Localized Bone Augmentation and Implant Site Development
Periodontal bone loss, gingival recession, tooth loss, and long-term use of removable appliances typically result in alveolar defects that prevent the placement of implants in an optimal prosthetic position. It also leads to soft-tissue deficiencies that are unacceptable. Fortunately, continuous innovations in surgical techniques and advances in the biologic understanding of bone-regenerative techniques have led to advanced implant procedures and an increased predictability in the reconstruction of alveolar ridge defects.27,38
Standard implant placement surgery, as described in Chapter 75, is based on adequate bone volume and quality in the desired implant location. The time-tested standard protocol allows for adequate remodeling and maturation of bone, with healing periods of 3 to 6 months. Recent implant procedures often challenge these original conventions by placing implants in areas with inadequate bone volume, simultaneous with bone augmentation, and restoring or loading implants after shorter healing periods. This chapter presents an overview of surgical bone augmentation procedures used to correct or to prevent alveolar ridge deficiencies for the optimal placement of dental implants.
Guided Bone Regeneration
Periodontal studies during the last several decades have led to new techniques and a new treatment approach referred to as guided tissue regeneration (GTR). Briefly, this concept is based on the principle that specific cells contribute to the formation of specific tissues. Exclusion of the faster-growing epithelium and connective tissue from a periodontal wound for a minimum of 6 to 8 weeks allows the slower-growing tissues to occupy the space adjacent to the tooth. Osteoblasts, cementoblasts, and periodontal ligament cells are then afforded the opportunity to regenerate a new periodontal attachment (defined by new bone and new connective tissue fibers inserted into newly formed cementum) on the previously diseased root surface. Chapter 61 includes a discussion on the concepts of GTR as related to periodontal regeneration.
The same basic principle of GTR has been applied to alveolar bone defects to regenerate new bone.13 Using a canine model, Schenk et al47 demonstrated with histology that bone regeneration in membrane-protected defects healed in a sequence of steps that simulated bone formation after tooth extraction. They found that after blood clot formation, bone regeneration was initiated by the formation of woven bone initially along new blood vasculature at the periphery of the defect. The new vascular supply emanated from the existing bone bed (recipient site). Cortical perforations surgically created in the recipient site are thought to enhance the blood supply and cellular access to the bone grafted area. The woven bone, which is formed quickly with a disorganized, immature structure, was subsequently replaced by lamellar bone with an organized, mature structure. Over time, bone remodeling continued with new, secondary osteons being formed.
Interestingly, long before the current concepts of GBR were introduced, Murray and coworkers40 demonstrated that when a cavity with a source of osteoblasts and a blood supply was isolated from adjacent soft tissues, it could fill with bone, whereas if the space were not protected, it would fill with fibrous connective tissue. In addition to this observation, they suggested that a bone graft placed in the space might interfere with bone formation because the graft would need to be resorbed before bone could occupy the space.
Bone is a unique tissue that has the capacity to regenerate itself completely. Because of its rigid calcified structure, however, bone has specific requirements that must be respected to achieve regeneration. Because the calcified structure of bone is not conducive to perfusion, new bone formation is critically dependent on establishing an adequate blood supply through the ingrowth of new vasculature while maintaining rigid fixation or stabilization for bone formation. Any movement of the segments of bone relative to one another (even micromotion) during healing results in disruption of the blood supply and a change in the type of tissue formed in the site from mineralized bone to fibrous connective tissue. Table 76-1 lists the biologic requirements for bone regeneration and the associated component of GBR surgical procedures needed to accomplish bone regeneration.
TABLE 76-1
Biologic Requirements for Bone Regeneration
Requirement | Surgical Procedure |
Blood supply | Cortical perforations |
Stabilization | Fixation screws, membrane tacks |
Osteoblasts | Autogenous bone (graft or recipient site) |
Confined space | Barrier membrane |
Space maintenance | Tenting screws, bone graft materials |
Wound coverage | Flap management, tension-free suturing |
Barrier Membranes
Nonresorbable Barrier Membranes.
Space can be maintained under a barrier membrane with bone graft material or tenting screws, thereby facilitating the regeneration of increased bone volume. Stiffer or titanium-reinforced (TR) membranes (Gore and Associates, Flagstaff, AZ) with space-maintaining capabilities can regenerate bone without the need for bone grafts or tenting apparatus.36,47 Stiffer membranes are able to promote significant amounts of new bone and maintain sufficient space without the addition of supportive devices. Ridge augmentation can be enhanced with a TR membrane in conjunction with implant placement in localized bone defects.24
Resorbable Barrier Membranes.
A possible disadvantage is that many resorbable membranes degrade before bone formation is completed, and the degradation process may produce varying degrees of inflammation.61 Recent developments include cross-linking of collagen to increase resistance to biodegradation and thus increase longevity of the barrier function.17 Fortunately, the mild inflammatory reaction caused by bioresorbable membranes does not seem to interfere with osteogenesis. Another disadvantage is that resorbable barrier membranes are quite pliable. The lack of stiffness often results in collapse of the membrane into the defect area.46 Thus resorbable membranes are best suited for situations that allow the graft material or hardware (tenting screws, plates) or the adjacent alveolar bone to maintain the desired dimensions.
Human histology has demonstrated that resorbable barrier membranes support the growth of new bone when used in GBR procedures for horizontal, vertical, sinus, and extraction socket defects.18,20,37 They have also been shown to reduce bone resorption when used over a monocortical bone block to augment horizontally deficient ridges.58 Geurs et al20 demonstrated that a bioabsorbable barrier membrane, used in a GBR procedure along with an allograft, was able to facilitate new bone formation. At present, it can be stated that biodegradable membranes have the potential to support bone formation if they are supported by bone graft material to resist collapse and if they are long-lasting enough to maintain their barrier function for extended periods in small to moderate bone defects.28,29
Bone Graft Materials
Osteoinduction involves new bone formation through stimulation of osteoprogenitors from the defect (or from the vasculature) to differentiate into osteoblasts and begin forming new bone. This induction of the bone-forming process by cells that would otherwise remain inactive occurs through cell mediators that “turn on” these bone-forming cells. The most widely studied of these mediators is the family of bone morphogenic proteins (BMPs). See Chapter 77 for a review of BMP use in bone augmentation.
Numerous bone graft materials have been used to aid in the reconstruction of bone defects. These range from allografts (derived from the same species) to xenografts (derived from a different species) and alloplast or synthetic graft materials. At a minimum, bone graft materials should be osteoconductive. Bone graft materials that are also osteoinductive are believed to be more advantageous than those that are only osteoconductive. Table 76-2 lists the properties of different classes of bone graft materials.
TABLE 76-2
Biologic Properties of Various Bone Graft Materials
Source | Osteoconductive | Osteoinductive | Osteogenic |
Alloplast | Yes | No | No |
Xenograft | Yes | No | No |
Allograft | Yes | Yes/No? | No |
Autograft | Yes | Yes | Yes |
Demineralized freeze-dried bone allograft (DFDBA) is thought to have osteoinductive effects because viable BMPs within the donor tissue matrix are exposed by the decalcification process.57 In contrast to this view, more recent reports suggest that bone augmentation with DFDBA is not osteoinductive because it does not contain the BMPs necessary to induce bone formation.4,8 Schwartz et al48 reported that variations in the amount of bone formation induced by BMPs in DFDBA may be related to the source (i.e., donor tissue) of the bone and the techniques used to process it. In addition to processing variations, it has been demonstrated that young donor bone results in significantly greater quantities of BMPs retained in the bone allograft matrix compared with older donor bone.49 Therefore the source of donor bone can greatly influence its osteoinductive capacity.
Autogenous Bone
A retrospective analysis of 48 chin graft–harvesting procedures, suggests that maintaining a 5-mm margin of safety between graft harvest sites and vital structures (e.g., lower incisors, the inferior border of the chin, and the mental foramen) will minimize postoperative complications.21 In the 48 procedures, postoperative sequelae included bruising of the lower face (48/48), bruising of the upper neck (6/48), and paresthesia of the lower lip and incisors (6/48). No patient experienced facial disfigurement or muscle prolapse (chin droop). Three of the six patients with paresthesia experienced transient symptoms and recovered completely within 2 months, whereas symptoms persisted longer than 6 months in the other three patients. Not surprisingly, the larger harvest defects (trephined six-ring sites) resulted in a higher incidence of paresthesia, which was longer lasting than that of the smaller defects (trephined four-ring sites). Harvesting bone in a custom-shaped “block” did not result in paresthesia, presumably because these harvest sites were smaller than the four-ring and six-ring trephine-harvested sites.
Observation of the following basic principles can minimize the risk of postoperative morbidity:
1. Carefully evaluate the harvest site for potential risks. A critical radiographic evaluation before surgery can identify individuals with inferior alveolar nerve branches that extend anterior beyond the mental foramen.
2. Use extreme care in making incisions laterally toward the mental nerve, and dissect the area with blunt instruments to locate the foramen.
3. Do not elevate and reflect muscle attachments beyond the inferior border of the mandible.
4. Limit bone cuts to an area at least 5 mm away from the tooth apices, the inferior border of the mandible, and the mental foramen. Do not extend cuts or harvest bone deeper than 6 mm, and do not include both labial and lingual cortical plates.
5. Suture the wound in layers (muscle and overlying mucosa separately) to prevent postoperative wound separation.
When harvesting autogenous bone, regardless of site or method used, it is important to use techniques that prevent overheating and maintain viability of the bone cells. Exceeding 47° C (116.6° F) is known to cause bone necrosis.16 Thus the use of drills, trephines, or saws to cut bone should always be done with profuse irrigation to keep instruments and bone cooled. Precision of osseous cuts can be facilitated with new technologies such as piezoelectric bone surgery (see Chapter 80).
Localized Ridge Augmentation
Surgical reconstructive procedures for the preparation and placement of dental implants have become more numerous and complex. Depending on the size and morphology of the defect, various augmentation procedures can be used. These procedures have been categorized according to the deficient dimension: horizontal or vertical. Methods used to augment horizontal, as well as vertical, bone deficiencies include particulate bone grafts and monocortical block grafts. Barrier membranes can be used with bone grafts to reconstruct all types of alveolar bone defects. See Chapter 77 for a review of procedures used to achieve vertical augmentation. All the proven principles of GBR and flap management must be followed to achieve good results. These include an adequate blood supply; maintaining a stable, protected space for bone growth; and achieving tension-free flap wound closure.
Flap Management
Soft-tissue management is a critical aspect of bone augmentation procedures. Incisions, reflection, and manipulation should be designed to optimize blood supply and wound closure. The design and management of mucoperiosteal flaps must consider the increased dimensions of the ridge after augmentation as well as esthetics and approximation of the wound margins. The surgical procedure must be executed with the utmost of care in order to preserve the vascularity of the flap and to minimize tissue injury.1
Several flap techniques maintain a “submerged” position of bone grafts and barrier membranes during the entire healing process, including a remote or displaced incision.9,25 The advantage of a remote incision is that the wound opening is positioned away from the graft. A conventional crestal incision can be used, even in large supracrestal defects, as long as a periosteal releasing incision and coronal advancement of the flap achieve a tension-free closure.30 Most reports suggest removing sutures approximately 10 to 14 days after surgery. It is also suggested that no prosthesis be inserted for 2 to 3 weeks after surgery to avoid pressure over the wound during the early healing phase.
General concepts for flap management associated with ridge augmentation include the following:
1. It is desirable to make incisions remote relative to the placement of barrier membranes (e.g., vertical releasing incisions at least one tooth away from the site to be grafted). In the anterior maxilla, keeping vertical incisions remote is also an esthetic advantage.
2. Full mucoperiosteal flap elevation at least 5 mm beyond the edge of the bone defect is desirable.
3. The use of vertical incisions, although often required for surgical access, should be minimized.
4. Use of a periosteal releasing incision to give the flap elasticity and permit tension-free suturing is essential. This permits complete closure without stress on the wound margins.
5. Removable appliance should not be inserted over the wound for 2 weeks or more to avoid postoperative trauma to the surgical site.
6. Wound closure should incorporate a combination of mattress sutures to approximate connective tissues and interrupted sutures to adapt wound edges.
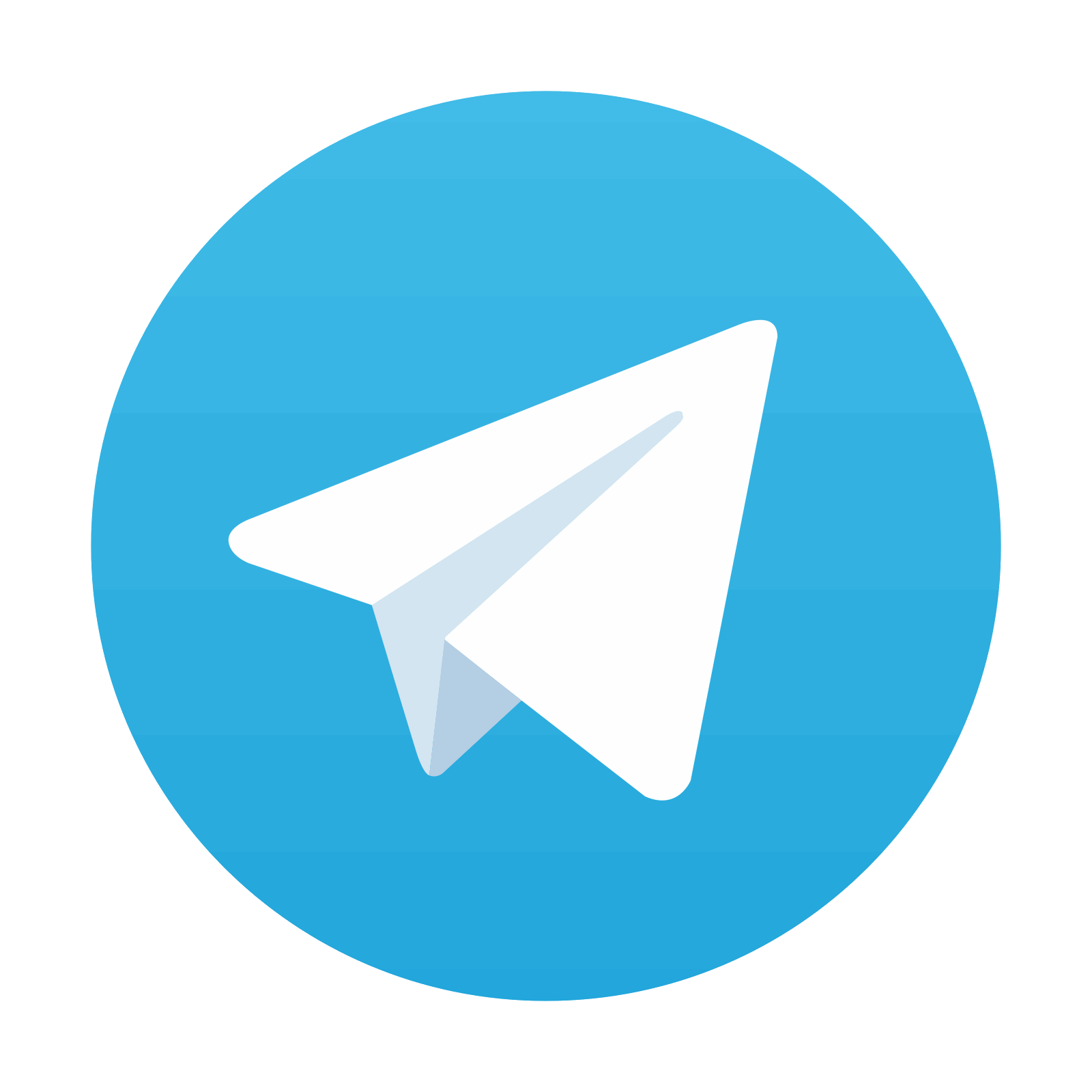
Stay updated, free dental videos. Join our Telegram channel

VIDEdental - Online dental courses
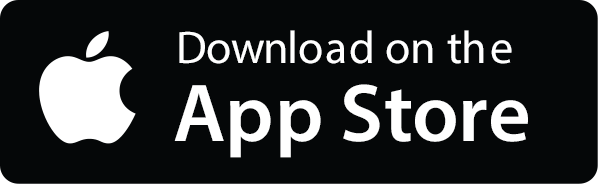
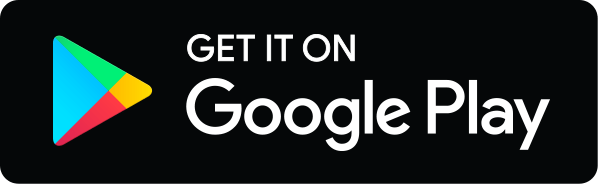