8
Embryonic development—the first few weeks
Chapter contents
8.1 Introduction
Embryology is a fascinating subject and is the foundation of the development, growth, and maturation of all the cells, organs, and tissues of the body. Strictly, embryology is the study of the early processes of development beginning at fertilization and following the processes that turn a single cell into a multicellular organism. It is all about generation of the building blocks required to make a human body. Developmental anatomy is the study of how these building blocks are turned into specific cells, tissues, and organs as well as the general growth of the body. As you will soon appreciate in the following paragraphs, all organs and systems do not develop at the same rate so there is a degree of overlap between embryology and developmental anatomy. For example, the heart and circulatory system must develop and be functioning very early in development to ensure adequate supplies of nutrients to the developing fetal tissues. Teeth, on the other hand, are not going to be used until about six months after birth at the earliest; while the heart is already beating away, each developing tooth is merely a tiny group of cells bearing little resemblance to a fully formed tooth.
Human gestation is considered to take nine months; more accurately, it usually lasts for 38 to 39 weeks from fertilization to birth. Clinically, it is divided into three trimesters of three months each. In this chapter, we will focus on events in the first few weeks. During the first two and a half weeks after fertilization, the very basic building blocks are formed from the single fertilized cell; this is the pre-embryonic period. The embryonic period covers the next five and half weeks during which these basic building blocks develop into the cells, tissues, and organs. As already indicated, some of these may be in a very rudimentary state at the end of the embryonic period. The remaining 30 or so weeks is the fetal period when the tissues and organs of the body grow and develop and the fetus grows considerably. We are not fully mature organisms at birth and have another 20 years a-growing.
This chapter is an account of the development of the embryo from fertilization to about the middle of the embryonic period when all the rudiments and building blocks of significance to dental students are formed. The subsequent development of specific systems—the circulatory, respiratory, and nervous systems—and structures forming the head and neck are dealt with in the corresponding sections describing their anatomy.
8.1.1 The importance of understanding development
Understanding the very early stages of development provides a general knowledge of how you developed and will equip you with a vocabulary to understand the terms and processes that takes place during the development of those systems relevant to dental practice.
Knowledge of the way in which different structures develop often clarifies the mature anatomy, especially the overall patterns that govern the way things are arranged in the body.
Embryology can provide a logical explanation of anatomy where there appears to be none. The embryology or developmental anatomy of given structures can often explain apparent anomalies or illogicalities. Why is the diaphragm innervated by nerves that originate in the neck? Why do nerves innervating the larynx go all the way down into the thorax and come all the way back up into the neck when it would be much simpler to direct the nerves straight across the neck to the larynx? The mysteries of the strange courses of the phrenic nerves and recurrent laryngeal nerves will be explained in context in Chapters 10 and 13 respectively.
As you read the descriptions in this and other chapters covering development, you will see that embryology and development is an awe-inspiring process. You will wonder how anybody ever arrives in this world with 20 tiny fingers and 20 tiny toes on those little limbs, those eyes, and those rosy cheeks wherewith life begins. Alas, many individuals do not. Something has gone wrong during their embryological development to cause the absence or deformity of tissues or organs. Such developmental abnormalities may be life-threatening at worst; even at best, many will cause functional and/or cosmetic defects that render life difficult.
As practising dentists, you will see abnormalities of the face, jaws, and teeth which you may be able to correct yourself or refer to one of your colleagues specializing in their treatment. You will be expected to understand how such conditions arose (if known) as many patients will be at least curious and may be very concerned about the consequences and possible risks of inheritance by their children. You will also come across records of abnormalities in other systems during the taking of patients’ medical histories. Some conditions will affect the way in which you treat your patients and must be taken into account during your treatment planning.
The most dangerous period for developmental abnormalities to arise is the pre-embryonic period. The embryonic period is only marginally less dangerous and still fraught with hazard. The fetus is not entirely safe until delivered at the end of gestation. The risks should be borne in mind when treating pregnant patients. The use of many drugs and chemicals is very strongly contraindicated during pregnancy as they can cause developmental defects.
8.2 Fundamental processes underlying development
Embryology is essentially the development of all the different types of cells of the body from a single cell. It follows, therefore, that cell division or mitosis is a major process in development. However, cell division also needs to be limited in certain areas at certain stages of development, otherwise each cell type would be equally as numerous; your teeth would be the same size as your brain. The rate of mitosis in one tissue also needs to be coordinated with cell division in other tissues so that structures develop in harmony. Cell death is another important process in embryological development. It may seem strange to introduce death into a chapter about the beginning of life; cell death during development is not random but is programmed cell death or apoptosis in which cells are programmed to commit suicide at specific times and places. Two examples of the role played by apoptosis are the formation of hollow tubes from a solid cord of cells or the removal of tissues that would restrict the movement and development of other tissues.
Movement of cells is another absolutely vital process in development and takes place at several levels. Cells have to change shape to bring about a specific configuration so that their functions are maximally efficient. Coordinated changes of shape in all cells across a sheet of tissue can alter the shape of the tissue from a flat sheet into a curve or even a tube. If cells simply accumulated as a result of multiple cell divisions, we would end up as a large ball of cells without any recognizable form. Cells have to move as individuals or populations along defined pathways to produce definitive shapes and structures; this is cell migration. Often, one population of cells may be required to migrate while another remains static.
Ultimately, millions of cells are going to arise from one single fertilized ovum. It may be surprising that only about 200 different basic cell types, recognizable by their different shapes and functions, make up the human body although some cell types are further subdivided by function or chemical differences that cannot be seen directly with microscopes. Tissues are aggregates of cells, usually comprising several different cell types. Cells must, therefore, use the repertoires outlined in the previous paragraphs to divide and migrate to the correct locations at the right time. Ultimately, tissues are combined to form specific organs and systems by further division, migration, and combination.
You will already be aware that most cells in the body have a specific function; when cells are fully differentiated to carry out their specific function, most cannot change their function at all and certainly not radically. Fat cells cannot be used as neurons and muscle cells cannot be converted into bone. What creates this difference between cells? All cells within the body contain the same genetic information, with the exception of red blood cells that lack nuclei and the gametes (ova and spermatozoa) that only contain half the genetic information. So why is a neuron different from an adipocyte (fat cell)? It is all due to gene expression—the specific genes that are expressed within each cell type that make each one unique from another cell by determining the types of proteins that a particular cell will produce. The proteins, in turn, determine the shape and function of cells, how they attach to each other and other cell types, what type of receptors they carry and, therefore, what messengers they can respond to, and many other functions. Many genes are active in all cells to direct basic cell functions such as energy production and repair; these are housekeeping genes. During development, many genes direct the formation of proteins that switch on or switch off other genes; these are gene-regulatory proteins. Essentially, as development proceeds, the majority of genes within a cell are switched off so that only a very limited amount of the total genome in each cell is operating to produce the specific function required. The orderly switching on and off of genes during development ensures that cell division, death, migration, and differentiation take place in the correct sequence, at the correct time, and in the correct place to produce the complex functioning organism that is the human body.
Another important concept to understand is that once cells embark down a pathway of differentiation, there is usually no going back to an earlier less differentiated stage. Cells, therefore, become much more restricted in what they can become as development proceeds until eventually they are fully differentiated into a specific cell type. There are cells that retain some of their embryonic characteristics and can differentiate into several cell lines. These cells are useful for generating new cells to replace cells lost by normal wear and tear, trauma, or disease; they are known as stem cells (see Box 8.3).
As cells of the embryo differentiate, they initially form specific germ layers from which certain cell types, but not others, can differentiate. Four germ layers develop during the pre-embryonic and early embryonic period, each giving rise to a specific set of cell types. Cells originating from two or more different germ layers are usually required to form tissues and organs. The development of the right number of cells of each kind, their aggregation, and differentiation must be coordinated precisely. Signalling between different germ layers is described as an interaction. Often one germ layer determines what another germ layer does by causing a change in shape, movement, or function. This process is induction; the determinant germ layer produces signalling molecules that act on another recipient germ layer. The inducing cells may not show any obvious change as they act on other cells, but may then exhibit marked changes in their own behaviour. This change is often induced by the cells they originally induced and when germ layers take it in turns to induce each other, this is a reciprocal interaction.
Now the fundamental processes involved in embryological development and growth have been outlined, we will apply them to examine how development takes place in the first few weeks of life.
A whole raft of arcane terminology is used in many textbooks to describe embryos and the nervous system. As you will see, the front and back and head and ‘tail’ end of an embryo can be distinguished from a very early stage; everything can, therefore, be referred to the anatomical position (Section 1.3.1), obviating the need for obscure terminology.
8.3 The first three weeks of development
8.3.1 The first week
The major events in the first week of life are fertilization followed by rapid cell division to produce more cells. Towards the end of the first week, two distinct groups of cells can be recognized, those that will go on to form the embryo and those that are involved in implanting the embryo in the uterine wall and forming the placenta.
The sequence of events in the first week of life is shown diagrammatically in Figure 8.1. This diagram represents the ovarian (Fallopian) tube connecting to the uterus in the top right hand corner; the ovary from which the ova (eggs) are released would be off the bottom left of the picture. Events begin at the bottom left hand corner and follow in sequence to the top left and across and down to the bottom right.
Fig. 8.1 Events of the first week: fertilization and cleavage.
Fertilization usually occurs between 12–24 hours after ovulation, the release of an ovum (egg) from the ovary (Figure 8.1A). Fertilization occurs in the distal third of the oviduct when an ovum is fertilized by a spermatozoon. The gametes (ovum and spermatozoon) each contain only 23 chromosomes—the haploid number. When the egg is fertilized as shown in Figure 8.1B, the chromosomes from each gamete meet in the nucleus of the zygote, producing 46 chromosomes (the diploid number), 22 pairs of autosomal chromosomes, and one pair of sex chromosomes. The sex of the zygote depends on whether the sex chromosome of the fertilizing sperm is an X or a Y; the sex chromosome carried on the ovum is always an X. At fertilization, the zygote commences a series of mitotic cell divisions (cleavage divisions). These divisions will finally result in the formation of a new individual comprising millions of cells, all derived from one single cell. The zygote will give rise to all other cells in the body. Put another way, it has the potential to produce all cells of the body and is, therefore, totipotential.
As shown in Figure 8.1C to F, the original single-celled zygote divides into two, four, eight, and 16 cells. Each cell is known as a blastomere. The time after fertilization at which each stage is reached is shown in Figure 8.1 the two-cell stage is attained about 30 hours post-fertilization, the 4-cell stage after 40–50 hours, and the 12–16 cell stage at about three days. By the time the 16-cell stage has been reached, the ball of blastomeres, now called a morula, has reached the uterus. If you have not noticed already in Figure 8.1, the 16-cell stage morula (F) is the same size as the original ovum (A). This is a depiction of the reality; the 16 blastomeres occupy no more space than did the original egg. As each cleavage division occurs, each new nucleus takes its share of the original cytoplasm of the ovum. Only new nuclei and cell membranes are added at each division. Note in Figure 8.1A to E that the dividing ovum is surrounded by the zona pellucida; it prevents the entry of subsequent sperm once one has penetrated the ovum and fertilized it.
If the blastomeres making up the 16-cell morula were separated from each other at this stage, each one has the potential to form a separate embryo; each blastomere is still totipotential. These totipotential cells are known more generally as embryonic stem cells and are the subject of intense ethical debate about their use in medical research.
Further examination of the final steps of the journey of the fertilized ovum to the uterus in Figure 8.1G shows that the morula continues to divide but the cells now begin to segregate. Those forming the inner cell mass in the centre are differentiated from those on the surface of the ball. The inner cell mass forms the embryo whereas the outer cells will form the trophoblast from which the placenta will develop. The blastocoel shown in Figure 8.1H is created as uterine fluid passes between the outer cells; the inner cell mass gravitates to one end of this cavity. The resulting structure is now known as a blastocyst which contains 100–150 cells. The zona pellucida has disappeared.
Towards the end of the first week, at about 5½–6 days after fertilization, the blastocyst becomes attached to the uterine wall as shown in the last picture in the sequence of Figure 8.1. Attachment of the blastocyst is the first event in the process known as implantation during which the placenta forms. The placenta is the organ where exchange of nutrients and waste products takes place between the maternal and fetal circulation. Figure 8.2A shows the effects of proteolytic secretions from the trophoblast; they erode the lining of the uterus and cells from the outer cell mass invade the space created. The blastocyst thus becomes implanted (embedded) in the uterine wall.
As you can imagine, even in this apparently simple sequence of mitoses during the first week, there is a lot of scope for things to go wrong. These are described in Box 8.1.
Box 8.1 Early embryonic abnormalities
Accidents of fertilization are common but their causes are poorly understood. It has been estimated that between one and two out of three zygotes develop an abnormal number of chromosomes or major mutations within the normal number of chromosomes as early cleavage divisions take place. Most of these abnormal embryos spontaneously abort in the very early stages of pregnancy, often without the mother being aware that an abortion has occurred, the loss of the embryo appearing as a late period. Because so many abnormal embryos spontaneously abort, only some six per 100,000 individual live births have detectable chromosome abnormalities. Probably the best />
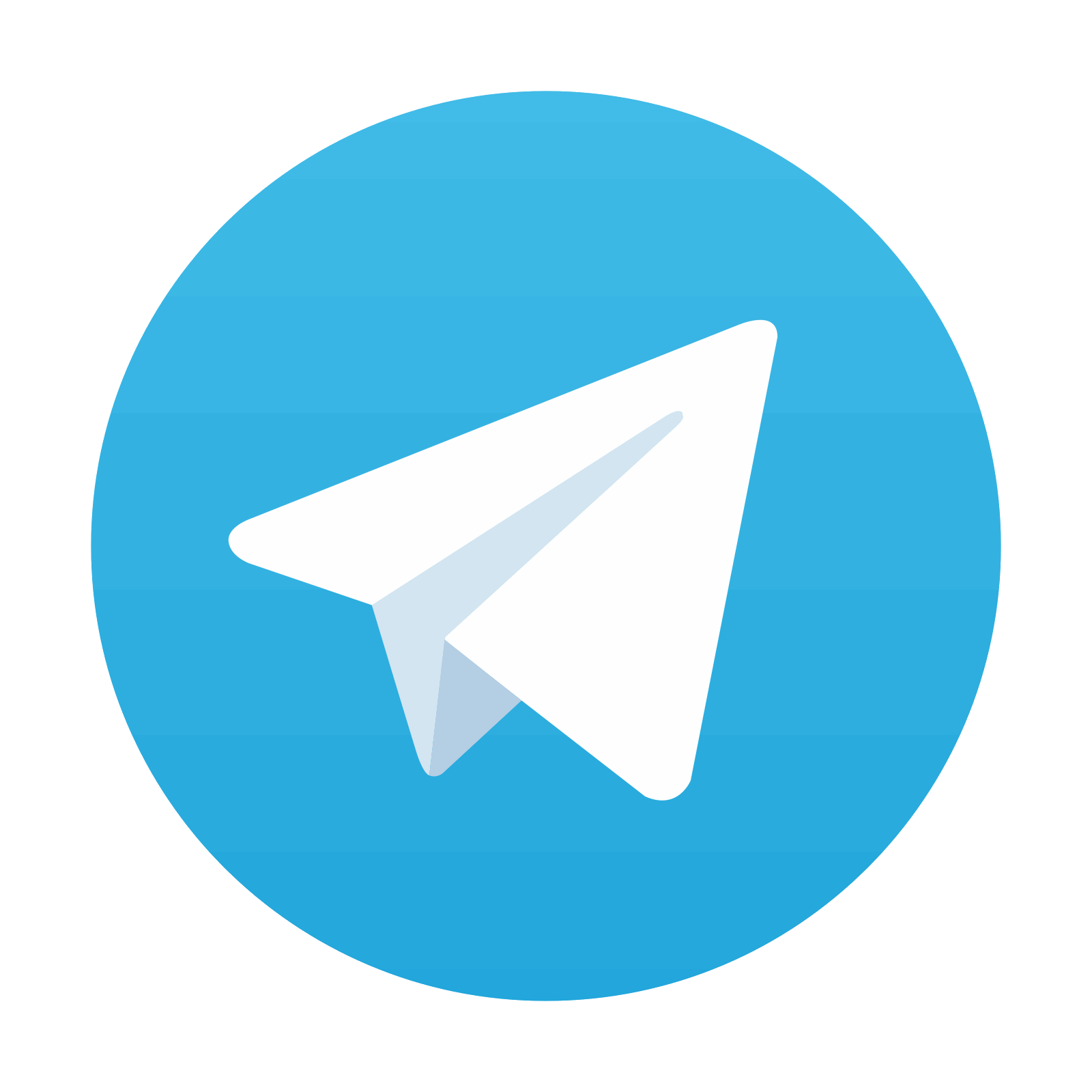
Stay updated, free dental videos. Join our Telegram channel

VIDEdental - Online dental courses
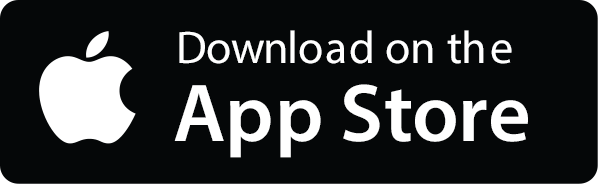
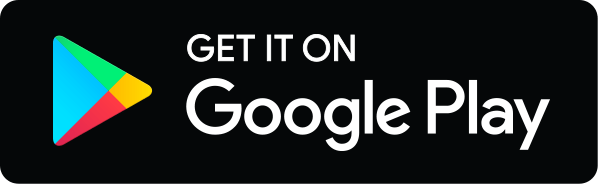