6
Clinical Applications of Digital Technology in Fixed Prosthodontics
Ramtin Sadid‐Zadeh
6.1 History of Computer‐Aided Design/Computer‐Aided Manufacturing Technology in Fixed Prosthodontics
In the early 1970s, a French dentist Dr. Francois Duret introduced computer‐aided design/computer‐aided manufacturing (CAD/CAM) concepts into the field of fixed prosthodontics. In 1984, he invented and patented the Duret system, demonstrating the ability of CAD/CAM to generate fixed dental prostheses (FDPs) (Rekow 1987). In the 1980s, Drs. Werner Mörmann and Marco Brandestini introduced the first commercial CAD/CAM system to a dental clinic in the University of Zurich in Switzerland (Mörmann et al. 1987). This breakthrough technology brought major changes into the practice of dentistry by permitting dentists to finish ceramic restorations in one clinical session (Fasbinder 2006). Also, several other research groups introduced their own systems between 1985 and 1990s (Mehl and Hickel 2009). The most developed of them was Diane Rekow’s DentiCAD system, also called the Minnesota system, which provided 3D measurement of teeth surfaces via stereo‐photogrammetry. Since then, a number of technologies have emerged for CAD/CAM’s use in the production of FDPs.
6.2 Current State of Computer‐Aided Restorations in Fixed Prosthodontics
Dental restorations fabricated with digital impressions present statistically similar marginal discrepancies to conventional impressions (Chochlidakis et al. 2016). Most CAD/CAM‐fabricated crowns have a clinically acceptable marginal gap of less than 150 μm, which makes them a viable alternative to conventionally fabricated crowns. Moreover, comparison reveals that the conventional workflow’s potential for error is higher due to its greater number of steps (Figure 6.1). It is also crucial to mention that both vertical and horizontal discrepancies characterize the marginal fit of restoration (Holmes et al. 1989). When studies report clinical acceptability for the marginal fit of CAD/CAM restorations, they refer to vertical discrepancies, as horizontal discrepancies are inevitable when manufacturing CAD/CAM restorations and can be modified after fabrication is complete (Figure 6.2).
Clinical research has not yet established how the various systems and software affect the marginal fit of CAD/CAM FDP. A review of the available literature reveals that many studies do not report important variables including the internal and marginal settings for the designed restorations or the milling strategy used for manufacturing restorations. This lack of information in the literature defy to generalize that CAD/CAM restorations produced by all CAD/CAM systems have a clinically acceptable marginal and internal fit. Instead, these reports relate to a specific CAD/CAM system with a defined CAD software setting and CAM milling strategy.

Figure 6.1 Commonly used workflows for the fabrication of restorations in fixed prosthodontics.
6.3 Factors Impacting The Quality of CAD/CAM Fixed Dental Prostheses
The clinical goal for a cemented restoration is a marginal discrepancy value between 25 and 40 μm (American Dental Association 1970); however, clinical evaluation of FDP suggests that typical marginal gaps – considered clinically acceptable for conventionally cemented restorations – range between 120 and 150 μm (Holmes et al. 1989, Fransson et al. 1985). Moreover, the marginal gap of crowns produced by multiple CAD/CAM systems is reported to be between 14 and 206.8 μm, suggesting that this range rarely encompasses acceptable values (Brawek et al. 2013, Tsirogiannis et al. 2016, Patzelt et al. 2014, Lim et al. 2018, Renne et al. 2017, In‐Sung et al. 2003, May et al. 1998, Neves et al. 2014, Renne et al. 2015, Ng et al. 2014, Alqahtani 2017, Yang et al. 2015, Sadid‐Zadeh et al. 2019, Hamza et al. 2013, Abdel‐Azim et al. 2015, Kim et al. 2016). Multiple factors influence the marginal gap of CAD/CAM FDP reported by different authors, including the quality of tooth preparation, the optical scanner, CAD software settings, manufacturing process (mode of milling, number of axes in the milling machine, and diameter of the cutting bur), and material used for the production of CAD/CAM crown (Renne et al. 2012, Brawek et al. 2013, Tsirogiannis et al. 2016, Patzelt et al. 2014, Lim et al. 2018, Sadid‐Zadeh et al. 2019, Renne et al. 2017, Hack et al. 2015).

Figure 6.2 Red arrows present horizontal discrepancy at the margin of a computer‐aided design/computer‐aided manufacturing monolithic zirconia crown.
6.3.1 Tooth Preparation
CAD/CAM and conventionally fabricated restorations share similar principles of tooth preparation. However, a number of adaptations are needed to accommodate the CAD/CAM system. Two recent publications assessed the quality of teeth prepared for zirconia FDP; they found that fewer than 7% of preparations fulfilled total occlusal convergence, undercuts, amount of occlusal reduction, axial height, and finish line design (Winkelmeyer et al. 2016, Güth et al. 2013b). However, a number of key principles required for CAD/CAM restorations – such as the presence or absence of rounded line angles, occlusal anatomy, and a smooth finish line – were not assessed in these studies. Recent data from 392 teeth prepared for monolithic zirconia crowns found that the majority of preparations had one or more errors that violated the principles of tooth preparation, and over 50% had an error specific to milled restorations, such as a rough, irregular, and stepped finish line and/or a non‐anatomical occlusal surface (Sadid‐Zadeh et al. 2021a) (Figure 6.3).
Finish line design has an impact on the marginal gap of CAD/CAM crowns; studies report an improved marginal fit when a modified shoulder is used. However, the marginal gap is not significantly impacted by the use of a chamfer finish line (Subasi et al. 2012, Euán et al. 2012, Re et al. 2014, Euán et al. 2014) or does a knife‐edge finish line (Cetik et al. 2017). Depending on the materials used, the finish line design may also impact the strength of CAD/CAM crowns. For example, CAD/CAM monolithic lithium disilicate crowns with a modified shoulder have a greater fracture strength than those with a chamfer finish line design. In addition to finish line design, the quality of the finish line is crucial to the marginal integrity of CAD/CAM crowns. A smooth and defined finish line produces a restoration with a smaller marginal gap than a rough finish line (Renne et al. 2012).

Figure 6.3 Distribution of errors found in teeth prepared for computer‐aided design/computer‐aided manufacturing of monolithic zirconia crowns.
Research results regarding the impact of finish line curvature on the marginal fit of CAD/CAM crowns are conflicting; however, the results favor finish lines with less curvature, which leads to a crown with a smaller marginal gap when compared with more curvature of the finish line. Studies assessing the impact of finish line curvature on the marginal fit of crowns were performed using two CAD/CAM systems, and the results may not be assumed to apply to current CAD/CAM systems (Tao and Han 2009, Asavapanumas and Leevailoj 2013). It is crucial to assess the impact of finish line curvature on the marginal gap of CAD/CAM crowns fabricated with recently developed CAD/CAM systems.
The presence of occlusal anatomy has a significant effect on the resistance form of restorations. Studies have shown that CAD/CAM crowns fabricated on preparations with anatomical occlusal form have a significantly smaller marginal gap than those fabricated on preparations with non‐anatomical forms (Habib et al. 2014, Sadid‐Zadeh et al. 2020b) (Table 6.1). The amount of occlusal reduction necessary for CAD/CAM restorations though is similar to conventionally fabricated restorations and depends on the material being used. The material’s strength, opacity, and nature determine the amount of occlusal and axial reduction required, with bilayered restorations requiring more tooth reduction than monolithic ones. However, a well‐rounded occlusal line angle is required to compensate diameter of burs cutting the intaglio surface of the restorations. Otherwise, the restoration is over‐ or under‐milled impaction internal or marginal adaptation of the restoration (Figure 6.4).
6.3.2 Optical Scanners
For the digital workflow in fixed prosthodontics, prepared teeth are either directly recorded with an intraoral optical scanner (IOS) (see Chapter 2) or their replica, the working casts and dies, are recorded using a desktop optical scanner (DOS) (Figure 6.2). A number of studies have confirmed the accuracy of IOSs and DOSs, supporting their use in fixed prosthodontics. Research has evaluated the trueness and precision of different IOSs, revealing that their accuracy is up to par with conventional impressions for recording short‐span areas (Nedelcu et al. 2018, Ren et al. 2020); however, DOSs have better accuracy compared with IOSs for complete arch scans. The accuracy of virtual models captured by IOSs is impacted by factors such as operator experience, software version, scanning protocol, type of substrate, and the length of the arch being scanned (Ender and Mehl 2013a, 2013b, Müller et al. 2016, Medina‐Sotomayor et al. 2018, Dutton et al. 2020, Resende et al. 2021). However, the accuracy of virtual models produced by DOSs is influenced by camera resolution, the number of cameras, software version, and the accuracy of conventional impressions and the working casts and dies. For additional information on the accuracy of optical scanners, please refer to Chapter 2.
Table 6.1 Marginal gap (μm) of computer‐aided design/computer‐aided manufacturing crowns for teeth prepared with various anatomical occlusal form.
Sadid‐Zadeh et al. 2020b | Intercuspal angulation | 100° | 110° | 120° | 140° | 160° | 180° |
Mean ± SD (μm) | 43 ± 30 | 43 ± 20 | 43 ± 30 | 59 ± 40 | 40 ± 20 | 72 ± 40 | |
Habib et al. 2014 | Occlusal anatomy | Anatomical | Semi‐anatomical | Non‐anatomical | |||
Mean ± SD (μm) | 121 ± 62 | 134 ± 69 | 159 ± 63 |
The quality of images produced by optical scanners may be tied to that of CAD/CAM restorations (Patzelt et al. 2014). However, it should not be generally assumed that CAD/CAM restorations fabricated using DOSs have better marginal and internal fit than those produced by using IOSs, or vice‐versa. As a result, even if studies have concluded that CAD/CAM single crowns and three‐unit partial FDP have a clinically acceptable marginal and internal fit (Abdel‐Azim et al. 2015, Pedroche et al. 2016, Su and Sun 2016, Shembesh et al. 2017, Arezoobakhsh et al. 2020), the results should be interpreted for the specific IOS, and DOS used, and the outcomes should not be extended to all the systems. Figure 6.5 shows examples of virtual models produced by the TRIOS (TRIOS3; 3ShapeA/S) and Planscan (Planmeca USA) intraoral scanners. CAD/CAM lithium disilicate crowns fabricated using the TRIOS scanner demonstrated better marginal and internal fit than those of the Planscan system (Sadid‐Zadeh et al. 2020a). However, this data cannot be applied to newer versions of IOSs from the same manufacturers. Providers must assess and compare clinical outcomes for specific IOSs to produce restorations with the best marginal and internal fit.

Figure 6.4 Prepared tooth with a sharp line angle and over reduced intaglio surface of a simulated crown (a); two‐dimensional cross‐section of the prepared tooth and coping at mesial (b); ill‐adapted coping (light brown line) at the sharp cusp of the prepared tooth (black line) (c).
6.3.3 Computer‐Aided Design
Appropriate parameters in the computer‐aided design (CAD) software must be applied to the virtual restoration to ensure the optimum marginal and internal fit of the produced CAD/CAM crown. Despite the importance of these parameters, only a few studies assessing the marginal and internal fit of CAD/CAM restorations have reported details of the parameters used. A practitioner must learn the parameters defined in the CAD software used in their practice to ensure high‐quality work is produced for patient care. Defined parameters differ in varying CAD software. A simplified example of internal parameters can be found in PlanDesign software (Planmeca USA), wherein the “spacer thickness” and “marginal ramp” by default are set to 100 and 250 μm, respectively (Figure 6.6). In PlanDesign software, the spacer thickness is defined as the space between the intaglio surface of the designed restoration and the virtual die; the margin ramp is defined as the length of the margin on the designed restoration in close contact with the finish line of the virtual die. When these parameters are modified from the default to fabricate restorations with an acceptable resistance form, the marginal gap can be affected. For example, Renne et al. (2012) and Neves et al. (2014) applied the default settings of internal parameters to fabricate CAD/CAM lithium disilicate crowns, and manufactured crowns with a marginal gap of 62.3 and 66.9 μm, respectively, without assessing the resistance form of crowns. However, when Sadid‐Zadeh et al. (2020a) applied stringent internal parameters (die spacer = 50 μm; margin ramp = 0.8 mm) to fabricate restorations with a clinically acceptable resistance form, the marginal gap increased to 123.7 μm.

Figure 6.5 Quality of virtual models produced by two intraoral scanners: TRIOS3 (a) and Planscan (b) observed by STL viewer software (3dviewer.net).

Figure 6.6 Internal parameters used for designed restoration in PlanDesign software. Spacer thickness (left); margin ramp (right).
Figure 6.7 shows another example of the internal parameters for CAD software (Dental System; 3Shape A/S). The parameters for this software are more elaborate. Here, the die spacer is defined as “cement gap,” the gap at the margin is defined as “extra cement gap,” the distance from the finish line and the base of the axial wall is defined as the “distance to margin line,” and the area above the base of the axial wall, which ensures a smooth transition between the two parts of the interface that have different offsets is referred to as “smooth distance.” When Kale et al. (2016) assessed the impact of die spacers on the marginal gap of CAD/CAM crowns, they found that applying fewer than 50 μm of “cement gap” leads to a significantly larger marginal gap. The above examples present differences in internal parameters’ definitions and their importance in producing clinically acceptable marginal gaps and resistance forms.
6.3.4 Computer‐Aided Manufacturing
The compatibility of CAD software with CAM systems and its impact on the quality of produced crowns should also be considered. For example, Sadid‐Zadeh et al. (2020) reported that CAD/CAM‐fabricated single crowns using the Trios3 IOS and Dental System Software (3Shape/AS) are not compatible with PlanMill40 (Planmeca USA) when using standard milling strategy. Their pilot study found that this workflow results in restorations with a visually significant open margin. In addition to CAD and CAM compatibility, the type of milling machine, its number of axes, and milling strategy are also essential factors impacting the quality of CAD/CAM restorations.

Figure 6.7 Internal parameters used for designed restoration in Dental System software. Cement gap (a); extra cement gap (b); distance to margin line (c); smooth distance (d).

Figure 6.8 Burs used in PlanMill 40 for milling of restorations (left); burs used in PrograMill PM7 suitable for milling zirconia restorations (right): ellipsoidal (a); conical (b); tapered (c); 0.5 mm (d); 1 mm (e); 2.5 mm (f).
Milling machines use various burs and strategies to detail the external and intaglio surface of restorations (Figure 6.8). Both the milling machine and its strategy may impact the marginal gap and the internal adaptation of the restoration. For example, a 3‐axis milling machine (PlanMill40; Planmeca USA) has two strategies for milling restorations: standard and detailed. The detailed strategy uses a diamond bur with a 1 mm diameter, while the standard strategy uses a 1.6 mm bur to cut the intaglio surface of restorations. As seen in Figure 6.9, the internal adaptation of the design restoration, specifically at the cusp tip, is affected by the milling strategy. Designed restorations simulated with the detailed milling strategy have better internal adaptation than ones simulated with the standard milling strategy. Also, restorations produced by the detailed strategy of PlanMill40 have a significantly smaller marginal gap and better resistance form than those fabricated with the standard milling strategy (Sadid‐Zadeh et al. 2020a).

Figure 6.9 Simulation of milled restoration in PlanDesign software presenting the internal adaptation of crown to die (red box) in the detailed strategy (a); standard strategy (b) along with the bur used for cutting the intaglio surface of the crown for each milling strategy.
The number of axes in a given milling machine may also affect the marginal gap of manufactured restorations. Hamza and Sherif (2017) assessed the marginal gap of zirconia crowns fabricated using five milling machines, comparing 4‐axis and 5‐axis models, and concluded that the 5‐axis milling machine produced significantly better marginal gaps. However, zirconia billets from varying manufacturers were used in this study, which makes it difficult to conclude if the effect is attributable to the milling machine or to the difference in materials. Conversely, Sadid‐Zadeh et al. (2019) found that a 3‐axis milling machine at the detailed milling strategy (PlanMill40; Planmeca USA) produced significantly better lithium disilicate restorations than a 5‐axis milling machine in the millin strategy of “cavity finishing 3‐2” with “additional internal preparation detail” (Roland DGA Corporation; Irvine, CA). However, these data cannot be applied across milling machines. These studies do, however, inform us that different milling machines produce varying marginal gaps, and the practitioner should be aware of this impact. Clinicians must assess a wide variety of factors to produce high‐quality CAD/CAM restorations.
6.4 Materials Used for CAD/CAM Fixed Dental Prostheses
Materials used in digital workflow for CAD/CAM FDP are divided into three basic categories: die materials, pattern materials, and restorative materials. Die materials are used to manufacture working casts with or without removable dies (Figure 6.10). The manufactured working cast provides an opportunity to assess the marginal integrity and interproximal contact of CAD/CAM restorations. Pattern materials allow a practitioner to take advantage of the digital workflow when fabricating restorations through the conventional cast or press technique. Finally, the wide variety of available restorative materials allows practitioners to make selections based on the necessary strength and esthetics of the material and the clinical presentation of patients.

Figure 6.10 Three‐dimensional printed working cast with removable dies (a); solid cast (b).
6.4.1 Die Materials
The quality of FDP is determined by marginal and internal fit as well as appropriate occlusal and interproximal contacts (Kuhn et al. 2015, Mously et al. 2014, Kale et al. 2016, Chochlidakis et al. 2016, Anadioti et al. 2014, McLean and von Fraunhofer 1971). Accurate impressions are necessary for replicating the prepared and neighboring teeth, which are essential to ensure a precise fit of the fabricated restoration (Persson et al. 2008, Ender and Mehl 2013a, Cho et al. 2015, Güth et al. 2013a). An inaccurate impression or replica, on the other hand, leads to a restoration that can be compromised in the aspects of internal and marginal adaptation and/or interproximal and occlusal contacts.
Conventionally, the above aspects of fabricated restorations are assessed and modified on working casts and dies fabricated from the conventional definitive impression. When a partial digital workflow (Figure 6.1) is used, CAD/CAM restorations could also be assessed and modified on conventional working casts and dies; however, no physical model exists for the assessment and modification in the complete digital workflow (Figure 6.1). Apart from internal adaptation, vertical gap, and interproximal and occlusal contacts, which should be assessed and modified for all FDP, CAD/CAM FDP has integral horizontal discrepancies requiring modification (Holmes et al. 1989). The assessment and modification of CAD/CAM restoration fabricated through a complete digital workflow could be done at the chairside by the practitioner or at the laboratory side by the laboratory technician. Chairside modifications are time‐consuming and sometimes difficult due to the presence of surrounding soft and hard tissue. On the other hand, alterations in the laboratory side require CAD/CAM working casts and dies manufactured from digital impressions. Irrespective of the fabrication method, working casts and dies must:
- Replicate the prepared tooth’s geometry and that of the surrounding soft and hard tissue
- Be free of voids and distortions
- Allow adequate access and visualization of the margin
- Have high strength and good abrasion resistance
- Demonstrate dimensional stability over time
In partial digital workflow (Figure 6.1), conventional working casts and dies are recorded using a DOS. The variety of gypsum color appears to have no impact on the accuracy of dies or the fit of CAD/CAM crowns (Kim et al. 2015, Tan et al. 2018). It should be noted that in the conventional workflow, high‐expansion gypsum is needed to compensate for the shrinkage of the cast restoration; conversely CAD/CAM restorations may benefit from low‐expansion gypsum for creating a die with dimensional geometry closer to that of the prepared tooth.
In a complete digital workflow (Figure 6.1), virtual models are used to design and fabricate restorations. Virtual models have multiple advantages over conventional working casts and dies, including the ability to digitally manipulate the model by subtracting and recapturing digital impressions to resolve isolated errors (Figure 6.11), storing the model without the need for physical space, and ease of sharing data. A virtual model can be converted into an analog working cast and die by subtractive or additive techniques if necessary. Then, CAD/CAM working casts and dies may be used to assess and modify the restoration at the margin and interproximal/occlusal contacts.
Fabrication of complex shapes such as working casts and dies with subtractive technique, also referred to as milling, is difficult, as the axes of milling machines are limited (Liu et al. 2006). Furthermore, the use of subtractive technique, also referred to as three‐dimensional (3D) printing, results in significant waste, and errors may occur due to the diameter of burs used in milling machines (Örtorp et al. 2011). In contrast, additive technique (described in Chapter 3) transforms virtual model files into slice data and builds them layer by layer to create the desired shapes; minimal material is wasted, and features such as undercuts and complex geometry are easily replicated (Liu et al. 2006). Both the additive and subtractive techniques use resin to create CAD/CAM working casts and dies and produce dies with high strength and good abrasion resistance. Although casts fabricated via the additive technique have inferior accuracy compared with conventional gypsum models; FDP fabricated from these models have clinically acceptable marginal integrity and internal fit (Jang et al. 2020). Inaccuracies in CAD/CAM working casts and dies increase as the length of the arch being scanned and printed increases. This may be due to inaccuracy in digital scans, or the shrinkage of resin materials during the subtractive technique processing (Park et al. 2020). Despite these shortcomings, when a complete digital workflow is used to fabricate a FDP, 3D printed working cast and dies are used to assess and modify internal/marginal discrepancies and interproximal/occlusal contacts (Figure 6.12). Ongoing research continues to create 3D printed working casts and dies that are as – or more – accurate than their gypsum counterparts. For more information regarding 3D printing, refer to Chapter 3.

Figure 6.11 Error in recording margin of prepared teeth no. 6 and no. 7 by an intraoral optical scanner (IOS). Virtual model at margin is deleted (a); three‐dimensional images are recaptured with an IOS (b).

Figure 6.12 Digital model with designed crown (a); three‐dimensional printed resin working cast with computer‐aided design/computer‐aided manufacturing of ceramic crown (b).
6.4.2 Pattern Materials
Patterns are conventionally formed freehand from wax for the fabrication of cast or pressed FDP. However, wax patterns can now be manufactured using the subtractive or additive technique (Figure 6.13). The pattern materials are mainly composed of polymers and wax, and CAD/CAM patterns have a number of advantages over conventional patterns; they are strong, unaffected by variations in temperature, they are time‐ and cost‐effective, and they do not rely on the laboratory technician experience. Wax patterns can be used for the fabrication of large or complex frameworks, where small distortions in a connector can compromise the fit of the entire framework. Subtractive fabrication of patterns is limited by factors such as the complexity of the restoration; however, the additive technique can be used to manufacture patterns with complex shapes. The CAD/CAM wax pattern can later be used to fabricate FDP by cast or pressed technique.

Figure 6.13 Wax pattern manufactured by subtractive technique for a conventional cast/pressed single crown; full contour (a); cutback (b).
Three‐dimensional printed and milled patterns have demonstrated clinically acceptable marginal fit in fixed prosthodontics and offer similar or superior internal and marginal fit (Lövgren et al. 2017, Homsy et al. 2018) compared with conventionally fabricated patterns. In addition, they produce crowns with similar or better marginal fit compared with conventionally produced wax patterns or milled crowns (Örtorp et al. 2011, Ghodsi et al. 2019, Sadid‐Zadeh et al. 2019, Koutsoukis et al. 2015, Revilla‐León et al. 2018). However, it is crucial to mention that the outcome of a CAD/CAM wax pattern – and, consequently, a FDP – is dependent on the quality of tooth preparation, quality of the digital impression, internal parameter settings in the CAD software, and the quality of manufacturing. In addition, the quality of the definitive restoration fabricated through this workflow depends on the laboratory technician’s skill in casting or pressing the CAD/CAM pattern.
6.4.3 Restorative Materials
Several restorative materials are developed for the fabrication of CAD/CAM FDPs to be manufactured by additive or subtractive techniques. Additively fabricated fixed restorations are limited to polymers and metal alloys (refer to Chapter 3 for materials used in additive technology). However, dental ceramics fabricated through additive manufacturing are being explored and have demonstrated encouraging primary outcomes for fabricating ceramic FDP. (Dehurtevent et al. 2017, Wang et al. 2019, Methani et al. 2020, Revilla‐León et al. 2020)
In the subtractive technique, varying sizes of billets are produced under controlled conditions to increase the material’s homogeneity and reduce microcracks and voids, which weaken the mechanical property of the material. Then, restorations are milled from billets at the chairside or in the dental laboratory (Figure 6.14). Subtractive techniques may make use of polymethyl methacrylate (PMMA), composite resin, ceramics, polyetheretherketone (PEEK), and metal alloys. In subtractive technique restorative materials are milled in either a dry or wet‐milling environment. In dry milling, materials are machined without the need for a cooling agent. Dry milling is required for materials such as partially sintered zirconium oxide. In wet milling, coolant is sprayed during manufacturing to protect the burs and material against overheating. The latter technique is necessary for manufacturing metal alloys and glass ceramics.

Figure 6.14 Various sizes of billets for manufacturing fixed dental prostheses by subtractive technique.
6.4.3.1 Polymethyl Methacrylate
Polymethyl methacrylate is a synthetic material produced from the polymerization of methyl methacrylate. Interim FDPs can be fabricated from PMMA billets by the subtractive technique. Depending on the milling machine used, PMMA FDP may be manufactured in a dry‐ or wet‐milling environment; PMMA materials used with subtractive technique possess better physical and mechanical properties than conventionally processed PMMA, including color stability, water absorption, wear, microhardness, flexural strength, and fracture resistance (Rayyan et al. 2015, Stawarczyk et al. 2012, Stawarczyk et al. 2013b, Song et al. 2020). The cytotoxicity of milled PMMA is also lower than that of conventional PMMA (Souza et al. 2020). These properties support PMMA interim restorations fabricated by subtractive technique for use as long‐term interim restorations (Figure 6.15).
Polymethyl methacrylate billets are used to manufacture interim restorations by indirect or indirect‐direct technique. Indirect techniques in the fabrication of CAD/CAM interim restorations require a digital record of prepared teeth, design of restorations on the virtual models, and manufacturing of CAD/CAM restorations. Then, the restorations are luted on the prepared teeth. However, in the indirect–direct technique, a shell is designed on the virtual models with unprepared teeth; then, the shell is relined intraorally with the conventional PMMA after tooth preparation and luted on the prepared tooth (Figure 6.16). CAD/CAM interim FDP fabricated by the indirect technique (Figure 6.15) are reported to have better marginal integrity and fracture strength than those fabricated conventionally (Peñate et al. 2015, Edelhoff et al. 2016, Reymus et al. 2020, Wimmer et al. 2014, Alt et al. 2011, Stawarczyk et al. 2012, Abdullah et al. 2016, Abdullah et al. 2018, Peng et al. 2020). However, interim partial FDPs fabricated by an indirect–direct technique using CAD/CAM and conventionally fabricated shells have not shown a significant difference in the fracture strength of FDPs (Sadid‐Zadeh et al. 2021b).

Figure 6.15 Single crowns designed on minimally prepared teeth of a patient with Amelogenesis imperfecta (a) to mill polymethyl methacrylate crowns by indirect technique (b).
Source: Courtesy of Dr. Ariana Mendel, Prosthodontics residents at University at Buffalo.

Figure 6.16 Shell designed on unprepared maxillary anterior teeth (a); polymethyl methacrylate shell milled for the fabrication of interim fixed dental prosthesis by the indirect–direct technique (b).
6.4.3.2 Composite Resins
Composite resins are composed of organic resin reinforced with inorganic particle fillers. Direct composite resins are applied onto the tooth structure and polymerized intraorally with a light‐cure unit. Indirect composite resins are pre‐polymerized restorations that are luted to the tooth structure. The subtractive technique can be used to fabricate indirect composite resin restorations. This technique overcomes some shortcomings of direct composites, such as polymerization shrinkage, marginal microleakage, long‐term color instability, and low wear resistance.
Infiltrated ceramic resins are developed to improve the mechanical properties of composite resins. This category includes composite resins, in which a polymer matrix is infiltrated with ceramic filler particles (e.g. Lava Ultimate; 3M ESPE), and composite resins, in which ceramic network is infiltrated with polymer (e.g. Vita Enamic; VITA). The latter is a hybrid ceramic material composed of a structure‐sintered ceramic matrix with resin‐filled spaces between ceramic substrates, forming a double network hybrid. The mass percentage of inorganic ceramics is 86 wt.%, while the remaining 14 wt.% is an organic polymer (Leung et al. 2015). These materials have improved the mechanical properties of conventional composite resins (Shembish et al. 2016, Spitznagel et al. 2014).
Composite resins are easy to mill and polish. Restorations composed of them require processing only if adding photopolymerizable stains for characterization. They are indicated for the fabrication of long‐term interim crowns, definitive inlays, onlays, and single crowns. The minimum recommended reduction for complete coverage crowns is 1.5 mm at the occlusal/incisal and 1.0 mm at the circumferential; however, more conservative indirect restorations (such as laminate veneers) may be milled from these materials as thin as 0.2–0.4 mm, depending on the category of composite resins. These restorations are luted to the tooth structure. The bonding strategy varies by type of material; composite resins, in which ceramic network infiltrated by polymers – such as Vita Enamic (VITA) – are acid‐etched with 5% hydrofluoric acid for 60 seconds, after which silane is applied. However, composite resins containing a polymer matrix infiltrated with ceramic filler particles – such as Lava Ultimate (3M ESPE) – require air abrasion with 50 μm Al2O3 before silane is applied (Blatz et al. 2003).
CAD/CAM single crowns and partial coverage fixed restorations fabricated from composite resins with a ceramic network infiltrated by polymer (Vita Enamic; VITA), have a three‐year survival rate of 93.5% and 96.5%, respectively. Their failure is reported to occurred on molars, with crown fracture cited as the cause. Their success rate is reported to be lower than the survival rate due to marginal discoloration, decrease in marginal adaptation, and increase in surface roughness (Spitznagel et al. 2020, Spitznagel et al. 2018). Additionally, the five‐year success rate of composite resins containing a polymer matrix infiltrated with ceramic filler particles (Lava Ultimate; 3M ESPE) is similar to that of lucite‐based ceramics (Fasbinder et al. 2020). However, the performance of these materials is yet to be assessed in long‐term clinical trials.
6.4.3.3 Polyetheretherketone
Polyetheretherketone (PEEK) polymer has been used in medical devices for decades; however, high‐performance polymers have only recently been marketed for the fabrication of FDP (e.g. Juvera; Straumann and BioHPP; Bredent). These materials are found in a variety of compositions, including unfilled and filled with nanoceramic or titanium oxide fillers. PEEKs have a low elastic modulus of 4 GPa (similar to bone and dentin), making them an appropriate restorative option in implant dentistry. They have low solubility and water absorption (Liebermann et al. 2016), and their mechanical properties are favorable for use in load‐bearing areas; they also have better wear resistance than composite resin and PMMA (Wimmer et al. 2016, Alt et al. 2011, Stawarczyk et al. 2013a, Stawarczyk et al. 2015).
With regard to practical use, PEEKs can be easily polished and do not require post‐processing. They are indicated for single crowns and partial FDP in full contour or framework forms. Other materials such as composite, lithium disilicate, or zirconia can be bonded to a PEEK framework to complete the restoration. They can be wet‐ or dry‐milled following manufacturers’ recommendations. The recommended amount of reduction for this material is 1.5 mm at the occlusal/incisal and 1.0 mm at the circumferential; however, they can be milled as thin as 0.8 mm when used as a framework. Bonding procedures for these restorations include air abrasion with Al2O3, followed by the application of silane. Although these materials are commercially available for the fabrication of FDP, clinical trials are lacking to evaluate their long‐term success.
6.4.3.4 Silicate‐Based Ceramics
Silicate‐based ceramics contain both glass and crystalline forms of silica (SiO2). Dominant silica content provides translucency and can mimic the optical properties of enamel and dentin, making them ideal for restorations in the esthetic zone. However, it also makes them brittle, leading to low fracture resistance. Adhesive bonding is recommended for restorations fabricated from these materials to compensate for their brittleness. Silica‐based ceramics are also prone to chipping during the milling process; to minimize the risk of chipping, they are wet‐milled using diamond burs. Based on the type of crystalline, these ceramics are divided into three groups of feldspathic, leucite based, and lithium disilicate based ceramics.
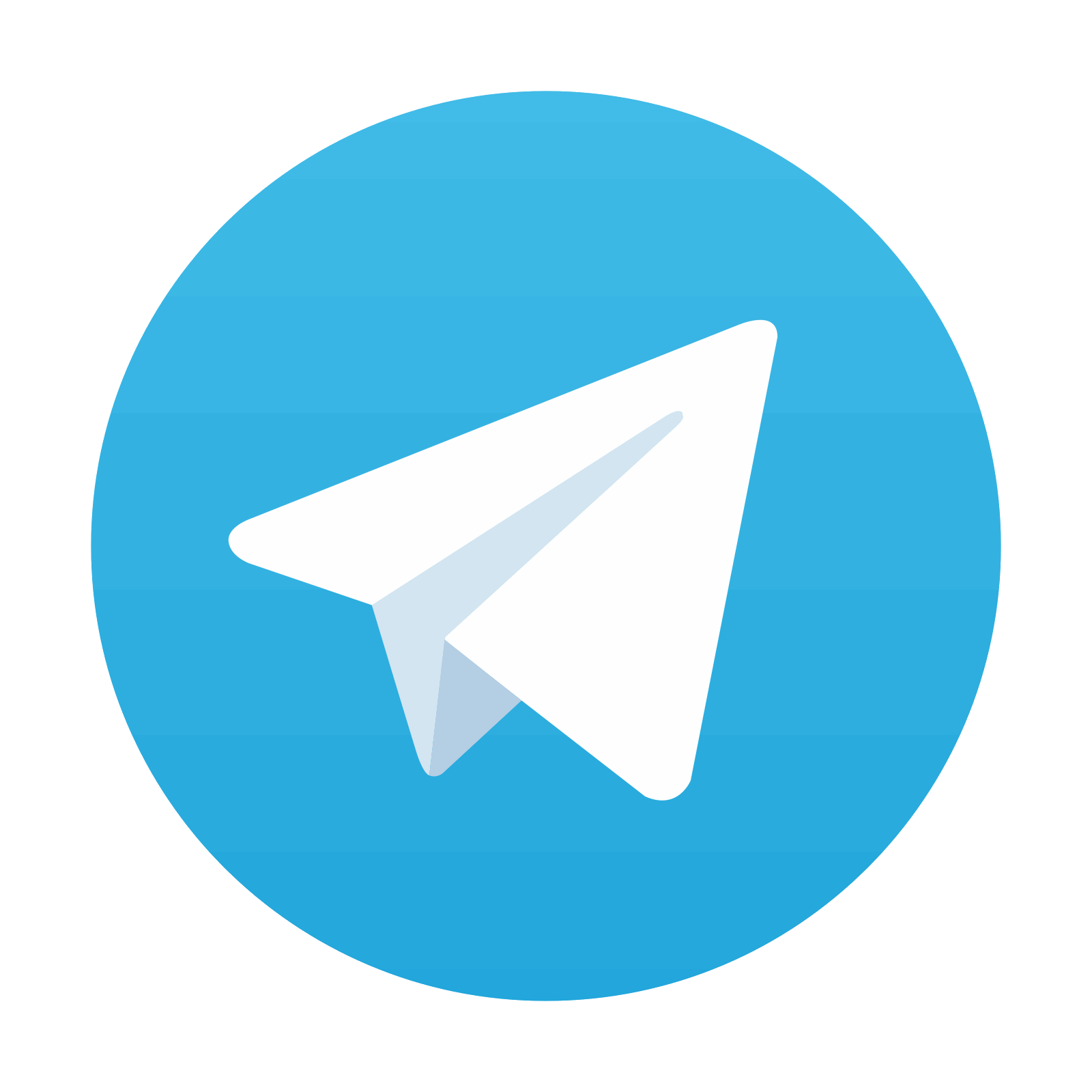
Stay updated, free dental videos. Join our Telegram channel

VIDEdental - Online dental courses
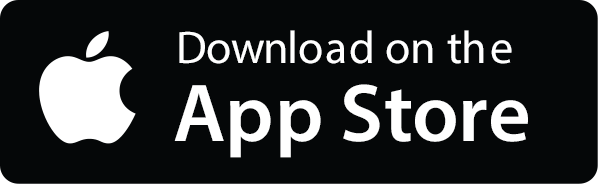
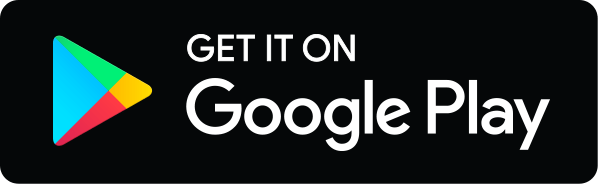