11
Virtual Articulators
Wei-Shao Lin, Chao-Chieh Yang, and Dean Morton
11.1 Traditional Mechanical Articulator
For years, dentists have tried to design an instrument that can accurately reproduce human mandibular movement. Articulators have been developed to simulate an individual patient’s mandibular movement, allowing dentists to evaluate occlusion, develop a treatment plan, and fabricate dental prostheses with appropriate occlusal design. Since Pfaff first articulated casts, and Jean Batiste developed a plaster articulator (Starcke 1999), many types of articulators have been developed (Becker and Kaiser 1993). There are three major components in an articulator design including (i) hinge axis, (ii) reference plane, and (iii) mechanical condyle. During the course of history, various theories were developed to locate the hinge axis and simulate condyle movement (Weinberg 1963). Based on different concepts, articulators can be divided into three categories: (i) nonadjustable, (ii) semi‐adjustable, and (iii) fully adjustable (Rihani 1980).
Initially, articulators were designed geometrically based on occlusion theory and provided limited or null adjustability. With limited adjustability, nonadjustable articulators do not provide adequate accuracy for complex indications (Hobo et al. 1976). Therefore, to accurately duplicate a patient’s mandibular movement, fully adjustable articulators that can accept and reproduce measurements recorded by a pantograph were developed (Guichet 1979). However, due to the complexity of the procedures and sophisticated articulator programming, such articulators have not been generally accepted in daily practice by dentists (Schweitzer 1969) (Figure 11.1a,b).
Semi‐adjustable articulators are perhaps the most commonly used articulators by dentists and prosthodontists (Figure 11.2). Multiple methods have been proposed to adjust various semi‐adjustable articulators. Christensen introduced a method to adjust sagittal condylar inclination (SCI) by using protrusive interocclusal records (Christensen 1905). Mechanical and electronic pantographs that use mandible tracings to record sagittal and horizontal condylar inclination (HCI) have been advocated (Celar and Tamaki 2002, Wagner et al. 2003). It has been reported that protrusive records will register a more shallow and less consistent HCI than pantographic tracings (Torabi et al. 2014). However, pantographic instruments are relatively expensive, and errors are more likely dependent on clinician experience. As a result, protrusive interocclusal records are more routinely taught in dental education and recommended in dental textbooks (Shillingburg et al. 1997) (Figure 11.3a,b).
A facebow is an instrument that can transfer a patient’s mandibular hinge axis to an articulator and record how the patient’s occlusal plane relates to the horizontal reference plane. In general, location of the hinge axis can be managed by two types of facebow, the kinematic and the arbitrary. Studies have demonstrated both facebow options can produce clinically acceptable prostheses when the number of restorative units is limited, and the vertical dimension of occlusion is not altered (Kois et al. 2013). An arbitrary articulation system that positions the maxillary cast by recording an esthetic reference position has also been proposed and accepted by general dentists and specialists (Lux et al. 2015).
Current and future dental practice will rely heavily on emerging and developing technologies that allow clinicians and technicians to compose a virtual patient and simulate function and esthetics. Although mechanical articulators will still play an important role in most practices and laboratories, virtual articulators will likely offer a wider range of possibilities and alternatives to improve clinical treatment outcomes. This chapter will focus on the introduction of the virtual articulator and its clinical applications.

Figure 11.1 (a) Fully adjustable articulator can be adjusted to duplicate a patient’s condylar path of movement as recorded by a pantograph tracing. A Denar D5A is an example of a fully adjustable articulator. The following adjustments can be made on Denar D5A, including protrusive angle 0–60°, immediate sideshift 0–4 mm, progressive sideshift 0–30°, rear wall 30° backward, top wall 30° up, 30° down, intercondylar distance 90–150 mm. Different views in this figure show various components that can be adjusted on wa Denar D5A articulator. (b) Articulator programming for a fully adjustable articulator could be accompanied by the electronic pantograph system (Cadiax Compact 2; GAMMA Medizinisch‐wissenschaftliche Fortbildungs‐GmbH) to record and reproduce the mechanical equivalent of mandibular movements.
11.2 Virtual Articulator
11.2.1 Need for Virtual Articulators
Computer‐aided design and computer‐aided manufacturing (CAD–CAM) is part of what is commonly described as digital dentistry and has become increasingly popular and important in dental practice. Virtual articulators have been and continue to be developed, using digitized datasets in a virtual environment to reproduce accurate occlusal relationships and functional jaw movements (Lepidi et al. 2021). The goal of virtual articulators is to overcome the inherent shortcomings of mechanical articulators and physical dental casts, which have been associated with a limited ability to simulate the variability of a patient’s biological articulation system. For example, mechanical articulators are not able to simulate the deformation of the human mandible under loading and different masticatory movements. Mechanical articulators are limited and do not allow for an accurate representation of complex masticatory cycles and phases. The influence of soft tissue resilience and time‐dependent mandibular movement is not taken into account when using mechanical articulators (Kordaß et al. 2002).

Figure 11.2 Semi‐adjustable articulator (Model 2240 Articulator; Whipmix Corp) can simulate condylar pathways by using average values or mechanical equivalents for part of the mandibular motions.

Figure 11.3 (a) A protrusive record can be made intraorally with record bases and registration material to program sagittal condylar inclination (SCI) on a semi‐adjustable articulator. (b) Based on the protrusive record, SCI was set as 35°.
Accurate articulation of dental casts is also a challenge, influenced by the possible deformation of occlusal registration material, distortion of dental casts, improper selection of occlusal recording technique, limited clinician capability, and operator errors (Zarbah 2018). The reliability of a mechanical articulator to reproduce intraoral protrusive and laterotrusive contacts is limited due to these shortcomings (Celar et al. 1999, Tamaki et al. 1997). Celar et al. showed that when a semi‐adjustable mechanical articulator (SAM2 P articulator; SAM Präzisionstechnik GmbH) and kinematic facebow (SAM Präzision‐stechnik GmbH, Munich, Germany) were used to articulate diagnostic casts, and when the articulator’s HCI and Bennett angles were adjusted according to hinge axis movements recorded with computerized axiography (Cadiax, version 4.12, Gamma GmbH, Klosterneuburg, Austria), mechanical articulators duplicate only 73% of intraoral protrusive and 81% of intraoral laterotrusive contacts (Celar et al. 1999). Virtual articulators have been developed to overcome these challenges associated with mechanical articulators.
11.2.2 History of Virtual Articulators
In 1999, a fully adjustable three‐dimensional (3D) virtual dental articulator based on a mathematical simulation of the articulator movements was introduced (Szentpétery 1999). This virtual articulator is capable of simulating movements not offered by some mechanical articulators, such as the curved Bennett movement. However, since it utilizes a mathematical simulation, Szentpétery’s virtual articulator has been reported to behave similarly to an average value articulator (Padmaja et al. 2015).
In 2002, Kordass and Gartner introduced a virtual articulator system, aimed at incorporating patient‐specific data into a software program (DentCam, Kavo; Hamburg, Germany) to generate simulated jaw movements and produce a dynamic and individualized visualization of occlusal contacts (Lepidi et al. 2021, Padmaja et al. 2015). A jaw motion analyzer (Zebris Company; Isny, Germany) is used to record patient‐specific mandibular movements by measuring the velocity of ultrasonic impulses emitted from transmitters and receivers. A digital sensor allows the determination of a reference plane (for example, the infraorbital or occlusal plane). Articulated virtual dental casts are then registered with recorded patient‐specific mandibular movements in the virtual articulator system (DentCam) (Gartner and Kordass 2003, Kordaß et al. 2002). By using recorded jaw motion, the virtual articulator provides a bridge to connect and complete digital data acquisition and CAD. Dental clinicians and technicians can have dynamic visualization of the occlusal contacts and optimize the occlusion on the CAD–CAM prostheses accordingly. The DentCam system may be considered a completely adjustable (CA) virtual articulator because it uses an electronic jaw registration system to accurately record movements of the mandible. Although it can accurately assess patient‐specific mandibular movements, it is less user‐friendly and expensive. It is more suitable for complex dental rehabilitations where the occlusal morphology of prostheses needs to be carefully designed to avoid occlusal interferences in excursions (Koralakunte and Aljanakh 2014, Lepidi et al. 2021).

Figure 11.4 (a) A mathematically simulated virtual articulator is similar to a mechanical articulator and can be adjusted with various predefined parameters to simulate desired mandibular movements. Intraoral scans can be positioned on a virtual articulator with virtual face bow transfer and interocclusal records. Programming of virtual articulators can be achieved by using average values or readings from the electronic pantograph system. (b) Virtual articulators can simulate limited mandibular movement and dynamic occlusal contacts based on predefined parameters.
In contrast to the CA virtual articulator, a mathematically simulated (MS) virtual articulator is an average value articulator and does not record or reproduce the individualized mandibular movement of each patient (Figure 11.4a). Similar to a mechanical articulator, a MS virtual articulator can be adjusted with various predefined parameters, such as Bennett angle or condylar inclination, to simulate desired mandibular movements. Although MS virtual articulators are not capable of reproducing patient‐specific mandibular movements, they may be indicated for clinical treatments where the occlusal morphology of prostheses can be managed to exclude occlusal disharmony. Although simulated dynamic occlusal contacts can be visualized in a MS virtual articulator, they may not accurately reflect occlusal contacts in excursions that occur in the patient (Figure 11.4b). Irrespective of these shortcomings, MS virtual articulators are more user‐friendly and less expensive than CA virtual articulators, and so are more widely accepted (Koralakunte and Aljanakh 2014, Lepidi et al. 2021).
Szentpétery’s virtual articulator can be considered as an early attempt to produce an MS virtual articulator. In 2009, 3D scanner and reverse engineering software programs were used to develop a new generation of MS virtual articulators. The new design introduced inter‐condylar distance as an adjustable component and provided a basis for digitalizing commercially available mechanical articulators. Dental clinicians and technicians can therefore pick and choose what they consider to be the most suitable MS virtual articulator when designing CAD/CAM prostheses (Koralakunte and Aljanakh 2014, Padmaja et al. 2015, Solaberrieta, Garmendia, et al. 2010) (Figure 11.5).

Figure 11.5 The application of facial scan, intraoral scan, and virtual articulator have opened a door for more accurate and predictable diagnosis, treatment planning, and CAD/CAM prostheses fabrication.
11.3 Virtual Articulation
11.3.1 Brief Overview of Clinical Procedures
Direct and indirect workflow options can be used to transfer digital data and position digital casts for MS virtual articulators (Úry et al. 2020). For the indirect workflow option, conventional analog impressions are made and the resulting stone dental casts are articulated using a facebow and interocclusal records on a mechanical articulator. A laboratory scanner is used to digitize the stone casts and articulation and transfer the information onto a virtual articulator (Úry et al. 2020). For the direct workflow option, intraoral scanners are used to obtain a digital representation of dental arches and interocclusal records. Instead of traditional facebow, various digital facebow techniques have been proposed to register the maxillary digital cast using a 3D facial scan and a static 3D dental patient (Amezua et al. 2021, Solaberrieta et al. 2015).
The static 3D dental patient can be registered with additional facial scans recording different facial expressions or with 3D digital volumes (craniofacial hard tissues) from cone‐beam computed tomography (CBCT) to satisfy different treatment demands (Amezua et al. 2021). Once the 3D dental patient is complete, a reference plane (such as Frankfort plane, Camper’s plane, or axis‐orbital plane) can be identified from the facial scan to register a virtual articulator to the 3D dental patient (Lam et al. 2018). In summary, although the specific nature of clinical steps may vary, the following three clinical steps are required to utilize a virtual articulator and a digital workflow: (i) digital data acquisition, such as intraoral scans (IOS), facial scans, CBCT scans, and virtual interocclusal records; (ii) virtual facebow, registering digitized maxilla to the same 3D coordinate system to 3D facial scan using desired digital facebow techniques; (iii) virtual articulation, positioning all digital data (a complete 3D dental patient) onto a virtual articulator (Figure 11.6).
11.3.2 Digital Data Acquisition
In dentistry, commonly used digital data includes IOS, facial scans, CBCT scans or digital volumes, and virtual static or dynamic interocclusal records.

Figure 11.6 In a brief summarized digital workflow, three clinical steps are generally needed to unitize a virtual articulator. Clinicians need to obtain digital diagnostic datasets and virtual face bow record and create a 3D virtual patient with all digital datasets registered to the same 3D coordinate. Subsequently, a virtual articulator can be aligned with the 3D virtual patient using anatomical soft and hard tissue landmarks.
11.3.3 Intraoral Scans
Conventional impressions for dental procedures can be uncomfortable and poorly tolerated by patients. Further, the dimensional changes associated with conventional impression materials and gypsum products can introduce inaccuracies in dental treatment. To overcome these challenges and improve efficiency, digital workflow options have been developed. The use of intraoral scanners to obtain digital data is often considered to be the first step in digital workflow options and the fabrication of CAD/CAM prostheses. The use of IOS, a digital workflow, and CAD/CAM prostheses fabrication, provides improved communication between dental clinicians and technicians, reduced treatment times, decreased storage requirements for analog impressions and casts and reduced material and labor costs. As a result, in recent time, there has been an explosion of intraoral scanning technologies and research (Richert et al. 2017).
Intraoral scanners most often comprise a handheld wand, a computer unit, and an operating software program. The goal of IOS is to accurately capture the 3D surface geometry of an object. In dentistry, this includes relevant soft tissues and the remaining dentition in various states (for example, pre‐treatment, preparations, and post‐treatment). The first generation of IOS technology was based on stitching 2D images together in a slow scanning process. To facilitate surface capture, an opaque coating on the surface to be scanned was often required (Nikoyan and Patel 2020, Richert et al. 2017) (Figure 11.7). Reflective surfaces, such as enamel or polished restorative surfaces (metallic direct or indirect restorations), can disrupt the data acquisition process due to overexposure and result in voids on the composed 3D object. IOS utilizing opaque surface coatings has been shown to be accurate, although the process can be a time‐consuming step for clinicians. It is often difficult to apply an opaque powder or coating evenly and prevent contamination by saliva during the scanning process, and reapplication of the powder or coating is often needed. As a result of these challenges, most contemporary intraoral scanners have evolved and no longer require the use of an opaque layer (Richert et al. 2017) (Figure 11.8a,b).

Figure 11.7 Older models of intraoral scanners may require surface powdering to facilitate image capturing. It may be difficult to prevent surface contamination and maintain an even coating during the scanning process.

Figure 11.8 (a) New generation of intraoral scanners does not require the use of an opaque powdering layer during scanning. (b) Furthermore, new intraoral scanners (TRIOS; 3Shape A/S) are often capable of preserving the surface geometry, color, and texture of scanned objects. Proprietary digital file format (3shape DCM in this example) may be used to store these digital model attributes.
CAD/CAM systems can be divided into two categories based on data‐sharing freedom: closed and open systems. A closed system uses proprietary processes from data acquisition through virtual design to prosthesis manufacturing in a closed digital environment. It is difficult to deviate from the predefined workflow in a closed system, and the flexibility of sharing digital data among different digital ecosystems is very low. However, a closed system provides a well‐defined service channel utilizing only one or specifically identified vendor for products or services. In contrast, an open system allows dental clinicians and technicians to obtain digital data in universally used file formats and enables data sharing among different digital platforms offered by various vendors (Ting‐Shu and Jian 2015).
Standard tessellation language (STL) is a widely used file format in the digital environment and can be utilized for IOS, 3D‐printing, and other CAD/CAM processes. The STL file format is supported by most digital software packages and represents the surface geometry of a 3D object without any representation of color, texture, or other common digital model attributes (Nikoyan and Patel 2020) (Figure 11.9a). The polygon file format (PLY) is another commonly used file format. The polygon file format is designed to be both flexible and portable, and it allows more comprehensive user‐defined elements and properties to be defined in a 3D object. Not only does the PLY file format document the surface geometry of a 3D object, but it also supports vertex colors, textures, and materials. Because of its simplicity and flexibility, the PLY file format has become increasingly popular in clinical dentistry and the academic and research world (McHenry and Bajcsy 2008) (Figure 11.9b).

Figure 11.9 (a) Standard tessellation language (STL) file is widely used in the digital environment but only represents the surface geometry of a 3D object without any representation of color, texture, or other common digital model attributes. (b) The polygon file format (PLY) file is getting more popular because it can preserve surface geometry, vertex colors, textures, and materials information in a 3D object. Unlike proprietary digital file format, such as 3shape DCM file, PLY format is accepted by most digital software programs
A high degree of accuracy should be incorporated into an IOS to utilize a virtual articulator successfully in the digital workflow. According to ISO 5725, accuracy can be defined by trueness and precision. Trueness refers to the closeness of agreement between test results and the true or desired reference value (validity). Precision refers to the closeness of agreement among test results (reliability) (Richert et al. 2017). Most current literature agrees that IOS can be sufficiently accurate to acquire digital diagnostic impressions for treatment planning purposes and short‐span prostheses in the partially edentulous scenario. Although patient satisfaction and preference are superior for IOS than those with conventional impression procedures, the accuracy and time efficiency of IOS are decreased for longer‐span indications, especially in the complete edentulous scenario. While acquiring IOS, dental clinicians should be mindful that span length, scanning sequence, scanned surface texture, and morphography may all affect the overall accuracy of IOS (Kihara et al. 2020, Michelinakis et al. 2021, Sawase and Kuroshima 2020).
11.3.4 Facial Scan
When using mechanical articulators, a facebow transfer record is used to register the patient’s maxillary arch to a selected reference plane and orient the maxillary cast in close relationship to the hinge axis of the condyles. Accurate facebow transfer allows articulated casts to better mimic the patient’s occlusal contacts. In addition, facebow transfer can be used to establish the appropriate maxillary position and orientation to a patient’s face or head for proper esthetic assessment and evaluation. Although there are different virtual facebow techniques proposed in the literature, it is common to see facial scans used in addition, to provide common registration points. Many authors refer to this process as a “virtual facebow” technique (Lam et al. 2016, Solaberrieta, Garmendia, et al. 2015). After the accurate alignment between facial and IOS, the virtual patient assembly can be aligned on the virtual articulator based on a reference plane identified through anatomic landmarks on the facial scans. Clinical examples of a virtual facebow transfer will be shown in the later section of this chapter.
Technologies used to obtain surface facial scans include laser‐based surface imaging and optics‐based imaging (stereophotogrammetry, structured light, and Moire´ fringe projection) (Schendel et al. 2011). Laser‐based imaging acquires 3D data via sending laser beams (spots or stripes) across the target and measuring the time it takes for the projected laser beams to return to scanner sensors. It often takes up to 30 seconds or more to obtain a facial scan using this method, and movement during the scanning process may increase the possibility of distortion, noise, and voids in the scanned data. Because the laser is used, patient’s eyes must be closed during scanning, and this may interfere with natural facial expression and distort any anatomical landmarks in the proximity of the eyes (Tzou and Frey 2011
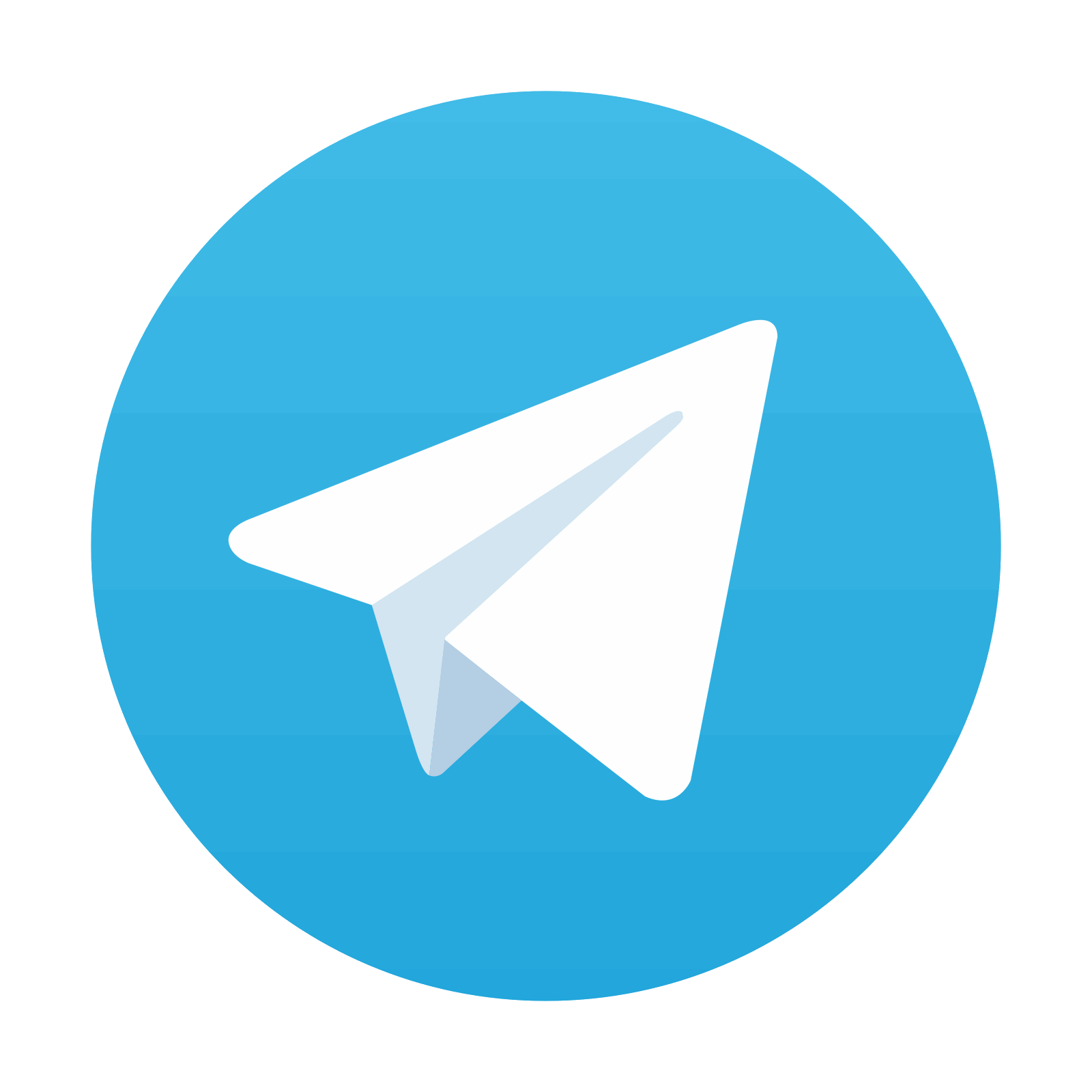
Stay updated, free dental videos. Join our Telegram channel

VIDEdental - Online dental courses
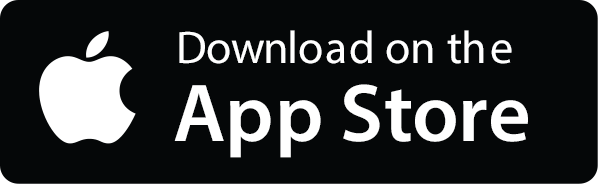
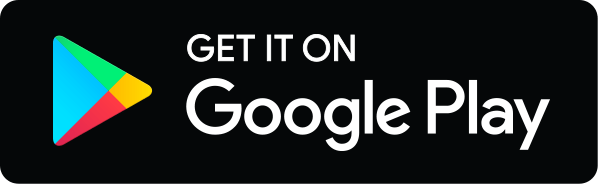