18
The cranial nerves
Chapter contents
18.5 The oculomotor (CN III), trochlear (CN IV), and abducens nerves (CN VI) |
18.8 The vestibulocochlear nerves (CN VIII), auditory, and vestibular pathways |
18.1 Introduction
The cranial nerves are the most important neural structures relevant to dental students and practitioners. The cranial nerves are the nerve supply to all the structures in the head and neck and underpin of the anatomy and function of these regions—the head and neck will not work without them. In a wider context, correct functioning of the cranial nerves is a very good indicator of the health or otherwise of the CNS; it may be necessary to test the function of some, or even all, of the cranial nerves at times to assess neural function. In addition, many of the cranial nerves may be involved in various diseases of the head and neck.
As outlined in Chapter 3, 12 pairs of cranial nerves arising from the brain form one major component of the peripheral nervous system, the 31 pairs of spinal nerves forming the other. Each pair of cranial nerves has a name and number. Conventionally, they are numbered using the Roman numerals I to XII. The nerves are numbered from one to 12, according to their origin from the brain; nerves with the lowest numbers arise from the most anterior aspect of the brain (the forebrain) whereas those with highest numbers arise from the lowest part (the medulla).
Several aspects of any nerve anywhere in the body are required to describe its anatomy and function in complete detail:
• Its origins and terminations in the CNS;
• Its neuronal components—are they motor, sensory, or autonomic?
• Its course to and from its target tissues;
• Its distribution to specific areas and structures through specific branches;
• Its overall functions and specific functions of its component parts.
In addition, if the clinical significance is going to be appreciated, we will also need to consider:
• The effects of damage or disease on the nerve;
• Its important relationships to other structures;
• How to test whether the nerve is functioning correctly.
Given that there are 12 pairs of nerves, does a competent dentist need to know everything in the two lists about every cranial nerve? The answer, you will be relieved to hear, is ‘no’. To paraphrase George Orwell—‘all cranial nerves are important, but some are more important than others’. You should be able to list, for all 12 pairs of cranial nerves:
• Their component types of neurons;
• Their function;
• How to test their function;
• And outline their distribution.
More detail than this bald outline is required in some of the different headings for some nerves (III, IX, X, and XII), but precise detail is required for a few (V and VII). Why have certain nerves been emphasized? The nerves that require detailed knowledge are those nerves that dentists will be working on everyday, either by anaesthetizing them or trying to avoid damage to them during surgical procedures.
18.2 General anatomy of the cranial nerves
Cranial nerves are similar to spinal nerves in many respects, but differ significantly in others. As we have seen in Chapters 3 and 15, the general plan of all spinal nerves is identical; they are mixed nerves containing motor and sensory processes. As described in Chapter 17, some spinal nerves originating from the thoracic, lumbar, and sacral regions of the spinal cord also contain autonomic neurons. Cranial nerves are not all identical in composition; some are purely motor nerves, some are mixed somatic sensory and motor nerves, and others contain autonomic components. Some purely sensory cranial nerves are specialized to serve specific sensations such as olfaction, vision and hearing, and balance (the special senses). In addition, some cranial nerves contain neuronal types that are not found in spinal nerves such as those conveying the sensation of taste.
Despite these differences, some similarities can still be seen. The cell bodies of somatic sensory components in cranial nerves are found in ganglia lying outside the CNS, analogous to the dorsal root ganglia of spinal nerves.
It should also be pointed out that of the 12 pairs of cranial nerves, only the third to twelfth nerves are true peripheral nerves, the distinction being that their processes are ensheathed by Schwann cells as opposed to glial cells. The first two pairs of cranial nerves develop as outgrowths from the brain and carry their covering glial cells and meninges with them. They are, in fact, components of the CNS rather than the PNS, but are still considered as cranial nerves.
18.2.1 Neuronal types in cranial nerves
Table 18.1 lists the types of neurons found in each of the cranial nerves. Do not just learn the table. Read the following explanation to understand the distinctions between the different neuronal types, then use the table for quick reference and a reminder of the salient points.
The first point to note is that cranial nerves I (olfactory), II (optic), and VIII (vestibulocochlear) do not fall within the general plan in Table 18.1 because they are nerves conveying the special senses of smell, vision and hearing, and balance respectively.
The muscles of the head and neck are derived embryologically from mesoderm just like muscle tissue anywhere else in the body (see Chapter 8). Most of these muscles are voluntary muscle, therefore, we should expect them to be innervated by somatic motor nerves. This is clearly the case for muscles supplied by cranial nerves III (oculomotor), IV (trochlear), VI (abducens), and XII (hypoglossal). The third, fourth, and sixth cranial nerves supply the extraocular muscles that move the eyes within the orbital cavities. These muscles are derived from preotic somites in the anterior part of the developing head. The hypoglossal nerves supply the muscles of the tongue derived from occipital somites that develop near the base of the skull formed by the occipital bone (see Section 21.8). Cranial nerve XI (spinal accessory) supplies two muscles in the neck whose embryonic origins are uncertain, but these nerves certainly comprise somatic motor neurons.
Table 18.1 Neuronal types in the third to twelfth cranial nerves
During development of the face and neck, a series of paired sausage-like structures grow out from around the hindbrain as ectomesenchymal cells migrate as the neural tube closes (see Section 8.3.4). These structures are the pharyngeal (or branchial) arches (see Section 20.1). Initially, there are six pairs of arches, but the lower three pairs rapidly coalesce to form complexes known as the fourth arches. Certain muscle groups in the head and neck derive from mesoderm within each arch, each group being derived from a specific arch. Each pair of arches is innervated by a specific pair of cranial nerves and any structures derived from those arches are innervated by those cranial nerves. Muscles from arch 1 is supplied by cranial nerve V (trigeminal), arch 2 by cranial nerve VII (facial), arch 3 by cranial nerve IX (glossopharyngeal), and arch 4 by cranial nerve X (vagus). These cranial nerves also contain somatic motor nerves, sometimes referred to as branchiomotor nerves (see Box 18.1).
As already outlined in the introduction to this chapter and Chapter 16, four pairs of cranial nerves (III, VII, IX, and X) contain autonomic parasympathetic motor neurons, often called secretomotor neurons.
Some cranial nerves deal specifically with special senses as we have seen. However, the other cranial convey somatic sensory innervation from the skin of the head and mucosa lining the respiratory and gastrointestinal tracts as they pass through the head and neck. Cranial nerves V, IX, and X have major somatic sensory components.
As we have seen in Chapter 17, visceral sensation is somewhat different from somatic sensation; much of the sensation from the viscera is unconscious and functions to control the function of internal organ by monitoring their stretch or distension, for example. Two pairs of cranial nerves (IX and X) contain general visceral sensory nerves, controlling cardiovascular, respiratory, and gastrointestinal function.
Box 18.1 Mistaken origins of pharyngeal arch muscles
When anatomists and embryologists were first working out the developmental relationships between the muscles of the pharyngeal arches and the cranial nerves, they believed that muscles that developed in the pharyngeal arches were derived from a different source of mesoderm than that which forms voluntary muscles elsewhere in the body. They thought that the muscles derived from mesoderm that also produced some of the internal organs so they distinguished the nerves innervating these muscles as branchiomotor (or special visceral motor) nerves. We now know that these pioneer anatomists were wrong, but the name is still used in many textbooks. You may think it is an arbitrary distinction, but you will see why the distinction is important when we examine the cranial nerve nuclei in the next section. Under this classification, a given cranial nerve can contain either somatic motor or branchiomotor components, but not both.
The final neuronal type found in cranial nerves is designated as special visceral sensory; this is just a special term for neurons that convey the sensation of taste. Cranial nerves VII, IX, and X contain taste neurons.
18.2.2 Cranial nerve nuclei
You should now appreciate from the description above and Table 18.1 that the cranial nerves differ quite markedly in structure and function. It follows from this that the terminations of each nerve in the brain will differ, depending on their functions. As described in Chapter 15, the spinal cord has the same overall structure along its entire length. The spinal cord has grey matter dorsal horns throughout its length that receive incoming somatic sensory processes that all spinal nerves contain. Likewise, the ventral horns extend the length of the spinal cord spinal nerves because all spinal nerves contain somatic motor components that originate from cell bodies in the ventral horns. Some areas of the spinal cord also have intermediolateral horns where autonomic axons arise.
In contrast to spinal nerves, cranial nerves only need areas in the CNS for the synaptic termination of the specific types of neurons they carry so that appropriate connections can be made. To put it another way, if a cranial nerve lacks a particular component, then there is no need for a connecting area. For example, a cranial nerve that has purely motor functions has no need for the equivalent of dorsal horns for reception of sensation.
The dorsal and ventral horns of the spinal cord develop from structures called the alar and basal laminae as shown in Figure 18.1A. The internal structure of the brainstem from which the third to twelfth cranial nerves arise is actually analogous to the spinal cord, but the structures are displaced. As shown in Figure 18.1B, the grey matter is split vertically and pushed laterally by the presence of the fourth ventricle. Sensory, motor, and autonomic areas of grey matter still maintain their relationships relative to each other, but sensory areas in the brainstem lie laterally to the motor areas; there is a corresponding intermediate area that serves general visceral functions.
Fig. 18.1 A) Development and structure of the spinal cord. B) Development and structure of brainstem.
The grey matter in the brainstem is, therefore, discontinuous because there are only areas specific to each different function of each cranial nerve. These discrete areas of grey matter are the cranial nerve nuclei. If you imagine a series of columns extending into the brainstem from the grey horns of the spinal cord, it emphasizes the similarity between the arrangement of the grey matter of the spinal cord and brainstem. Each column will then be found to contain nuclei with functions similar to those of the grey horn with which they align. One column can be imagined as an upward extension of the dorsal horn, containing nuclei associated with somatic sensory neurons like the dorsal horn itself. A column in line with the ventral horn contains nuclei where the cell bodies of somatic motor neurons are located as in the ventral horn. Six columns of cranial nerve nuclei are shown in Figures 18.1 and 18.2:
• Column 1 (most laterally) contains somatic sensory nuclei;
• Column 2 contains taste (special visceral sensory) nuclei;
• Column 3 contains general visceral sensory nuclei;
• Column 4 contains parasympathetic (general visceral motor) nuclei;
• Column 5 contains (branchiomotor) motor nuclei for nerves that innervate muscles derived from the pharyngeal arches;
• Column 6 (nearest the midline) contains somatic motor nuclei to muscles derived from somites.
Columns 1 and 2 are sensory, 3 and 4 autonomic, and 5 and 6 sensory. However, as Figure 18.2 shows, these nuclei occupy only parts of their respective columns with long stretches where no nuclei are present.
The position of cranial nerve nuclei
The position and number of nuclei for each cranial nerve (excluding those serving the special senses) can be worked out using a few basic rules. As we all know, rules are made to be broken as we will see as each nerve is described. There are exceptions to these general rules and some cranial nerves make use of nuclei of other nerves for similar functions. The rules for working out the cranial nerve nuclei for nerves III to VII and IX to XII are as follows:
1. There is one nucleus for each function of the cranial nerve on each side;
2. Sensory nuclei lie lateral to motor nuclei with autonomic nuclei in between (see Figure 18.1B);
3. The nuclei are at the level at which the cranial nerves attach to the brainstem.
Using Table 18.1 which outlines the function of each component of the cranial nerves as a basis and using information about the level of origin of each pair of cranial nerves described in Chapter 15 and illustrated in Figure 15.10, we can build up a scheme for the cranial nerve nuclei using the three rules.
We will use the hypoglossal nerve as an example. From Table 18.1, we can see that this is a pure somatic motor nerve so rule 1 tells us there should be one motor nucleus on each side of the brainstem. Rule 2 tells us that each nucleus should lie close to the midline. The hypoglossal nerves are attached to the lower medulla just above the spinal cord so the application of rule 3 tells us the nuclei should be low down in the medulla. Look at Figure 18.2 that shows the actual cranial nerve nuclei in a schematic diagram and confirm that these predictions are correct; the hypoglossal nuclei are indeed adjacent to the midline low in the medulla. If we take another example of the trigeminal nerve (CN V), there should be two nuclei in each half of the brainstem, a somatic sensory and a motor nucleus, according to rule 1 and the information in Table 18.1. The sensory nucleus should lie laterally to the motor nucleus (rule 2) and they should be at the level of the pons (rule 3). Now look again at Figure 18.2. There are two nuclei and the sensory nucleus is lateral to the motor one. Clearly, the motor nucleus is in the pons; note that it is slightly lateral to the hypoglossal nucleus, reflecting the different embryological origins of the two nerves. Look at the sensory column. What is going on with the sensory nucleus shown in Figure 18.2? It extends way beyond the pons where we would expect it to be located down into the spinal cord and up through to the midbrain. The reason for the vast expansion of the trigeminal sensory nucleus is that it receives sensation from areas of the head that are very sensitive and highly discriminative such as the tongue, lips, and eyelids. If discrimination is to be preserved, then incoming nerves cannot converge and must retain their individuality. They simply will not pack into the available space in the predicted location in the pons and so expand above and below that level.
If Figure 18.2 is examined against the information in Table 18.1, several other anomalies will be apparent. Some are anatomical whereas others are functional. Anatomically, the parasympathetic nucleus of the oculomotor nerve (the Edinger–Westphal nucleus) is displaced more medially than it should be. Similarly, the motor nucleus of the vagus is more laterally than it should be; this nucleus is called the nucleus ambiguus because its position is ambiguous. The ninth (glossopharyngeal) and tenth (vagus) nerves should have somatic sensory nuclei in the medulla, but they are clearly missing from Figure 18.2. These nerves use another nucleus, in this case, the sensory nucleus of the trigeminal nerve. The rules also predict separate nuclei for the termination of taste neurons travelling in the facial, glossopharyngeal, and vagus nerves. However, these all converge into a single area, the upper part of the nucleus of the tractus solitarius. General visceral sensory neurons, principally in the vagus, but also in the glossopharyngeal nerve, converge to terminate in the lower part of the nucleus of the tractus solitarius. As can be seen in Figure 18.2, this part inclines medially to lie in the general visceral sensory column.
In the following sections of this chapter, an overview of the individual cranial nerves is presented. The main emphasis is on the types of neurons in the cranial nerves, the central connections between cranial nerve nuclei and other parts of the brain, their functions, and clinical tests. Only an outline of the course and distribution of each of the cranial nerves will be given here. Where further detail is required, it will be described in Section 4 on the regional anatomy of the head and neck.
Fig. 18.2 The arrangement of the cranial nerve nuclei in a dorsal view of the brainstem. Motor nuclei are shown on the right and sensory nuclei on the left for clarity.
18.3 The olfactory nerves (CN I)
The olfactory nerves convey the sense of smell from the nose to the olfactory areas in the temporal lobes (see Section 15.4.2). The olfactory nerves are usually clearly visible, running along the underside of each frontal lobe as seen in Figure 15.17C. As described in Chapter 15, each nerve ends in the olfactory bulb, a bulge created by the presence of cell bodies positioned in the anterior cranial fossa above the nasal cavities. Olfactory mucosa only covers a small area in the roof of each nasal cavity and comprises specialized neurons. Their axons pass through perforations, forming the cribriform plate of the ethmoid bone to synapse in the olfactory bulb. The axons of the olfactory bulb cells form the olfactory nerves that run back to enter the temporal lobes and terminate the olfactory cortex. The connections of the olfactory cortex with other parts of the brain have been described in Chapter 15.
18.3.1 Clinical testing of the olfactory nerves
If required, the function of the olfactory nerves are tested by holding a strong smelling substance, such as smelling salts, under each nostril in turn while occluding the other nostril.
18.4 The optic nerves (CN II)
The optic nerves are the first components in a series of neurons that form the visual pathways, conveying vision from the retina of the eyeballs to the visual cortex in the occipital lobes.
Cells called rods and cones are the photoreceptors in the retina in each eyeball. The optic nerves are the axons of cells that connect indirectly to the rods and cones. These axons emerge as the optic nerve from the posterior aspect of each eyeball and have a long course inside the bony orbits before passing through the optic canals into the middle cranial fossa. As Figure 15.24 shows, the optic nerves can be seen very clearly on the underside of the brain medial to the internal carotid arteries. This is not surprising as the optic nerves are the largest of the cranial nerves, each nerve containing about a million axons. Figure 18.3 indicates that they have quite short intracranial course before the two nerves merge with each other to form the optic chiasma.
18.4.1 The visual pathways
The rest of the visual pathway is illustrated in Figure 18.3 which should be followed as you read this description. As the two optic nerves converge at the optic chiasma, the axons from the nasal half of each retina decussate whereas those from the temporal half of the retina do not cross. The axons passing through the optic chiasma diverge again to form the optic tracts, the continuations of the optic pathway. Each optic tract contains temporal axons from the eyeball of its own side and nasal axons from the opposite eyeball. Most of the axons in the optic tract terminate in the lateral geniculate nucleus of the thalamus. Post-synaptic axons form the optic radiation on each side which passes through the posterior limb of the internal capsule to the visual cortex in the upper and lower lips of the calcarine sulcus of the occipital lobe.
As Figure 18.3 shows, some of the axons in the optic tract have collateral axons that branch off before the lateral geniculate nucleus to end in the pretectal area and superior colliculus of the midbrain. These parts of the midbrain also receive collateral axons from the optic radiation and neurons that pass back from the visual cortex. These pathways to the midbrain are involved in reflex responses to light, including the pupillary light reflex (constriction of the pupil in response to bright light) and the accommodation reflex (ocular convergence, pupillary constriction, and change in the curvature of the lens when viewing close objects) as well as reflex movements of the eyes and neck to track objects moving across the visual fields.
It is important to understand that the retinal images of the visual fields are inverted and reversed right to left by the optics of the cornea and lens of the eye. In clinical practice, visual fields (or fields of vision) are referred to rather than the parts of the retina. As Figure 18.3 demonstrates, information from the temporal field of vision actually ends up on the nasal part of the retina. Due to the decussation of nasal axons and the continuation of temporal axons, each optic tract and radiation contains information from both eyes, which is important for stereoscopic vision. The representation of each eye in the optic tract and radiation also explains why light reflexes are consensual and will occur in both eyes at the same time—a bright stimulus delivered to one eye will cause pupillary constriction in both eyes, for example.
Fig. 18.3 The visual pathways.
Box 18.2 The effects of damage and disease on the visual pathways
It should be apparent from the above account that lesions at different parts of the optic pathway will produce different visual defects. A lesion in one eye or the optic nerve may lead to blindness in that eye. A common cause of damage to the optic nerve is compression due to raised intraocular pressure due to glaucoma. Damage to the optic chiasma, usually due to the pressure from an enlarged pituitary gland caused by tumourous growth, produces a condition known as bitemporal hemianopia (= half blindness in both eyes in the temporal fields of vision). The pressure on the chiasma will tend to interrupt conduction in the decussating axons from the temporal visual field while the nasal visual fields are unaffected. Consequently, the patient will lose sight in the left and right temporal fields of vision.
Depending on the exact level of the lesion, damage to the optic tract, radiation, or visual cortex of one side will produce loss of different parts of the visual fields of both eyes in quite complex manners, which are beyond the scope of dental practice.
Clinical testing of the optic nerves and visual pathways
Tests of visual acuity and visual loss are the specialist realm of optometrists. However, most dental students and practitioners will be familiar with many of the routine tests employed as they will have had their own eyes tested at some stage in their career. If you have not had your eyes tested yet, better sooner than later.
18.5 The oculomotor (CN III), trochlear (CN IV), and abducens nerves (CN VI)
The third (oculomotor), fourth (trochlear), and sixth (abducens) cranial nerves are treated together because as shown in Table 18.1, they are all somatic motor nerves supplying extraocular muscles controlling eye movement. In addition, the table indicates that the oculomotor nerve also contains parasympathetic neurons that control the pupillary diameter and lens curvature within the eye; these movements are reflex responses to light conditions and focusing requirements. The meaning of oculomotor should be fairly obvious—motor to the ocular apparatus (eye). However, the names given to the fourth and sixth cranial nerves are much less obvious; all will become clear as these nerves are described.
18.5.1 The extraocular muscles
Before the functions of the nerves are described, a brief explanation of the extraocular muscles is required, although they will be described more fully in Section 30.4.1. There are six extraocular muscles in each orbit and their dispositions are shown in Figure 18.4. The four rectus muscles (= straight) turn the eyeball (globe) in the same direction as their names imply:
• The superior rectus turns the eye upwards;
• The inferior rectus turns the eye downwards;
• The lateral rectus turns the eye laterally;
• The medial rectus turns the eye medially.
Note that the superior and inferior recti also exert a medial pull on the globe because the longitudinal axis of the orbits is at an angle to the midline whereas the eyes look straight ahead as shown in Figure 30.7.
The superior and inferior oblique muscles have more complicated and less obvious actions determined by their position of attachment to the globe. Both oblique muscles turn the eye laterally, but the superior oblique muscle turns the eye downwards, and the inferior rectus turns it upwards; these two muscles have the opposite effect to their name on vertical eye movement.
Fig. 18.4 The extraocular muscles.
18.5.2 The oculomotor nerves
The oculomotor nerves supply the superior, inferior and medial rectus muscles, and the inferior oblique muscle. They also carry the major motor nerve supply to the levator palpebrae superioris muscle elevating the upper eyelid.
The somatic motor neurons to these extraocular muscles originate in the oculomotor nucleus which is situated in the midbrain at the level of the superior colliculus as shown in Figure 18.2. The motor units in the extraocular muscles are very small, allowing very precise control of eye movements; each axon supplies about six muscle fibres. As can be seen in Figure 18.5, the oculomotor nerves are usually visible, exiting the under surface of the midbrain. They can usually be seen between the posterior cerebral and superior cerebellar arteries as shown in Figure 15.24 on an intact brain; a short stump may be visible, entering the cavernous sinus when the brain has been removed from the skull.
The oculomotor nucleus receives inputs from the cerebral cortex for the voluntary control of eye movements. It is easy to forget that in any direction of gaze where there is a horizontal component, each eye is moving in an opposite direction. As you are reading this text from left to right, your right eye is moving laterally, but your left eye is moving medially. If you were watching a game of tennis, you would only be able to follow the ball so far by eye movement alone; you would eventually have to turn your head in the direction of travel and may even have to turn your body. Of course, when you have been lucky enough to obtain those tickets to the final, you will not be thinking of eye, head, and body movement; they will be happening subconsciously so you do not miss the action. Visual inputs via the superior colliculus and pretectal area and from the vestibular nuclei (see Section 18.8.2) give information about movement in the visual fields and head position, respectively. Outputs allow eye, head, and body movement to be coordinated through connections to nuclei controlling the other extraocular muscles, including those of the other side, and the relevant segments of the ventral horns of the spinal cord.
The oculomotor nerves also carry parasympathetic preganglionic axons to the sphincter pupillae and ciliary muscle of the eyeball. These nerves originate from the Edinger–Westphal nucleus close to the oculomotor motor nucleus shown in Figure 18.2. They synapse in the ciliary ganglion in the back of the orbit. Post-ganglionic axons travel with the short ciliary nerves of the ophthalmic trigeminal nerve to enter the eyeball. The Edinger–Westphal nucleus receives inputs from the pretectal area and superior colliculus which are the areas in which collateral axons from the optic radiations terminate. The optic nerves and oculomotor nerves form reflex arcs for the pupillary light and accommodation reflexes through the tectal areas of the midbrain. The sphincter pupillae muscles constrict the pupils in response to bright light to prevent damage to the retina. The ciliary muscles thicken the lens and increase its curvature. This has the effect of focusing near objects on to the retina and is part of the accommodation reflex that occurs when changing your view from a distant object such as the screen in a lecture theatre to a near object such as your notepad on the bench. The accommodation reflex also involves pupillary constriction and convergence of the eyes.
18.5.3 The trochlear nerves
Each trochlear nerve innervates only the superior oblique muscle on each side. The trochlear nerves are consequently very thin, about the size of a strand of cotton, and, therefore, difficult to observe. The trochlear nerves are unique in that all the axons beginning in the trochlear nuclei cross the midline within the midbrain to supply the superior oblique of the contralateral eye. Furthermore, as shown in Figure 15.12, they are the only cranial nerves that emerge from the dorsal surface of the brainstem where they emerge below the contralateral inferior colliculus.
Fig. 18.5 The origin of the cranial nerves from the brainstem.
As shown in Figure 18.4, the superior oblique muscles attach to the common tendinous ring in the posterior part of the orbit and run forwards between the medial wall and roof of the orbit. They then pass through a fibrous pulley and turn back on to the globe. This apparently bizarre course converts a backward pull into the correct direction. The Latin word for pulley is ‘trochlea’, hence the name of this nerve. Because the trochlear nerves are so flimsy and exit from the posterior midbrain, they often />
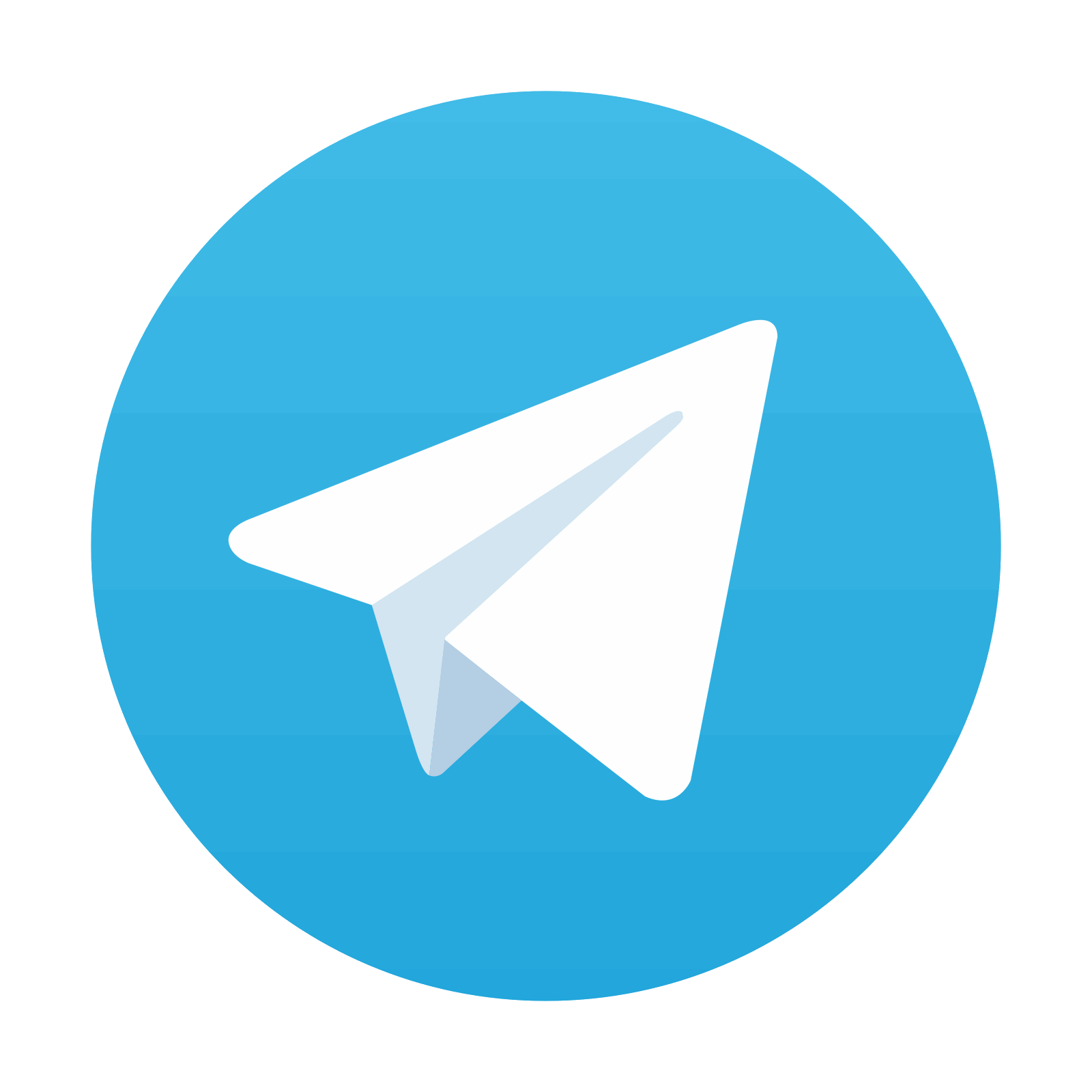
Stay updated, free dental videos. Join our Telegram channel

VIDEdental - Online dental courses
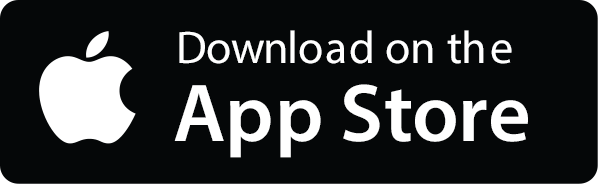
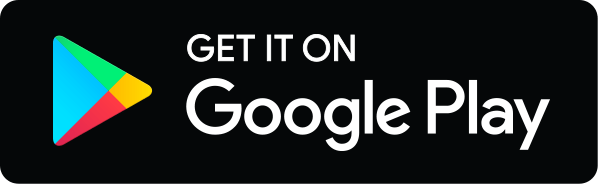