographic Films
In 1931, Hofrath in Germany and Broadbent in the United States introduced cephalometric radiography to orthodontics. The new element of diagnosis provided better understanding of clinical orthodontics regarding dental and skeletal normal and abnormal positions. It also delivered elements for clinical research in malocclusion and underlying skeletal proportions, and its findings could be followed long term to determine different growth patterns and trends. For the next 30 years, orthodontics involving cephalometric analysis would expand and many authors would create their own analyses, all claiming to have created the standard by which every orthodontic patient should be treated (Jacobson 1975, McNamara 1984, Steiner 1953, Tweed 1954).

Figure 13.5 Midpalatal skeletal maturation system stages.
Table 13.1 Skeletal maturation stages of the midpalatal suture description.
Stage | Description |
---|---|
1 | Straight with parallel high‐density lines and little or no interdigitation; appears as a complete radiolucent line from the floor of the nasal cavity inferiorly completely through the crestal bone between the maxillary central incisors. |
2 | Interdigitated less than half the distance from the floor of the nasal cavity inferiorly to the crestal bone between the maxillary central incisors but not completely radiolucent as in stage 1. |
3 | Interdigitated more than half the distance from the floor of the nasal cavity inferiorly to the crestal bone between the maxillary central incisors but not fully fused as in stage 4. |
4 | Fully interdigitated from the nasal floor inferiorly down the crestal bone between the maxillary central incisors. |
The lateral cephalometric X‐ray diagnostic tool provides professionals with the ability to diagnose the position of the maxillary and mandibular jaw bones in relation to the cranial base by indicating their spatial position, either one or both jaws at fault, either too far forward or backward, and their relation to each other. Each jaw position combination can indicate if orthodontic treatment may be provided only by dental movement, or by alteration of the structural skeleton by means of orthognathic surgery. The lateral cephalometric X‐ray also shows the position of the dental structures, mostly position and inclination of incisors and position of molars, in relation to the jaws they are located on and in relation to each other (Figure 13.6a).

Figure 13.6 (a) Digital lateral cephalometric X‐ray. (b) Digital lateral cephalometric X‐ray and the dental and skeletal structures’ tracing. (c) Digital lateral cephalometric tracing superimpositions to determine the treatment effects.
Between 1984 and 1985, authors started to investigate the possibility of using digital cephalometric measurements (Cohen et al. 1984, Konchak and Koehler 1985). Konchac and Koehler wrote the first software using a digital pad to record landmarks and produce meaningful analysis by means of computer‐assisted programs. Their software used vectors and vector algebra and the capabilities of the Pascal computer language to determine angular measurements and distances. Their study was the first to claim that digital cephalometric X‐rays produced a superior method of cephalometric analysis with respect to accuracy and speed of completion (Konchak and Koehler 1985).
The ability of acquiring a digital image in contrast to the old processed films would lay not in the ability to instantly verify the film quality, but more on the reduction of X‐ray dose to the patients (Gijbels et al. 2004, Visser et al. 2001), possibility of instant magnification of image visualization, changes in contrast for depicting hard and soft tissue elements, reliability of landmarks location (Konchak and Koehler 1985, Macrì and Wenzel 1993), easiness of changes on soft or hard landmarks after being detected, creation of hundreds of different cephalometric analyses, verification of treatment changes given by tracing superimpositions, and treatment plan: surgical and non‐surgical predictions.
Besides the old and reliable hand tracing of lateral cephalometric X‐rays, the orthodontic specialty has the ability to choose from many different cephalometric software to manage cephalometric tracings, each one with their unique features and characteristics (Figure 13.6b). Furthermore, the digital technology allows for orthodontic professionals to superimpose cephalometric tracings such as initial (black), intermediate (blue), final (red), and retention (green) in a significant number of cranial structures to observe effects of growth and/or treatment (Figure 13.6c).
In the United States, in 2021, the available software capable of tracing and analyzing lateral cephalometric X‐rays are (not in order of preference or importance): Planmeca Romexis®, Dolphin Imaging®, Quick Ceph Systems, CephX®, OrisCeph Rx CE®, topsCephMate™, CephNinja®, PRO‐CEPH V3®, Joe‐ceph®, Facad®, Viewbox 4®, and others, not listed here. Furthermore, the orthodontic profession can now utilize online services such as “easyceph” and others to accomplish this important diagnostic evaluation.
13.3.1.4 Cone Beam Computed Tomography
Cone beam computed tomography was introduced to dentistry in 1998 (Mozzo et al. 1998) and approved for use in the United States in 2001 (Kapila and Nervina 2015) Since then, orthodontics has adopted the technology to assist in the specialty diagnosis and treatment plan. The pure capability of visualizing 3D images over a plane film, increased the knowledge of deep structures inside the cranium, and accelerated the knowledge of anatomical landmarks and pathologies unseen on regular films. Skeptical clinical orthodontists postponed their beliefs that CBCT could provide more relevant clinical information than the long‐used panoramic and lateral cephalometric radiographs. This technology has gained market share since CBCT science has been able to demonstrate its advantages over plane films, especially in the areas of location of dental impactions and supernumerary teeth, root resorptions, airway studies, TADs, orthognathic surgery and craniofacial disorders, transverse discrepancies, and other orthodontic characteristics as well as matching digital intraoral scans with CBCT technology.
Cone beam computed tomography uses a cone‐shaped tube instead of a collimated fan beam with spiral CT scanners. The resulting cone‐shaped X‐ray beam performs a 360° rotation, synchronously with an area detector, around the object being scanned while its angulation remains constant throughout the entire scanning period. This movement allows an entire region, such as the craniofacial region, to be captured in a single rotation of the radiation source instead of “multiple slices” that are being generated by conventional CT units (Mozzo et al. 1998) (Figure 13.7a,b). Also, the CBCT X‐ray beams are almost parallel and closer to the sensor, thereby, providing an orthogonal beam projection that minimizes image distortion and improving its quality (Haney et al. 2010). These images contain isotropic voxels (volume elements) such that each volume element has equal dimensions in all three orthogonal planes, allowing accurate multi‐planar images in any direction desired by the practitioner (Abdelkarim 2019) (Figure 13.7c,d).
Although CBCT increased the capability of producing more accurate diagnosis, many orthodontists still question its validity to improve orthodontic treatment quality. The greatest concerns still lay on the amount of radiation produced by this diagnostic test. Ionizing radiation is carcinogenic and has the potential to cause other deleterious effects, despite the amount delivered (Abdelkarim 2019). If a professional considers the number of child patients in comparison with adults, one will find that most orthodontic professionals treat more children than adults and if children are more susceptible to radiation than adults, then professionals should consider limiting unnecessary exposure to radiation.
13.4 Cone Beam Computed Tomography Dosage
Cone beam computed tomography effective doses are smaller than those of a medical CT. However, CBCT machines produce a wide range of effective doses and they are strongly correlated with the size of the field of view (FOV). Increasing the FOV increases proportionally the amount of radiation (Ludlow et al. 2006). Therefore, reducing the size of the FOV is one of the best ways to reduce the effective dose of CBCT. However, most adjustments to reduce radiation might affect the quality of the images. In all attempts to reduce radiation dosage, CBCT will usually generate more radiation than lateral cephalometric and panoramic films together (Abdelkarim 2019). An effective dose of a digital panoramic radiograph has the range of 6–38 microSieverts (μSv) (Ludlow et al. 2006) and the effective dose of a cephalometric radiograph has the range of 2–10 μSv (Silva et al. 2008). CBCT effective dose may vary from 5.3 to 1025 μSv, depending on the size of the FOV, specific technique factors, and the machine itself (Ludlow et al. 2008) (Table 13.2).
The use of a CBCT should be considered only when these scenarios are anticipated to: (i) determine the magnitude of a craniofacial deformity, (ii) improve diagnosis, such as specifically localizing impacted and supernumerary teeth; (iii) improve capabilities to establish appropriate surgical guides for surgical procedures; and (iv) verify TMJ anomalies that could cause asymmetry or open bites, such as joint degenerations. The CBCT exam should only be taken if the treatment to be rendered could potentially be altered, otherwise a plain 2D film could be the best option for imaging. However, a plane‐lateral cephalometric X‐ray can be obtained following CBCT and can be measured quite accurately (Ludlow et al. 2009, Moshiri et al. 2007). Furthermore, a CBCT exam should be taken if an oral surgeon finds it difficult to determine the best buccal or lingual access to remove an impacted tooth, to determine whether a tooth has crossed a midline, to determine the thickness of enamel or root canal, the amount of root resorptions of adjacent teeth, the airway volume, or to measure physiologic changes due to craniofacial growth by superimposing on the anterior cranial base (Bosio et al. 2011, Cevidanes et al. 2006, Chang et al. 2013, Ludlow et al. 2009).

Figure 13.7 (a) Digital cone beam computed tomography (CBCT) occlusal view with contrast. (b) Digital cone beam computed tomography (CBCT) lateral view with contrast. (c) Digital cone beam computed tomography (CBCT) axial view. (d) Digital cone beam computed tomography (CBCT) sagittal view.
Table 13.2 Dosage comparison between all types of X‐rays.
Imaging technique | Approximate range of effective dose (μSv) |
---|---|
Medical head computed tomography | 1000–2000 |
Cone beam computed tomography | 5.3–1025 |
Panoramic radiography | 2–10 |
Cephalometric radiography | 6–38 |
Despite controversies on the use of CBCT technology as standard of care, the American Association of Orthodontists issued a resolution in 2010 stating that “while there may be clinical situations where a CBCT radiograph may be of value, the use of such technology is not routinely required for orthodontic radiography.” Furthermore, studies have demonstrated that a CBCT image taken at the initiation of orthodontic treatment does not significantly impact treatment decisions (Kapila et al. 2011) and, knowing exactly where impacted canines are located or the position of condyles inside of the mandibular fossa does not significantly impact the choice of treatment plan or even the mechanics used to execute the proposed mechanics (Halazonetis 2012, Rinchuse and Kandasamy 2012). However, CBCT images can significantly impact the visualization of each orthodontic case, and they can provide detail in all planes of spaces as seen in the figures above.
- CBCT machines
The major CBCT machines in the United States market in 2021 are, in no particular order: Planmeca (Promax, Viso), Carestream Dental (CS8200, 9600 3D), i‐CAT, Kavo OP 3D, Dentsply Sirona (Axeos, Orthophos SL/S 3D, Galileos), PreXion 3D (Explorer/Excelsior), among other smaller manufacturers. Each machine has its own characteristics and readers should investigate online for specific characteristics they intend to observe in each unit.
- Magnetic resonance imaging
Magnetic resonance imaging (MRI) can produce cross‐sectional images of the human body in any plan without exposing the patient to hazardous ionizing radiation. These images are produced by the interaction of the body’s hydrogen nuclei, high magnetic energy fields, and radio frequency pulses.
Felix Bloch at Stanford University and Edward Purcell at Harvard University proved that certain nuclei behave like miniature magnets. They possessed north and south poles when placed in a magnetic field (Bloch et al. 1946, Purcell et al. 1946). They showed that the magnetic moments could be torqued by an externally applied radio frequency pulse that pushed the magnetic field moments to a higher level. When the externally applied radio frequency pulse was stopped, the magnetic moments returned to their original position and energy state, and emitted the “lost” energy as a MR signal characteristic of that proton. They received the 1952 Nobel Prize for this discovery.
Three major components make part of the MRI facility: (i) the gantry, which houses the patient in a bore of a huge magnet containing the radio frequency coils; (ii) the operating console, where scanning procedures are controlled; and (iii) the computer system, which provides data acquisition, processing and storage, and display functions. The raw MR data signals from the patient are computers encoded into an image through an electronic network connecting the three components (Tasaki and Westesson 1993).
Currently, MRI technology has been used more to diagnose temporomandibular joint disorders (TMD) than any other major application for diagnosis and treatment plan in orthodontics (Figure 13.8a,b,c,d). Furthermore, some reports have shown that metallic braces do not interfere with some types of MRI exams (Miao et al. 2020), indicating that metallic braces do not need to be removed while conducting these exams for orthodontic purposes or otherwise.
13.4.1 Surface Imaging
13.4.1.1 Digital Photography
In 2002, an article published by Palomo et al., described the experience to switch the department of orthodontics at Case Western Reserve, in Cleveland, OH from a film base to digital photography (Palomo et al. 2004). The authors defined the advantages of digital photography over film systems, including rapid image production, easy and quick deletion of imperfect images, no need for film and its associated expenses, decreased cost for enlargement, ease of editing and image storage, improved communication, and effortless placement in presentations or publications (Palomo et al. 2004). Thus, digital photos became suited for immediate data transmission, for instance, to a lab, to a colleague, or even to the patients themselves (Bengel 2000). Since that time, digital photography has become the standard for the specialty and for dentistry in general.

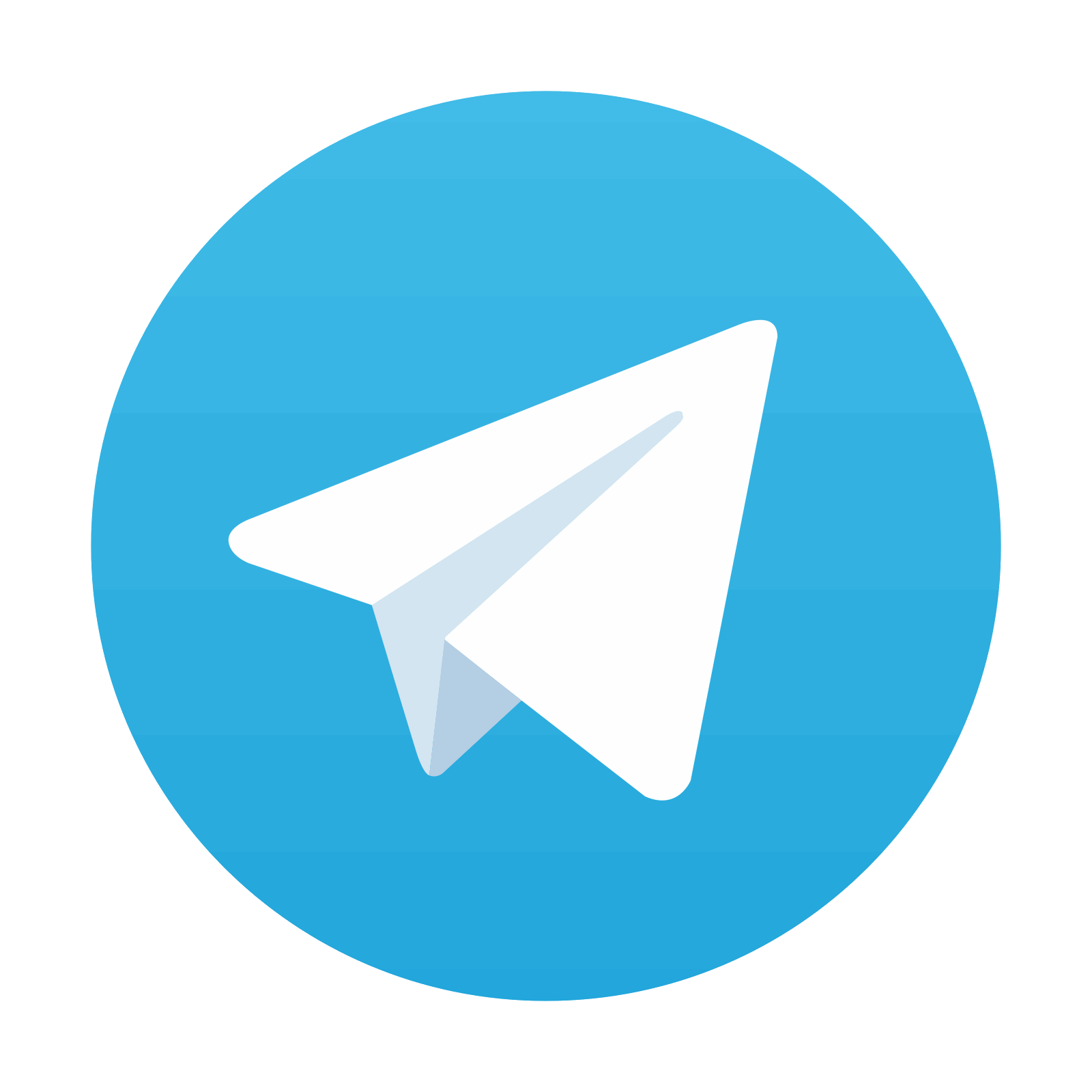
Stay updated, free dental videos. Join our Telegram channel

VIDEdental - Online dental courses
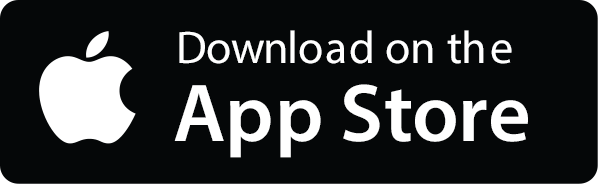
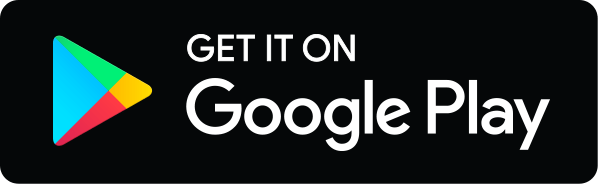