Materials used to support or replace diseased tissues, collectively called biomaterials , play an important role in craniomaxillofacial surgery. Collaboration among material scientists, biomaterial engineers, clinicians, and clinical investigators has accelerated understanding of the requirements and potentials of various implant materials. Alloplastic materials play an essential part in the reconstruction of function and contour in craniomaxillofacial surgery. Many different polymers, metals, ceramics, and composites are used as biomaterials. Some, such as osteosynthesis materials, are used to achieve a temporary goal; others, such as artificial joints, need to function for sometimes a lifetime.There are many requirements that biomaterials must meet if they are to form either a temporary or a lasting union with the part of the body being treated. Adequate strength or, more probably, suitable mechanical characteristics, is a necessary but not the only feature; chemical and electrical factors and biological responses all contribute to success or failure.
Very often, the primary requirement is mechanical strength. Biocompatibility is considered a secondary requirement, despite the fact that many biomaterials applications in the human eventually fail due to infection. In his Science paper, the famous biomaterial scientist, Antony G. Cristina, went as far as to call biomaterials in the human body a “microbial time bomb,” because they seem to have an almost “magnetic” action on infectious microorganisms. Whether a biomaterial becomes infected depends in part on what is described as a “race for the surface” between tissue cells and microorganisms. The so-called wetability of a biomaterial’s surface determines the outcome of this race, either full integration of an implant (when the race is won by tissue cells) or infection (when it is won by microorganisms) ( Fig. 30-1 ).
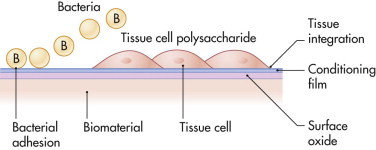
This chapter reviews the biomaterials most commonly used in craniomaxillofacial surgery.
Bone Substitutes
Bone grafts are increasingly used in cases of trauma, tumor surgery, and congenital absence or hypoplasia and for strictly esthetic purposes. Many surgeons prefer the use of autogenous bone grafts to reconstruct bony defects. Autogenous bone grafts have disadvantages including shortage of donor sites, donor site morbidity, growth deformity, and unpredictable resorption. The favorite donor sites for craniomaxillofacial reconstruction are calvaria, rib, and iliac bone. It seems that there is less resorption or at least slower with cranial bone than with rib or iliac bone. Over the past 20 years, many kinds of biomaterials have been developed that can be used as bone graft substitutes. They have demonstrated their usefulness in craniomaxillofacial reconstruction with their ability to augment and replace portions of the craniofacial skeleton.
Demineralized Bone
Demineralized bone can be used for reconstruction of craniomaxillofacial defects. The advantages of using demineralized bone are that it is pliable, easy to shape and to fit, available in limitless supply, and free from donor morbidity, which is particularly useful in children. Implantation of demineralized bone hardly affects tissue reaction and osteoclastic activity; 8 to 12 weeks after the implantation of demineralized bone, new bone formation was noticed in histological evaluations. Fragmentation of the implanted demineralized bone was observed 12 weeks postoperatively but in combination with new bone formation and without multinuclear cell activity. Hydrolytic enzymes may be the cause of this fragmentation. At 4 years after implantation, there were still large areas of nonvital bone without osteocytes in the bone lacunae and osteoblasts on the surface. Several areas showed fragmentation of the autogenous bone matrix. Several areas contiguous with nonvital bone showed evidence of transformation into living bone and remodeling. Active resorption, osteoclasts, and inflammation of fibrous changes were not observed in the long term.
Demineralized bone pastes are composed of living osteoblasts derived from homograft materials. Theoretically, they provide osteogenic cells capable of inducing osteogenesis. Demineralized bone pastes can be used alone or in combination with other materials such as hydroxyapatites. The advantage of such a combination is that it provides a structural supporting matrix with cells that have osteogenic ability.
Hydroxyapatite
Hydroxyapatite (HA) is the principal mineral component of bone and determines 60% of the calcified human skeleton. It has been manufactured synthetically for more than 30 years and has been in clinical use for at least 20 years. Certain marine corals consist of HA and have a structure that is similar to that of human bone. There are two forms of HA: ceramic and nonceramic. Nonceramic HA is not sintered after the HA crystals have been formed and therefore is more absorbable in vivo than the ceramic form. Ceramic forms of HA have excellent biocompatibility and show osteoconduction and osseointegration when placed in direct contact with viable bone. Osteoinduction is not evident because of the absence of inductive growth factors. Nonceramic HA can also be formed into cements, whereas ceramic HA cannot.
Ceramic Hydroxyapatite
Ceramic HA is synthesized in crystal form at low pH and then heated (sintered) at 700° C to 1300° C to form a solid mass of HA. Ceramic HA is available in two forms: dense and porous. The dense form is completely synthetic, it has no pores and can be fabricated into blocks or granules, which are difficult to shape and do not permit tissue ingrowth. Granules have greater contour adaptability than the solid blocks but have no intrinsic structural integrity and do not become mechanically stable until surrounded by fibro-osseous tissue. Dense HA granules are difficult to contain within the desired site of implantation, and there is a possibility of migration to unwanted areas after several months or years.
Porous HA can be produced synthetically, or it can be based on the skeletons of marine coral. The calcium carbonate skeleton of the coral is chemically converted to HA, with the original porous structure of the coral retained. Porous ceramic granules appear to be less prone to migration over time. Another means of preventing migration is to combine HA granules with resorbable carrier compounds. The very important advantage of porous HA is the ingrowth of fibro-osseous tissue: the implant becomes fixed to the surrounding bone within a few weeks. After completion of fibro-osseous tissue ingrowth, the implant consists of approximately 40% residual HA implant and 60% fibro-osseous tissue. Porous HA in block form is rather fragile and very difficult to contour ( Fig. 30-2 ). Therefore, its application has been limited in craniomaxillofacial surgery. Use of porous HA for alveolar ridge augmentation ( Fig. 30-3 ) has demonstrated that the implant is resistant to infection after fibro-osseous tissue ingrowth is finished, but it easily becomes exposed, especially if the overlying soft tissue is thin or compromised.
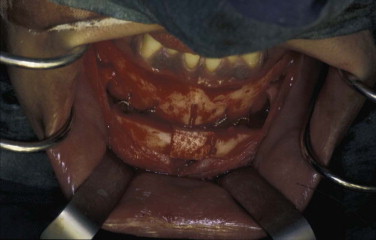
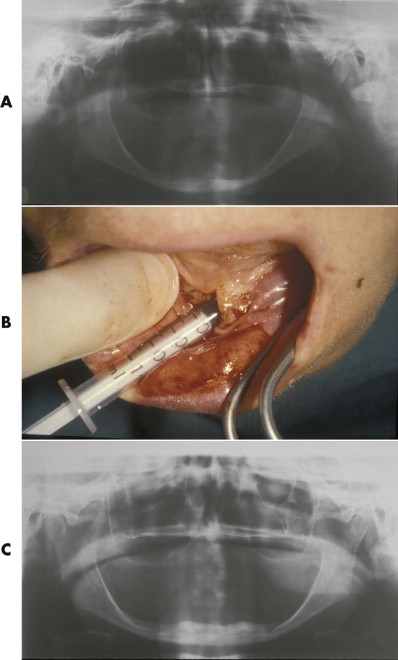
Porous ceramic HA can be chemically combined with various biomaterials to improve its physical properties. It can also be used as a carrier of bioactive substances such as bone morphogenetic proteins, which increase the ingrowth of bone into the pores.
Nonceramic Hydroxyapatite
Tetracalcium phosphate cement (HA cement) is a form of nonceramic HA. It is the only calcium phosphate cement that sets into a stable shape and is converted in vivo to pure HA. It can be produced by direct crystallization of HA at physiological pH and temperature and does not require heating to form a structurally stable implant. The dry cement is composed of tetracalcium phosphate and dicalcium phosphate. It sets in approximately 15 minutes and converts to HA within 4 hours. After conversion to HA it is no longer water soluble and is slowly replaced by bone over time. Its contour is stable. Animal studies proved that 35% of implanted HA was replaced by fibro-osseous tissue; after 12 months, bone replaced 75% of this fibro-osseous tissue. Even in human clinical trials, the cement was found to be functionally nonresorbable over 42 months on the basis of computed tomographic scans and incidentally by direct intraoperative inspections during secondary surgery.
These biologically active forms of HA cement are especially useful in situations where contour is not an important factor or where bone replacement of the implant material is necessary, such as reconstruction of skull base defects, orthopedic applications, and pediatric craniomaxillofacial surgery. HA cement may be used in the growing skull because it has been observed to have no adverse effects on development. HA has no toxic reactions, and it has a low rate of infection (about 4%) even when the HA implant is in contact with the paranasal sinuses or oral cavity. An important disadvantage of HA cement is that it is very difficult to give it the desired contour because it tends to settle with gravity during the setting process. HA granules may also be mixed with fibrocollagen and autogenous blood to form a paste that can be injected into subperiosteal pockets. It becomes firmly fixed by fibrous tissue ingrowth from the surrounding tissues. It has been used to augment the malar bone, premaxilla, nasal dorsum, and glabella area. Large volumes of this paste have been used to fill cranial defects in children. The infection rate was about 3%.
A mixture is on the market consisting of type I bovine dermal fibrillar collagen (PFC) and a mixture of 65% ceramic HA and 35% β-tricalcium phosphate (TCP) granules. The HA-TCP granules and the PFC are separately packed; they are mixed in the operating room to form a granular, nonsetting paste. Autogenous bone marrow can be added to the mixture to give it more osteoinductive and osteogenic properties. This mixture allows bony ingrowth and rapid vascularization. However, it is nonsetting and can easily become deformed before fibro-osseous tissue ingrowth has occurred. Contour change and volume loss occur because of resorption of both the collagen and the TCP component, amounting to about 40% of the total mixture. The addition of autogenous bone marrow to the mixture decreases the infection rate from 5% to 2.5%.
Polymers
In general, polymers are thought of as plastics, relatively weak solid materials that soften as temperature increases. Polymers are ubiquitous in the human environment and have begun to displace metals and ceramics from traditional applications. Polymers and polymer-based composites represent one of the most exciting areas of modern materials science. They combine moderate strength, low cost, and easy raw material availability with the ability to regulate physical properties by design of composition, internal structural arrangement, and processing. Nowhere has the impact of modern polymeric materials been greater than in medicine, with the resulting wide use of polymeric disposable supplies, dressings, and sutures and the incorporation of polymers into medical devices, surgical instruments, and implants. The most widely used applications are discussed in this chapter.
Polydimethylsiloxane
Polydimethylsiloxane, better known as silicone, has been widely used in craniomaxillofacial surgery. It has proved to be highly compatible with soft tissues. It is easy to shape, is resistant to the physiological environment, and can be produced with a wide range of mechanical properties. Its surface is hydrophobic ( Table 30-1 ). Depending on the number and nature of side chains and crosslinks and the average molecular weight, the resulting materials may be liquid or solid, rubbery or brittle. They may be produced as thermoplastics or as two-part thermoset materials. The latter are used to manufacture a custom mold either before implantation or in situ. The natural host response to the smooth surface of silicone is a fibrous encapsulation. To prevent extrusion of silicone implants, the overlying soft tissues should not be thin, unstable, or under tension ( Fig. 30-4 ). Injections of large amounts of liquid silicone for purposes such as breast augmentation may induce systemic toxicity.
Polymer Name | Chemical Functionality | Water Contact Angle (degrees) |
---|---|---|
Silicone rubber | -(O-Si-CH 3 )- | 111 |
Polyethylene | =CH 2 ′-CH 3 | 95-100 |
Polypropylene | 94 | |
Polytetrafluoroethylene (PTFE) | =CF 2 ′-CF 3 | 104 |
Polymethylmethacrylate (PMMA) | 70-80 | |
Polylactide (PLA) | ||
Titanium (oxide) | 35-45 | |
Stainless steel | 65-75 |
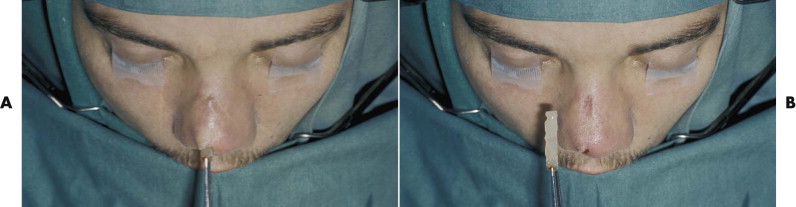
The poor abrasion resistance of silicone rubbers prevents their use in weight-bearing applications. Abrasion of silicone causes a granulomatous inflammatory reaction, both locally and in regional lymph nodes. Because of an ongoing controversy regarding the charge that silicone breast implants can cause connective tissue disease, silicone as a craniomaxillofacial implant material has disappeared from the market almost completely. Silicone blocks have been widely used for craniofacial augmentation purposes, such as malar bones, chin, and nasal dorsum. Care should be taken to prevent migration of the blocks and erosion of the underlying bones as well as perforation of the compromised overlying skin.
Polyethylene
Polyethylene is one of the most commonly used biomaterials. It is the base polymer for other materials such as polypropylene and polytetrafluoroethylene (PTFE). It elicits a minimal tissue reaction, especially when manufactured in a high-density, high-molecular-weight form. In this form, it serves as a reference standard for other materials because of its minimal tissue reaction. Ultra-high-molecular-weight polyethylene (UHMWPE) has proved to date to be the best polymer for load-bearing applications in metal-polymer wear pairs such as artificial joints. Artificial temporomandibular joints have been developed in which UHMWPE serves as an artificial disc placed between a metal fossa part and a ceramic condylar head ( Fig. 30-5 ).
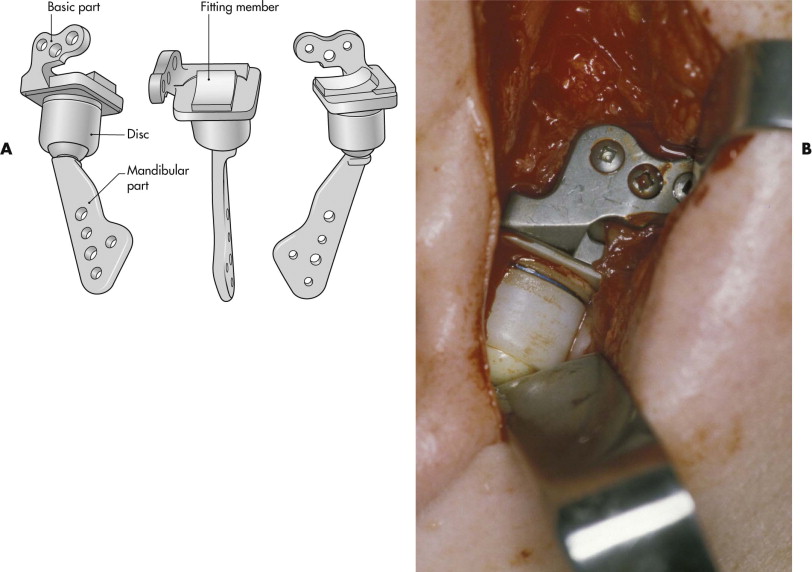
Polyethylene is also available in a porous form. The porous structure of this implant material allows ingrowth of soft tissues 1 week after implantation and bony ingrowth by 3 weeks. The porous polyethylene is easy to shape but difficult to remove after ingrowth. A porous polyethylene reinforced with titanium is available to reconstruct bony defects of the skull after tumor ablation or trauma. Excellent craniofacial symmetry and stability are achievable. Many implant forms are commercially available, including malar, chin, mandible, nasal bone, and orbital floor implants. Flexible porous polyethylene blocks can be ideally suited to repair small to medium-sized cranial defects. Host response to polyethylene is mild, and complications are rare. Porous polyethylene has an advantage over autogenous bone in that it is not susceptible to contour change. It has proved to be a safe and effective bone substitute for contouring of the facial skeleton.
Polymethylmethacrylate
Polymethylmethacrylate (PMMA) has been used as bone cement for 40 years. It is an acceptable space filler that is not resorbed. Its mechanical properties are excellent to serve as a bone replacement in the craniofacial area, and its surface is moderately hydrophobic (see Table 30-1 ).
Methyl methacrylate has two different components, a mixing powder polymer and a liquid monomer. The chemical reaction is exothermic, and the associated toxicity is related to the free monomer component. Cardiovascular collapse and even death have been described in patients undergoing a total hip replacement with freshly mixed methyl methacrylate but not with its use in craniofacial surgery. The free methyl methacrylate monomer can cause asthmatic reactions.
PMMA has been used for reconstruction of skull defects for many years, in cement form and in a presurgically shaped and polymerized solid implant form ( Fig. 30-6 ). Patients with isolated cranioplasty rarely experience infections, but the infection rate increases considerably (to about 23%) in patients undergoing a cranioplasty simultaneously with reconstruction of the orbital wall or nose. If the facial contour requirement is important, especially preshaped PMMA delivers a predictable contour without any resorption. PMMA may be used in adult patients with healthy overlying soft tissues and no infection. It is not an adequate implant material for reconstruction of craniofacial bones in the growing child.
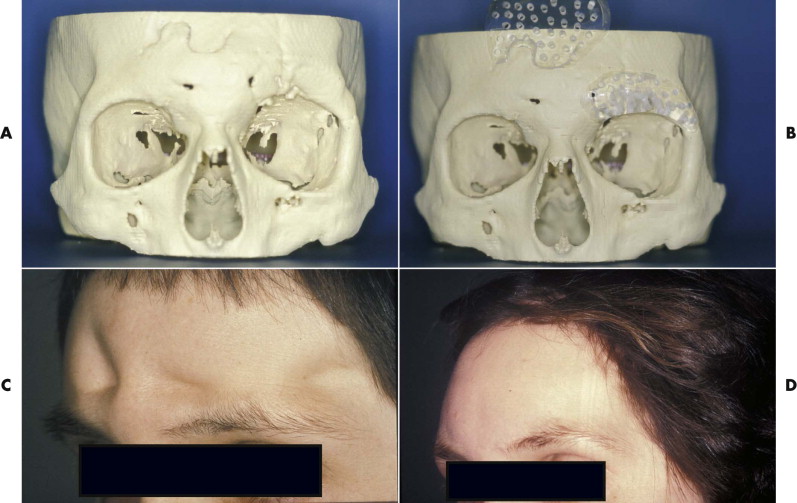
Polytetrafluoroethylene
Polytetrafluoroethylene (PTFE) is used as a base material for various implants. It is frequently used for so-called guided tissue regeneration of dental alveolar bone in dental implantology ( Fig. 30-7 ) and periodontology. PTFE sheets, sold under the trade name Gore-Tex, prevent fibrous tissue ingrowth and allow bony regeneration of a blood clot covered by the Gore-Tex fabric.
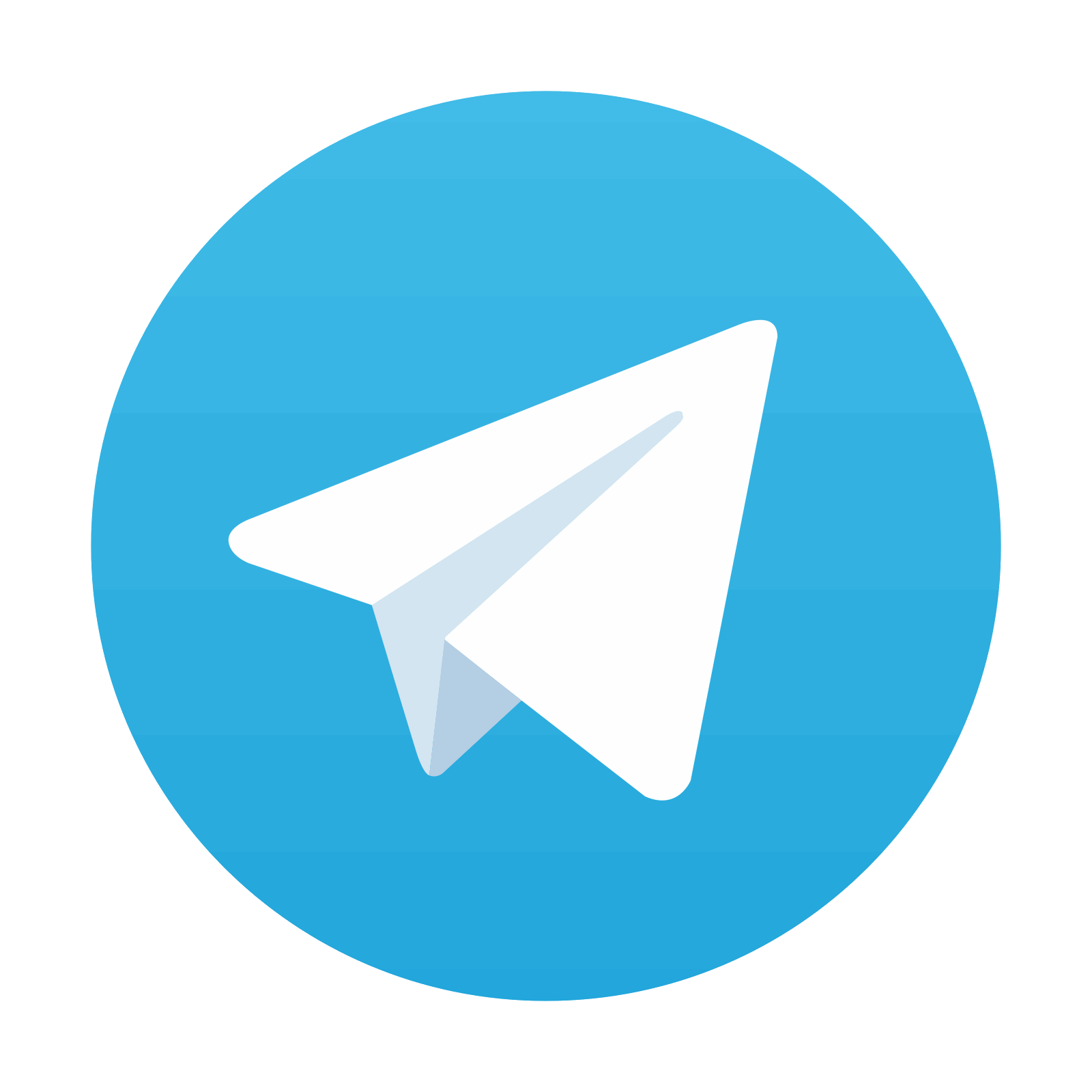
Stay updated, free dental videos. Join our Telegram channel

VIDEdental - Online dental courses
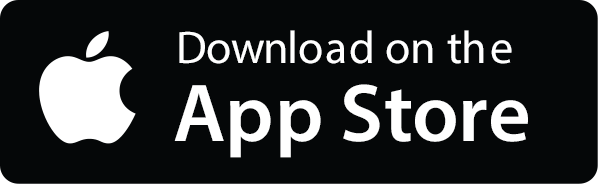
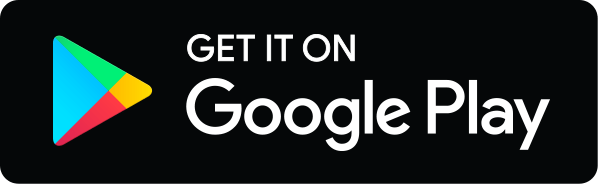