8
Clinical Applications of Digital Dental Technology in Removable Partial Prosthodontics
Scott Hollis and David R. Cagna
8.1 Introduction
Computer‐aided technologies have changed the landscape in dentistry over the past several decades, playing an important role in every aspect of dentistry, including removable prosthodontics. This chapter provides an overview of several digital advances in the design and fabrication of removable partial denture (RPD) frameworks.
8.2 A Brief Historical Perspective
Edentulism in the modern world has steadily declined since the introduction of preventive dental care in the mid‐twentieth century (Douglass and Watson 2002). Conversely, partial edentulism has steadily increased likely due to a better understanding of dental disease, increased life expectancy, and decreased tooth loss (Kim 2019). With the widespread introduction of endosseous dental implants in the 1980s, additional prosthodontic solutions became available for those affected by partial edentulism. However, the cost of implant therapy and implant‐assisted restorations may be prohibitive, limiting patient access and acceptance (Campbell et al. 2017).
Traditionally, RPDs have been manufactured using a lost wax protocol to cast materials such as Type IV gold alloy, nickel–chromium (Ni–Cr) alloy, cobalt–chromium (Co–Cr) alloy, and vanadium‐free titanium alloy (Koeppen and Mansueto 2008). Type IV gold was replaced by chrome‐based alloys in the mid‐twentieth century due in part to cost and favorable material properties, such as high strength, high modulus of elasticity, and good corrosion resistance. The reduced expense of fabrication associated with chrome‐based alloys made it possible to offer a more cost‐effective solution in the management of partial edentulism.
Unfortunately, constituents of the base metal alloys used in RPD framework fabrication, such as beryllium and nickel, have been associated with allergic and more severe reactions. Technicians appear to be particularly at risk during finishing and polishing procedures in poorly ventilated dental laboratories. This untoward consequence has encouraged the search for more biocompatible material and environmentally healthy processes (Haberman et al. 1993, Chandu et al. 2014, Syed et al. 2015). For this reason, titanium‐based alloys were introduced as an alternative casting material for framework fabrication. These alloys exhibit excellent mechanical properties, such as corrosion and wear resistance, high strength, and favorable ductility. However, a technique‐sensitive casting protocol and the inherent problem of porosity within cast titanium alloy frameworks led the profession to identify more predictable solutions. More recently, research has centered on computer‐aided design and computer‐aided manufacturing (CAD/CAM) technologies that offer both controlled processing and interesting new material choices for predictable framework outcomes (Mori et al. 1997, Baltag et al. 2002, Ohkubo et al. 2008).
8.3 Introduction of CAD/CAM Technologies
CAD/CAM technologies have had a substantially presence in the dental market since the early 1980s. Initial systems relied on numeric control machine milling for indirect dental restorations, typically single‐unit crown restorations, from solid block materials (Mörmann 2004). Quickly, the art and science of dental CAD/CAM technology evolved. Traditional industrial manufacturing techniques were scaled, modified, and implemented in the production of fixed and removable dental prostheses. Computer‐based processes were soon available to facilitate the design and fabrication of RPD frameworks using both subtractive and additive manufacturing techniques to produce definitive prostheses or resin/wax patterns for use in conventional lost wax casting. Of the many potential advantages that quickly became apparent in this expanding dental CAD/CAM environment, two of note include significant time savings at the laboratory bench and improved technician safety during material processing and finishing.
Sophisticated scanners and design software (Dental System, 3Shape Inc., New Providence, NJ; DentalCAD, exocad America Inc., Woburn, MA) have been developed to capture and manage digital data within the CAD/CAM process. Both software and digital equipment manufacturers, have contributed to the development of unique and effective workflows for RPD framework fabrication. In general, dental practitioners and dental laboratories begin by creating a three‐dimensional digital dental cast from a scan of the definitive conventional impression or a scan of the definitive dental cast using laboratory‐based scanning techniques (Campbell et al. 2017). This digital file is then uploaded to CAD software for cast surveyed and RPD framework design. This CAD file is then exported to digital‐based subtractive or additive manufacturing processes.
8.4 Subtractive Manufacturing Technology for RPD Frameworks
Subtractive manufacturing technology (SMT; e.g. computer numeric controlled (CNC) milling) has been used for dental restoration fabrication for over 40 years. Materials used in SMT for RPD frameworks include titanium‐based alloys, cobalt–chromium alloys, polymers, and waxes. Dental alloys (e.g. titanium alloy and Co–Cr alloy) have been used in subtractive manufacturing of dental prosthesis frameworks for decades (Drago et al. 2012). Suggested advantages of milling metals for dental applications include: (i) parent alloy and structurally homogeneous, (ii) theoretical potential for highly accurate framework fit, (iii) reduced production time, and (iv) improved patient satisfaction (Arnold et al. 2018). Disadvantages may include: (i) potential for high‐volume material waste, (ii) high cost of the technology, and (iii) potential inaccuracy of the framework due to damaged or worn cutting tools and operator error (Ohkubo et al. 2008). To overcome the potential problems associated with “hard” milling, Hong et al. (2020) reported promising results with “soft” milling and then sintering milled Co–Cr alloy frameworks. As illustrated in Figures 8.1–8.16, a CAD/CAM workflow that includes CNC milling of Co–Cr alloy can produce clinically acceptable results.

Figure 8.1 According to the dentist’s prescription. The digital mandibular cast is surveyed. Parallel, shaped, and arbitrary digital blockout is accomplished according to the prescribed removable partial denture design. Finally, relief is appropriately applied in edentulous areas.

Figure 8.2 Framework components are sequentially added to complete the digital design in the preparation for Co–Cr manufacturing via computer numeric controlled milling. Note the absence of clasps on abutments #20 and #29. Following the milling process, 18 gauge wrought wire clasps will be prepared and attached using conventional soldering/welding technique.

Figure 8.3 Maxillary master cast is surveyed, blocked‐out, and relieved according to the prescribed design in preparation for manufacturing.

Figure 8.4 Completed maxillary CAD framework is ready for Co–Cr milling.

Figure 8.5 Highly automated computer numeric controlled Röders TEC RXD5 high‐speed cutting five‐axis dental milling machine equipped with RCS‐1 automation robot and magazine (Röders GmbH, Germany).

Figure 8.6 One‐sided clamping of Co–Cr stock within the fixture permits efficient use of the available material. The parent material is automatically clamped on the rotary axis of the mill.

Figure 8.7 Milling production of a mandibular RPD framework. The machine’s integrated highly dynamic rotary swivel unit permits simultaneous five‐axis machining, positioned freely.

Figure 8.8 With milling production completed, the RPD framework is separated from its Co–Cr parent stock and forwarded for finishing and polishing (note the milling time of 2 : 13 : 02).

Figure 8.9 Note the exceptional quality of the as‐milled cameo surfaces of the maxillary framework (before finishing and polishing).

Figure 8.10 Note the exceptional quality of the as‐milled intaglio surface of the maxillary framework (before finishing and polishing).

Figure 8.11 Note the exceptional quality of the as‐milled cameo surface of the mandibular framework (before finishing and polishing). Also note that 18‐gauge wrought wire circumferential clasps (abutments #20 and #29) have been contoured and soldered/welded to the framework using conventional processing.

Figure 8.12 Note the exceptional quality of the as‐milled intaglio surface of the mandibular framework (before finishing and polishing).

Figure 8.13 Final Cr–Co framework on the master cast demonstrating the appearance of an exceptionally good fit.

Figure 8.14 Close‐up occlusal view of right‐side clasp assemblies. Note intimate adaptation of clasp arms and plate to abutments. Rests appear to fit accurately into their respective rest seats. Major connector appears well adapted to the palate.

Figure 8.15 Close‐up facial view of right‐side direct retainers. Clasp arms generally appear to be properly dimensioned and appropriately positioned as prescribed.

Figure 8.16 Close‐up image from a distal perspective of a molar clasp assembly. The direct retainer, rest, and reciprocal plate appear to be well adapted, of appropriate dimensions, and accurately fitting.
Polymers have had a long and extremely successful history in prosthodontics. As such, polymers have also been used in the digital fabrication of RPD frameworks due to desirable mechanical properties and ease of fabrication (Campbell et al. 2017). Polyoxymethylene (POM), also known as acetal or poly‐acetal resin, was introduced as a denture base and retentive clasp material in 1986 (Arikan et al. 2010). In 1999, Turner et al. suggested POM to be a suitable substitute for RPD metal direct retainer clasp arms. Favorable mechanical properties of POM include high strength, acceptable stiffness, good hardness, reasonable impact strength, low coefficient of friction, high wear resistance, reasonable dimensional stability, and low water sorption (Schierz et al. 2021). Additional advantages include favorable biocompatibility, lack of metallic taste, and low plaque affinity. POM comes in a variety of tooth color shades providing an esthetic option to traditional metal frameworks.
Potential disadvantages of POM include low thermal conductivity, color instability, reduced mechanical strength, and hastened degradation in the oral environment compared with conventional metal alloys (Papathanasiou et al. 2021). Modern methods of processing POM include CNC milling. Figures 8.17–8.27 illustrate a CAD/CAM workflow that incorporates milling of acetal resin. However, practitioners should consider potential shortcomings of this workflow, including suboptimal framework fit and excessive framework component dimensions, as illustrated in Figures 8.28–8.35.

Figure 8.17 Maxillary framework design is developed by the dentist, recorded, and submitted for fabrication. Master cast is scanned (in Eos X5 scanner, Dentsply Sirona, USA) in preparation for CAD. The maxillary master casts is beaded (approx. 0.5 mm deep) prior to scanning.

Figure 8.18 Scanned cast is digitally surveyed according to the prescribed design. Parallel (or tapered) blockout, shaped (ledging) blockout, arbitrary blockout, and relief is applied to accommodate prescription.

Figure 8.19 Framework components are sequentially added to the blocked‐out and relieved digital working cast. Each is carefully positioned and contoured to satisfy the dentist’s prescription.

Figure 8.20 Completed maxillary CAD framework.

Figure 8.21 Mandibular framework design is developed by the dentist, recorded, and submitted for laboratory fabrication. Master cast is scanned in preparation for CAD.

Figure 8.22 Scanned cast is digitally surveyed according to the prescribed framework design. Parallel (or tapered) blockout, shaped (ledging) blockout, arbitrary blockout, and relief are applied.

Figure 8.23 Framework components are sequentially added to the prepared digital working cast. Each is carefully positioned and contoured to satisfy the prescribed design.

Figure 8.24 Completed mandibular CAD framework.

Figure 8.25 Acetal framework manufacturing will be accomplished in the ZENOTEC® Select Hybrid CNC milling machine capable of five‐axis simultaneous operation (Wieland Dental+Technik GmbH & Co., Germany).

Figure 8.26 Parent acetal material is clamped into the fixture within the machine milling chamber in preparation for manufacturing.

Figure 8.27 Still tethered within the parent material, milling of these acetal frameworks is complete.

Figure 8.28 Completed acetal maxillary framework fitted to the master cast (cameo surface view).

Figure 8.29 Completed acetal mandibular framework fitted to the master cast (cameo surface view).

Figure 8.30 Note less than optimal marginal fit along lingual platting and rests. The dentist’s prescription indicated 18 gauge round clasps. This system (manufacturing method and material) cannot accommodate this request. Therefore, half‐round clasps of greater dimension were provided.

Figure 8.31 Again, note less than optimal marginal fit along lingual platting and the rest on tooth #21.

Figure 8.32 As required by both manufacturing method and material selection, note the excessively bulky circumferential clasp (tooth #20) in both vertical and horizontal dimensions.

Figure 8.33 Facial view of the excessively bulky circumferential clasp.
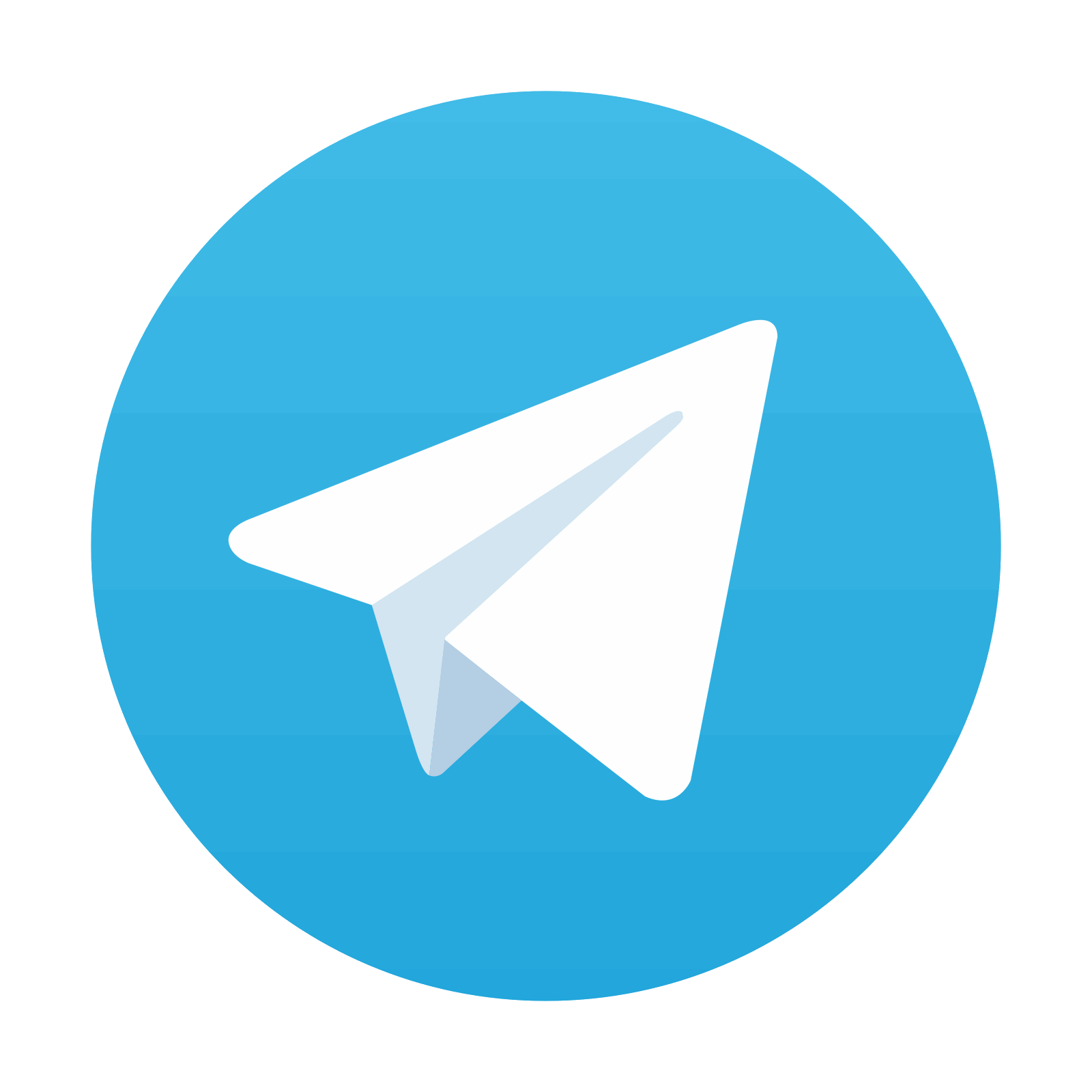
Stay updated, free dental videos. Join our Telegram channel

VIDEdental - Online dental courses
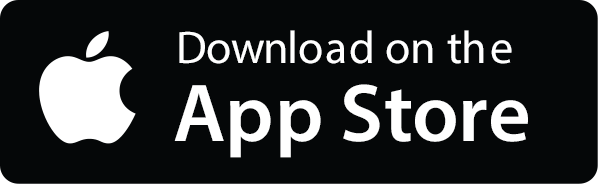
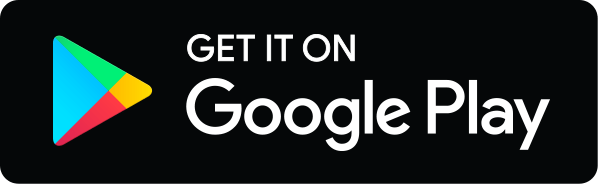