Biology
Radiobiology is the study of the effects of ionizing radiation on living systems. This discipline studies many levels of organization within biologic systems spanning broad ranges in size and time (Fig. 2-1). The initial interaction between ionizing radiation and matter occurs at the level of the electron within the first 10−13 seconds after exposure. These changes result in modification of biologic molecules within the following seconds to hours. The molecular changes may lead to alterations in cells and organisms that persist for hours, decades, and possibly generations. These changes may result in injury or death.
Radiation Chemistry
Radiation acts on living systems through direct and indirect effects. When the energy of a photon or secondary electron ionizes biologic macromolecules, the effect is termed direct. Alternatively, a photon may be absorbed by water in an organism, ionizing some of its water molecules. The resulting ions form free radicals that interact with and produce changes in biologic molecules. Because intermediate changes involving water molecules are required to alter the biologic molecules, this series of events is termed indirect.
Direct Effect
In direct effects, biologic molecules (RH, where R is the molecule and H is a hydrogen atom) absorb energy from ionizing radiation and form unstable free radicals (atoms or molecules having an unpaired electron in the valence orbital). Generation of free radicals occurs in less than 10−10 seconds after interaction with a photon. Free radicals are extremely reactive and have very short lives, quickly reforming into stable configurations by dissociation (breaking apart) or cross-linking (joining of two molecules). Free radicals play a dominant role in producing molecular changes in biologic molecules.
Free radical production:
< ?xml:namespace prefix = "mml" ns = "http://www.w3.org/1998/Math/MathML" />

Because the altered biologic molecules differ structurally and functionally from the original molecules, the consequence is a biologic change in the irradiated organism. Approximately one third of the biologic effects of x-ray exposure result from direct effects.
Indirect Effects
Because water is the predominant molecule in biologic systems (about 70%), it frequently participates in the interactions between x-ray photons and biologic molecules. A complex series of chemical changes occurs in water after exposure to ionizing radiation. Collectively, these reactions result in the radiolysis of water:

Although the radiolysis of water is complex, on balance, water is largely converted to hydrogen and hydroxyl free radicals.
Indirect effects are effects in which hydrogen and hydroxyl free radicals, produced by the action of radiation on water, interact with organic molecules. The interaction of hydrogen and hydroxyl free radicals with organic molecules results in the formation of organic free radicals. About two thirds of radiation-induced biologic damage results from indirect effects. Such reactions may involve the removal of hydrogen:
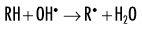
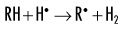
The OH• free radical is more important than H• in forming organic free radicals (R•). The resulting organic free radicals are unstable and transform into stable, altered molecules as described in the previous section on direct effects. These altered molecules have different chemical and biologic properties from the original molecules.
Radiation effects are thus caused primarily by direct effects and the diffusion of OH•. Both direct effects and indirect effects are completed within 10−5 seconds. The resulting damage may take hours to decades to become evident.
When dissolved oxygen is present, as is the case in normal tissues, hydroperoxyl free radicals may also be formed:
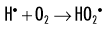
Hydroperoxyl free radicals contribute to the formation of hydrogen peroxide in tissues:
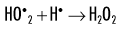

Both peroxyl radicals and hydrogen peroxide are oxidizing agents and are the primary toxins produced in the tissues by ionizing radiation. The reactions of these oxidizing agents with organic molecules are another form of indirect effects.
DNA Changes
Damage to a cell’s deoxyribonucleic acid (DNA) is the primary cause of radiation-induced cell death, heritable (genetic) mutations, and cancer formation (carcinogenesis). Radiation produces many different types of alterations in DNA, including the following:
• Breakage of one or both DNA strands
• Cross-linking of DNA strands within the helix to other DNA strands or to proteins
The most important of these types of damage are single-strand and double-strand breakage. Most single-strand breakage is of little biologic consequence because the broken strand is readily repaired by using the intact second strand as a template. Radiation may also cause clusters of double-strand damage in DNA (Fig. 2-2). A cluster is defined as two or more double-strand breaks within two turns of DNA. Such double-strand breakage is believed to be responsible for most cell killing, carcinogenesis, and heritable effects. A single photon may cause these damage clusters. When damage clusters may result in cell killing, it is good for killing tumor cells. However, when there are not enough clusters to cause cell killing, there is the risk that they will induce mutations that may lead to cancer.
Deterministic and Stochastic Effects
Radiation injury to organisms results from either the killing of large numbers of cells (deterministic effects) or sublethal damage to the genome of individual cells (stochastic effects) that results in cancer formation or heritable mutation. The differences between deterministic and stochastic effects are summarized in Table 2-1. Deterministic effects of radiation are effects seen when the radiation exposure to an organ or tissue exceeds a particular threshold level. The severity of this change is proportional to the dose; greater exposure leads to greater cell killing. At doses below the threshold, the effect does not occur. Stochastic effects are caused by sublethal radiation-induced damage to DNA. They have no minimum threshold for causation. Any dose of radiation has the potential to induce a stochastic effect. The probability of causing a stochastic effect increases as the radiation dose is increased.
TABLE 2-1
Comparison of Deterministic and Stochastic Effects of Radiation
Deterministic Effects | Stochastic Effects | |
Examples | Mucositis resulting from radiation therapy to oral cavity | Radiation-induced cancer |
Radiation-induced cataract formation | Heritable effects | |
Caused by | Killing of many cells | Sublethal damage to DNA |
Threshold dose? | Yes: Sufficient cell killing required to cause a clinical response | No: Even one photon could cause a change in DNA that leads to a cancer or heritable effect |
Severity of clinical effects and dose | Severity of clinical effects is proportional to dose; the greater the dose, the greater the effect | Severity of clinical effects is independent of dose; all-or-none response—an individual either has effect or does not |
Probability of having effect and dose | Probability of effect independent of dose; all individuals show effect when dose is above threshold | Frequency of effect proportional to dose; the greater the dose, the greater the chance of having the effect |
Deterministic Effects on Cells
Intracellular Structures
The effects of radiation on intracellular structures result from radiation-induced changes in their macromolecules. These changes are seen as structural and functional changes in cellular organelles. The changes may cause cell death.
Nucleus
A wide variety of radiobiologic data indicate that the nucleus is far more radiosensitive (in terms of lethality) than the cytoplasm, especially in dividing cells. The sensitive site in the nucleus is the DNA within chromosomes.
Chromosome Aberrations
Chromosomes serve as useful markers for radiation injury. They may be easily visualized and quantified, and the extent of their damage is related to cell survival. Chromosome aberrations are observed in irradiated cells at the time of mitosis when the DNA condenses to form chromosomes. The type of damage that may be observed depends on the stage of the cell in the cell cycle at the time of irradiation.
Figure 2-3 shows the stages of the cell cycle. If radiation exposure occurs after DNA synthesis (i.e., in late S or G2 phase), only one arm of the affected chromosome is broken (chromatid aberration) (Fig. 2-4, A). However, if the radiation-induced break occurs before the DNA has replicated (i.e., in G1 or early S phase), the damage is seen as a break in both arms (chromosome aberration) at the next mitosis (Fig. 2-4, B). Most simple breaks are repaired by biologic processes and go unrecognized. Figure 2-5 illustrates several common forms of chromosome aberrations resulting from incorrect repair. Formation of rings (Fig. 2-5, A) and dicentrics (Fig. 2-5, B) is lethal because the cell cannot complete mitosis. Translocations (Fig. 2-5, C) result in unequal distribution of chromatin material to daughter cells, and they may prevent completion of a subsequent mitosis. Chromosome aberrations have been detected in peripheral blood lymphocytes of patients exposed to medical diagnostic procedures. Additionally, the survivors of the atomic bombings of Hiroshima and Nagasaki have demonstrated chromosome aberrations in circulating lymphocytes more than 2 decades after the radiation exposure. The frequency of aberrations is generally proportional to the radiation dose received.
Cell Replication
Radiation is especially damaging to rapidly dividing cell systems, such as skin and intestinal mucosa, and hematopoietic tissues (Table 2-2). Irradiation of such cell populations causes a reduction in size of the irradiated tissue as a result of mitotic delay (inhibition of progression of the cells through the cell cycle) and reproductive cell death (usually during mitosis). The three mechanisms of reproductive death are DNA damage, bystander effect, and apoptosis.
TABLE 2-2
Relative Radiosensitivity of Various Cells
High | Intermediate | Low | |
Characteristics | Divide regularly | Divide occasionally in response to demand for more cells | Highly differentiated |
Long mitotic futures | When mature are incapable of division | ||
Undergo no or little differentiation between mitoses | |||
Examples | Spermatogenic and erythroblastic stem cells | Vascular endothelial cells | Neurons |
Basal cells of oral mucous membrane | Fibroblasts | Striated muscle cells | |
Acinar and ductal salivary gland cells | Squamous epithelial cells | ||
Parenchymal cells of liver, kidney, and thyroid | Erythrocytes |
DNA Damage
Cell death is caused by damage to DNA, which causes chromosome aberrations that cause the cell to die during the first few mitoses after irradiation. The rate of cell replication in various tissues—and thus the rate of reproductive death—accounts for the varying radiosensitivity of tissues. When a population of slowly dividing cells is irradiated, larger doses and longer time intervals are required for induction of deterministic effects than when a rapidly dividing cell system is involved.
Bystander Effect
Cells that are damaged by radiation release into their immediate environment molecules that kill nearby cells. This bystander effect can cause chromosome aberrations, cell killing, gene mutations, and carcinogenesis.
Apoptosis
Apoptosis, also known as programmed cell death, occurs during normal embryogenesis. Cells round up, draw away from their neighbors, and condense nuclear chromatin. This characteristic pattern, which is different from necrosis, can be induced/>
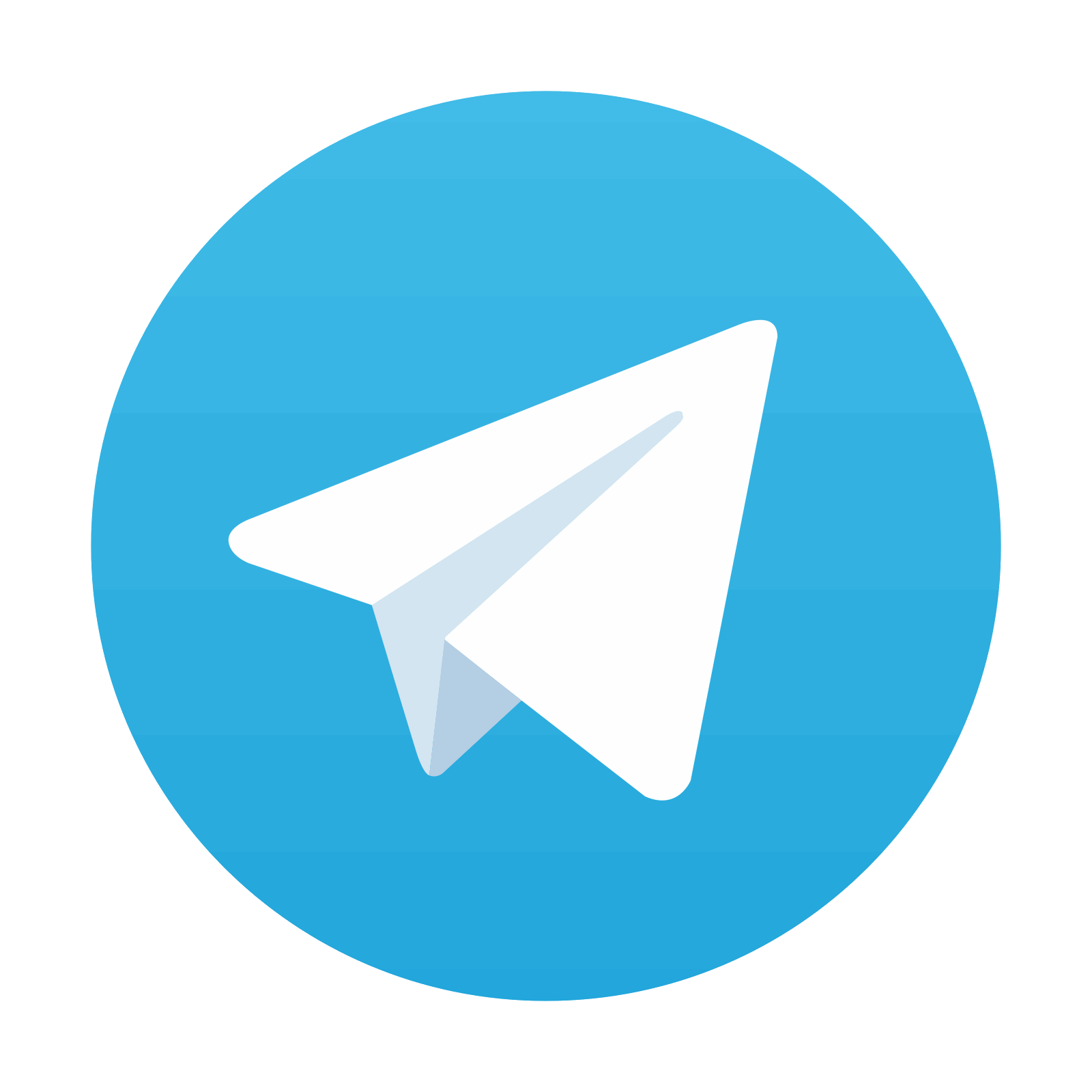
Stay updated, free dental videos. Join our Telegram channel

VIDEdental - Online dental courses
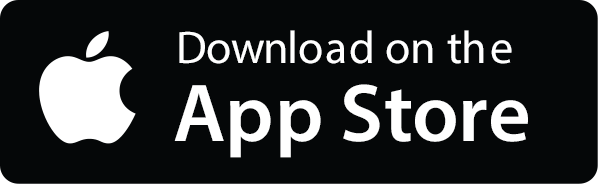
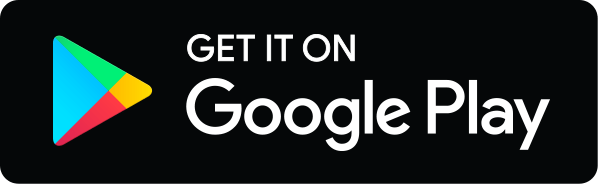