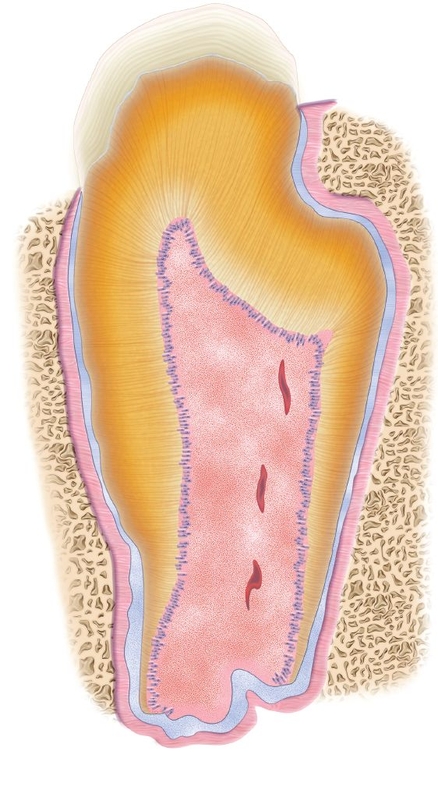
CHAPTER 22
De Novo Tooth Engineering to Replace Lost Teeth
All things are made new.
—Saint Paul
Certain animals, such as newts and hydra, have regenerative capacities. In general, humans lack this ability, although some human cells have innate abilities to replenish and repair injured tissues and organs. In particular, skin and liver are known to undergo regeneration. Stem cells, which exist in most human tissues and differentiate into tissue-forming cells, play important roles in this regenerative process. Because the use of stem cell–based therapies has enormous potential to improve human health, the knowledge obtained from stem cell research is laying the groundwork for a new era of regenerative medicine. While the characterization of stem cells is proceeding, many barriers remain to the application of these therapies in a clinical setting. As described in this chapter, the tooth is one of the organs in the human body that lacks sufficient regenerative capacity.
Tooth loss, whether resulting from caries, disease, trauma, or genetic predisposition, is always accompanied by alveolar bone loss.1,2 However, studies using transgenic mouse models suggest that tooth development is not dependent on bone formation.3–5 Because adult teeth are not shed and do not regrow, artificial dentition, tooth transplantation, and dental implants are often necessary to recover lost masticatory function. Complete restorative therapy including tooth, alveolar bone, and gingival tissues has not been attempted at present but most certainly will be achieved in the future.
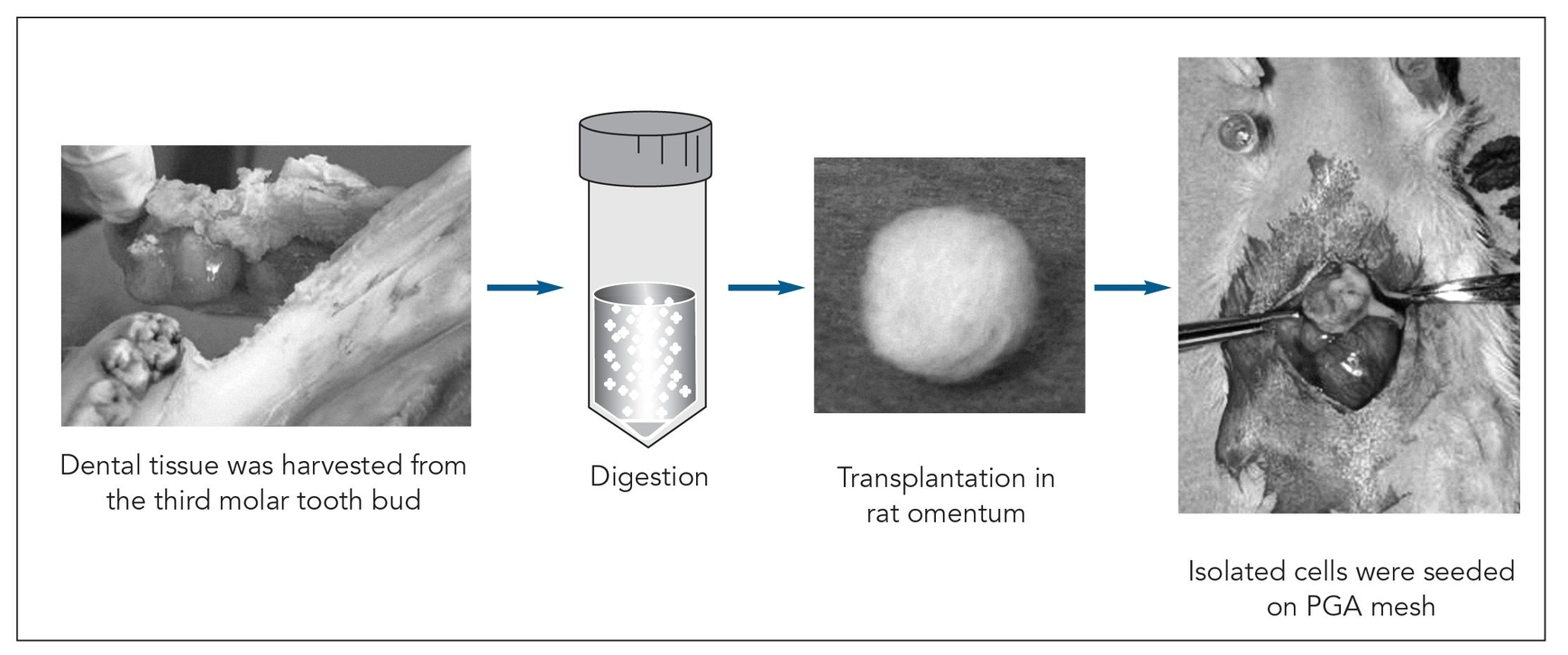
Fig 22-1 Strategy used to generate a tissue-engineered tooth from the third molar tooth bud of a 6-month-old pig. PGA—polyglycolic acid. (Reprinted from Honda et al10 with permission.)
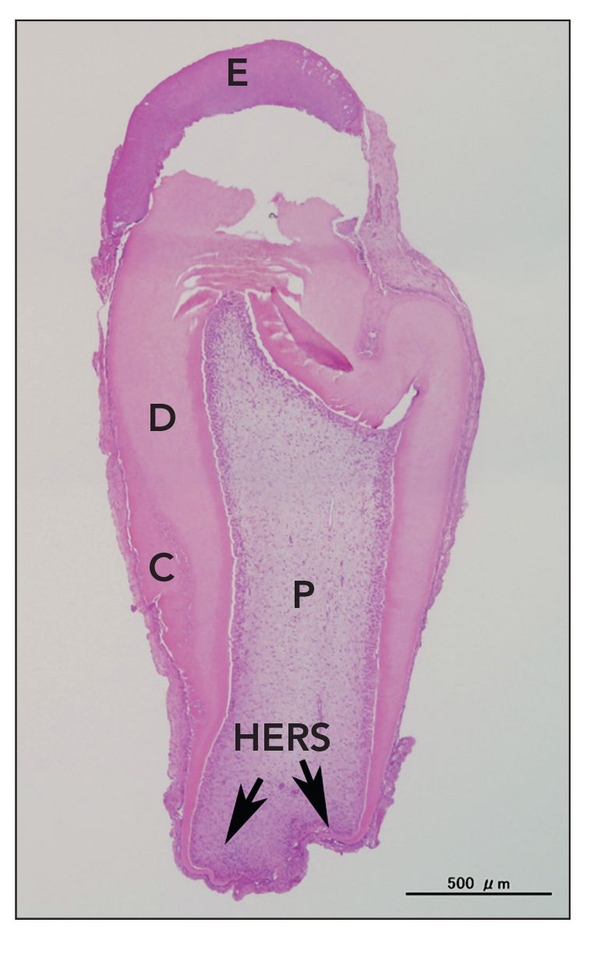
Fig 22-2 Tooth structure generated after seeding of dissociated tooth bud cells onto biodegradable scaffolds. At 25 weeks posttransplantation, a typical tooth with the regular shape was observed in implants obtained from a collagen sponge scaffold. However, the occurrence represented less than 5% of total tissue-engineered teeth. E—enamel; D—dentin; C—cementum; P—pulp; HERS—Hertwig epithelial root sheath (hematoxylin-eosin stain). (Reprinted from Sumita et al11 with permission.)
Given the successful use of dental implant and alveolar restorative procedures, a few questions must be asked: Is present treatment completely adequate? What is the ideal method to replace a missing tooth, especially when the tooth loss is associated with bone loss? Could the ideal method for the restoration of lost teeth and bone ultimately be entirely biologic?
Recent scientific study has focused on the use of stem cells or bioengineered scaffolds to replace missing teeth. Young et al6 first advocated the concept of generating a whole tooth using the principles of classic tissue engineering and, subsequently, reported their seminal work on tooth tissue engineering.7–9 Complex tooth structures were generated by seeding dissociated tooth bud cells onto biodegradable scaffolds, which were then grown in the omentum of immunodeficient rats for an extended period of time (Fig 22-1). Generated tooth structures displayed dentin at 20 weeks and enamel by 25 weeks posttransplantation (Fig 22-2). In addition, the developmental process of the generated tooth followed a process similar to that of the natural tooth. This technique successfully generated all tooth tissues, except periodontal ligament and the dental nerve, suggesting that dental progenitor and stem cells are present in developing molars.9,10
In theory, the patient’s own dental progenitor or stem cells could fulfill the function of lost tooth replacement. However, to produce a viable tissue-engineered tooth, four key milestones must be passed: (1) The size and shape of the engineered tooth must be predictable, controllable, and indistinguishable from the patient’s own tooth; (2) the periodontium must be present; (3) an alternative cell source must be identified because it is difficult to obtain tooth bud tissue from a patient; and (4) viable teeth must develop intra-alveolarly within the patient’s jaw and functionally progress into occlusion by the natural eruption process. The research progress of these four key elements of dental tissue engineering will be reviewed in this chapter.
Regulation of the Size and Shape of Tissue-Engineered Teeth
Tissue engineering is based on the plating of cells from an organ or tissue onto a prefabricated biodegradable scaffold to generate an alternative organ or tissue.12 In one study, porcine tooth buds harvested from the mandible at 6 months of age were dispersed by digestion as cell suspensions onto biodegradable scaffolds and subsequently grown in nude rat omentum. One-third of the molar bud cells within a single scaffold produced a tiny tooth structure. The mean dimension of the structure was approximately 2 × 3 mm by 20 to 25 weeks after transplantation. 6 The natural length of the porcine mandibular third molar is approximately 30 mm. Thus, the tissue-engineered tooth was only about one-tenth as long as the natural tooth. For the production of tissue-engineered teeth, therefore, it is necessary to understand which factors regulate tooth size.
Mechanical stress exerts a variety of effects on growth and differentiation of bone cells, such as osteoblasts and chondrocytes.12–14 Because shear stress facilitates secretion of bone matrix, it was hypothesized that appropriate shear stress may be essential for complete differentiation of a tooth bud, which may in turn increase the size of the tissue-engineered tooth. A study was designed to determine whether shear stress affects or regulates the size of a newly generating tooth. Tooth bud cells harvested from the porcine third molars at the stage of crown formation were seeded onto a biodegradable scaffold.8 Before transplantation, the cell-polymer constructs were exposed to shear stress in vitro for 12 hours.
At 15 weeks posttransplantation, the implants contained solid tissue. Implants exposed to shear stress grew to approximately 6 × 6 mm (Fig 22-3a), whereas implants not exposed to shear stress were approximately 2 × 2 mm (Fig 22-3b), a threefold difference. In addition, radiographic analysis revealed that calcified tissues occupied most of the area of the exposed implants (Figs 22-3c and 22-3d). Surprisingly, structures of the enamel-dentin complex formed by week 15, in contrast to the 20 to 25 weeks required for the previously used method.6,10
Odontogenesis is a complex phenomenon that involves differentiation of various cell types, extracellular matrix deposition, and mineralization. The results of this study showed that shear stress enhances odontogenic differentiation in vitro, which leads to early deposition of extracellular matrix and subsequent mineralization. This is the first report to show the effects of shear stress on odontogenic cell differentiation.8
There are limitations to applying this technology to clinical settings, however, because morphogenesis to date has resulted in teeth of irregular shape. Nevertheless, these findings may be useful in eventually generating tissue-engineered teeth.
Tissue-engineered tooth structures
Teeth generated in nude rat omentum rarely show morphogenesis similar to that of natural teeth; fewer than 10% of the generated teeth have a normal appearance.11 Most of the generated tooth tissues are rounded or stick shaped, despite the fact that histiogenesis appears to progress normally.11 Because the environment within the jaw itself must provide certain temporal cues for tooth generation, tissue-engineered product was directly transplanted into canine jaw.7 Tooth bud cells were harvested from canine first molars at the crown formation stage, and this heterogenous cell population was seeded onto a biodegradable scaffold. An autografting technique was used for this purpose. The cell-scaffold–based constructs were transplanted intra-alveolarly into extraction sockets in the mandible.
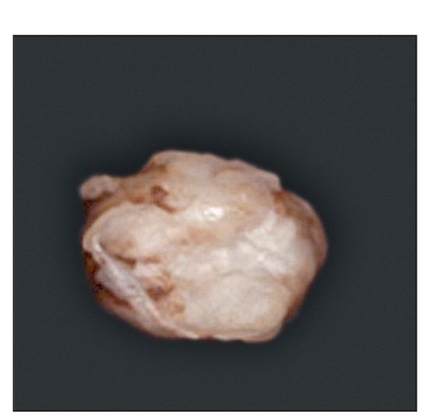
Fig 22-3a Gross appearance, 15 weeks posttransplantation, of a tissue-engineered tooth from a tooth bud cell exposed to shear stress. (Reprinted from Honda et al8 with permission.)
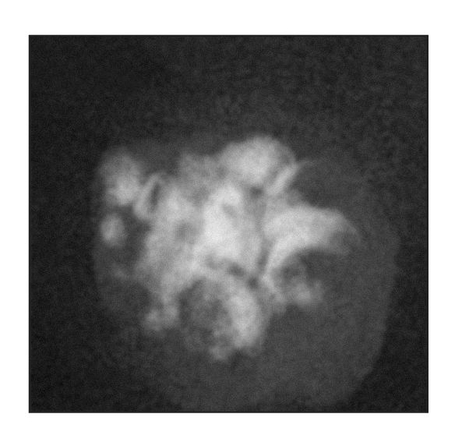
Fig 22-3b Gross appearance, 15 weeks posttransplantation, of a tissue-engineered tooth from a tooth bud cell not exposed to shear stress. (Reprinted from Honda et al8 with permission.)
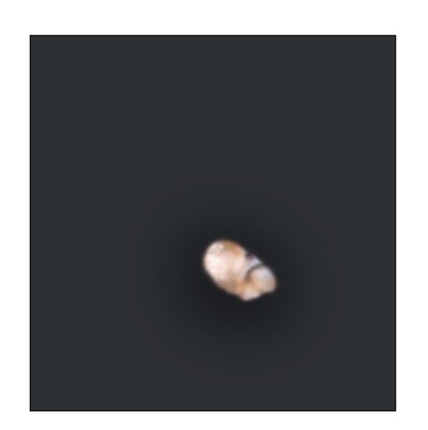
Fig 22-3c Radiographic analysis reveals that calcified tissues are readily observed in the exposed implants. (Reprinted from Honda et al8 with permission.)
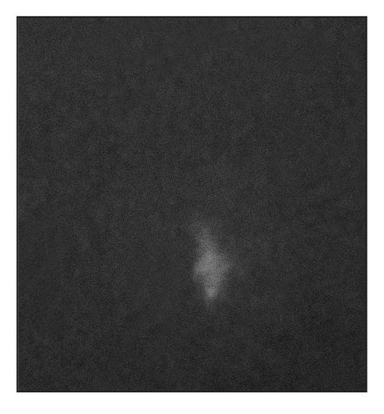
Fig 22-3d Radiographic analysis reveals only a small calcification in the nonexposed implants. (Reprinted from Honda et al8 with permission.)
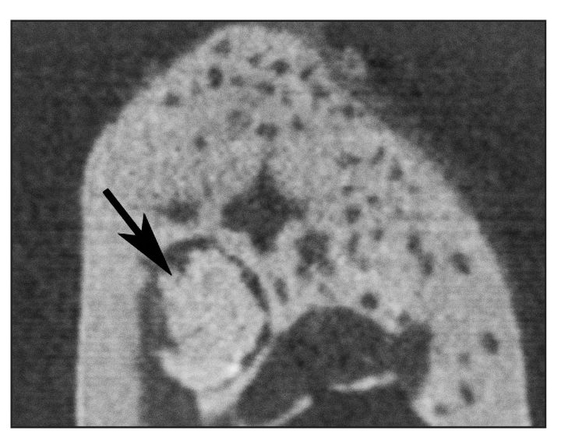
Fig 22-4a Reconstructed coronal cone beam microcomputed tomogram of a biopsy specimen. Round tissue (newly generated tissue) is observed in the mandible (arrow). (Reprinted from Honda et al7 with permission.)
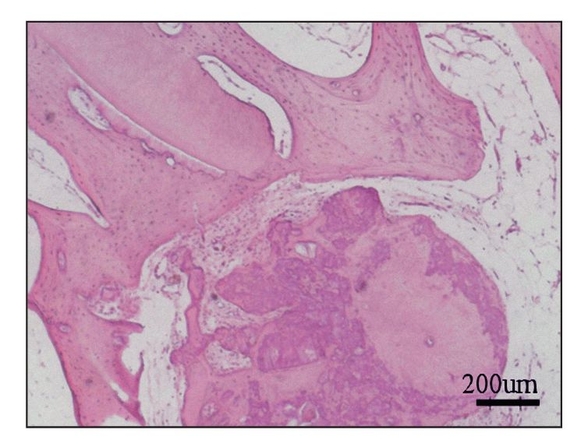
Fig 22-4b Section of generated tissue, 24 weeks posttransplantation. Dentin and bone tissues are apparent within the generated tissue (hematoxylin-eosin stain). (Reprinted from Honda et al7 with permission.)
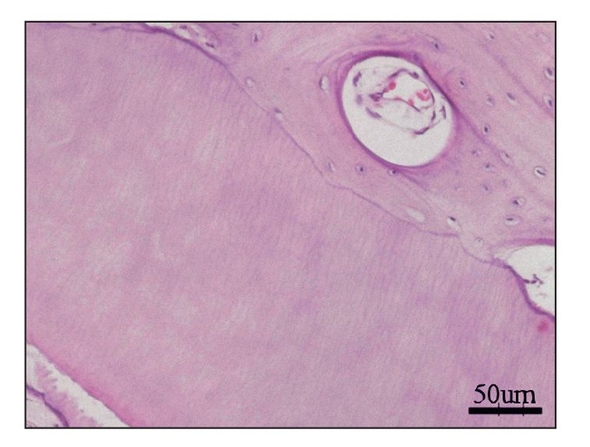
After 24 weeks, microcomputed tomography revealed a mass of calcified tissue in implant locations, although there was no evidence of emergence from the jaw (Fig 22-4a). Histologic analysis revealed a calcified mass consisting of bone and dentin. No tooth morphology was discernible (Fig 22-4b).7 Although the adult jaw environment contributed to the generation of dentin and bone (Fig 22-4c), these results suggest that fully developed jawbone does not regulate morphogenesis of developing tissue-engineered teeth.
Sequential seeding to regulate tooth number
To date, experiments to investigate the effects of alternative scaffolds,11 shear stress,8,15 and the alveolar jaw environment 7 have been performed on the regulation of the morphogenesis of a tissue-engineered tooth. Despite these approaches at regulation, generated teeth show abnormal morphogenesis with high variability in shape, and multiple teeth are usually generated in one scaffold (Fig 22-5).7,8,10,11 Alternatively, teeth generated from embryonic tooth bud cells in combination with scaffolds develop normal-shaped crowns.17 Thus, it is conceivable that postnatal tooth bud cells lose essential factors for regulation of tooth morphogenesis. Therefore, novel methods are required to solve this issue before generation of tissue-engineered teeth can be established.
Mammalian tooth development is regulated by reciprocal epithelial-mesenchymal interactions.18,19 Oral epithelium at the initiation stage has the potential to induce tooth initiation20,21 as shown with embryonic recombinant techniques. With this approach, an intact murine embryonic oral epithelium at embryonic day 10 is combined with isolated nondental mesenchymal cells. Recombination generates a tooth crown in vivo.21 After day 11, in the mouse, this potential transfers to the dental mesenchyme, which becomes capable of directing tooth morphogenesis.20–26
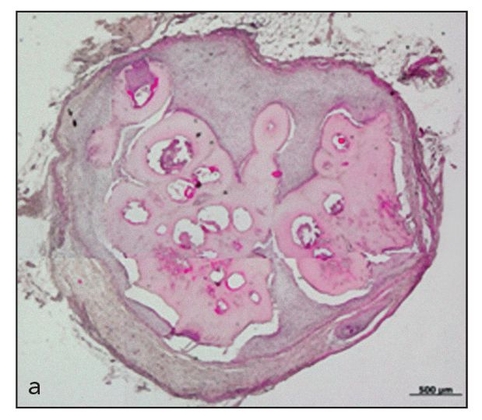
Fig 22-5a Engineered single dental tissue, consisting mainly of dentin, generated by 20 weeks posttransplantation (hematoxylin-eosin stain). (Reprinted from Honda et al16 with permission.)
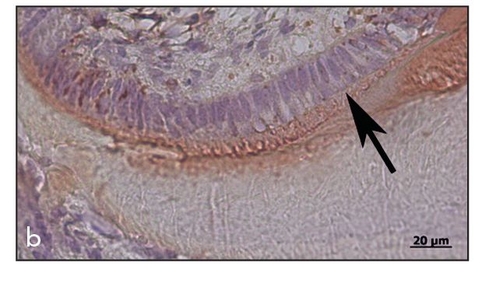
Fig 22-5b Increased magnification showing the perpendicular alignment of the ameloblasts (arrow) at the enamel surface, with immunopositive staining for amelogenin (immunohistochemistry stain for amelogenin). (Reprinted from Honda et al16 with permission.)
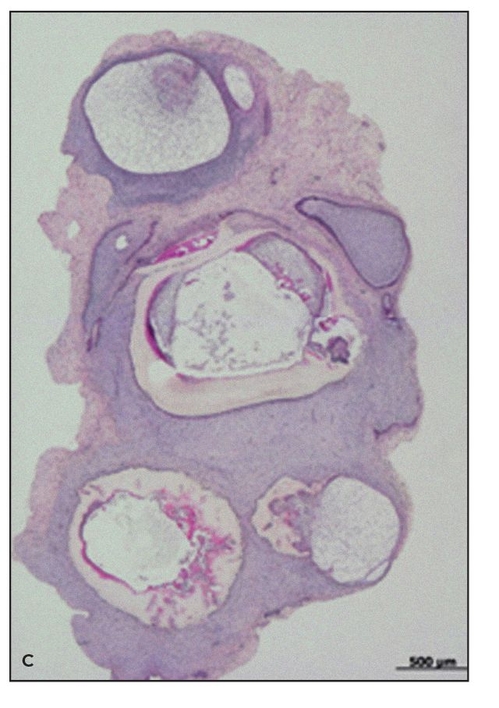
Fig 22-5c Multiple tooth tissues generated in a single scaffold after random seeding of the scaffold (hematoxylin-eosin stain). (Reprinted from Honda et al16 with permission.)
For a ti/>
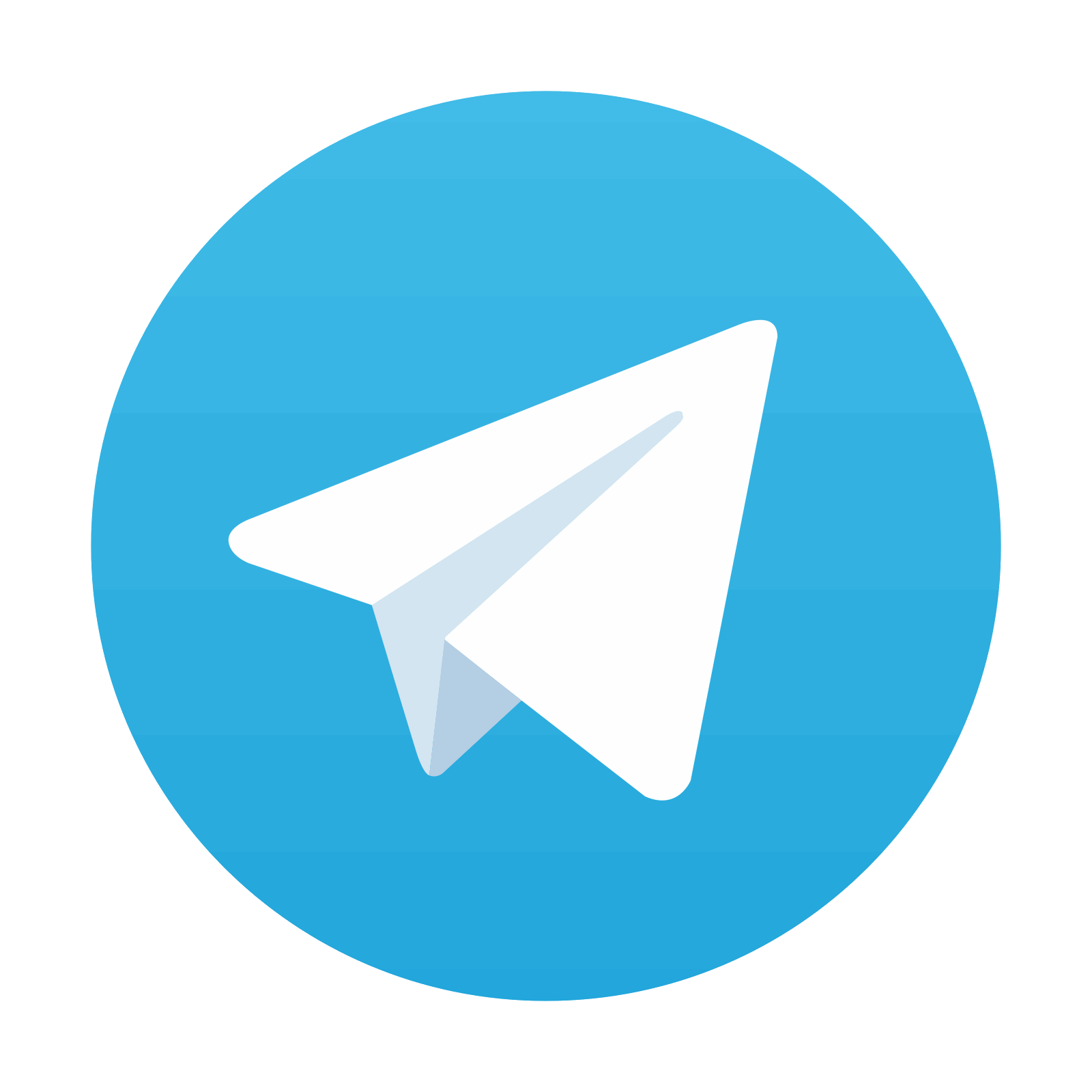
Stay updated, free dental videos. Join our Telegram channel

VIDEdental - Online dental courses
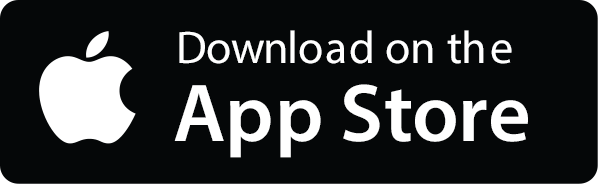
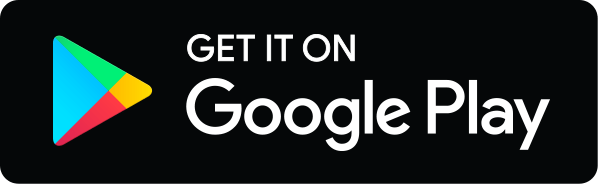