16
Major sensory and motor systems
Chapter contents
16.1 Introduction
The previous chapter provided an overview of the anatomy of the CNS, concentrating on structures that can be seen during dissection of the human brain and spinal cord or the study of anatomical models of these structures. Some indication of the function of different components of the CNS has been given in Chapter 15, but this chapter shows how the various anatomical components of the CNS are functionally linked together through sensory and motor pathways. These pathways enable the nervous system to convey information over considerable distances, to integrate the information, and formulate functional responses that coordinate activities of different parts of the body. It will be necessary to introduce some other structures in addition to those described in Chapter 15 during the description of major pathways; most are not visible to the naked eye and even when seen in microscopical sections, they require considerable practice to distinguish them. However, they are important landmarks or relay stations in the central nervous pathways and you need to know of them for a full understanding of pathways.
As emphasized in Chapter 14, our views of the structure and function of many aspects of the nervous system are constantly subject to revision in the light of new clinical and experimental observations and methods of investigation. This applies to nerve pathways just as much as any other aspect of the nervous system. This chapter presents a summary of current views on somatic sensory and motor functions and their application to the practice of dentistry. The special sensory pathways of olfaction, vision, and hearing are described in Chapter 18 in the context of the cranial nerves that form the first part of these pathways.
16.2 General sensory pathways
The information conveyed from the periphery by the sensory components of spinal and cranial nerves is destined to reach the cerebral cortex or the cerebellum. You will be conscious of sensory information that reaches the cerebral cortex, but mostly unaware of information that does not travel to the cortex. However, this does not mean that sensory information that does not attain cortical levels is of no value. For example, sensory neurons or their collateral processes form the afferent limbs of many reflex arcs. We simply could not function efficiently without proprioception informing us about the state of muscles and joints—another source of information that does not reach consciousness.
Sensory pathways may be classified in several different ways. They can be classified by the type of sensory stimulus they react to such as touch or temperature. Alternatively, they can be described in terms of the response to that sensory information. We can react to a stimulus, e.g. by retracting away from a noxious stimulus or taking a coat off if a room is too hot. Peripheral receptors responding to noxious, temperature, light touch, and pressure stimuli provoke a reaction. We can use sensory information to make fine judgements and discriminate to determine if we are holding a dental instrument tight enough, for example. Receptors for fine touch and vibration convey information used for discriminative purposes. Proprioceptive information from muscles, joints, and ligaments are also required for discrimination. Proprioception is also vital if we are to make meaningful and accurate motor responses to sensory stimuli; we need to know where our bodies are in space, where individual components are, what state our muscles are in, and what position joints are in. The sensory pathways that convey information from receptors sensitive to stimuli that produce a reaction follow one route through the CNS, the spinothalamic pathway, whereas those carrying discriminative information follow another route, the dorsal column–medial lemniscus pathway. Some proprioceptive information reaches the cerebral cortex by the latter pathway to aid discrimination, but the majority is conveyed only as far as the cerebellum by separate spinocerebellar pathways.
The basic plan of reactionary and discriminative pathways is the same. Typically, there are three principal neurons in each pathway between the peripheral receptor and the cerebral cortex.
• Primary sensory neurons are components of spinal or cranial nerves that convey information from the peripheral receptor to the CNS.
• Thalamic projection (or secondary) sensory neurons transfer sensory information within the CNS from the spinal cord or brainstem to the thalamus.
• Thalamocortical (or tertiary) neurons transmit information from the thalamus to sensory cortex.
The arrangement of both pathways is shown in Figure 16.1 and 16.5. Follow the general arrangement of the three-neuron chain in these figures as you read the following description.
In spinal nerves, sensory processes enter the spinal cord through the dorsal roots of the spinal nerves. The cell bodies of primary sensory neurons form swellings on their dorsal roots, the dorsal root ganglia; peripheral and central processes arise from these cell bodies. The peripheral processes travel in spinal nerves to the peripheral receptors and their central processes pass into the spinal cord through the dorsal roots to connect with thalamic projection neurons. The arrangement is essentially similar in the cranial nerves. Those cranial nerves that contain sensory neurons have ganglia near to their connections with the brain, containing the sensory cell bodies; their peripheral processes connect to receptors in the head and neck and their central processes enter the brainstem.
In the spinothalamic pathway, the cell bodies of thalamic projection neurons are situated within the dorsal grey matter of the spinal cord or in sensory cranial nerve nuclei in the brainstem. Note that cranial nerves are not all identical in composition; some are purely motor nerves whereas others are mixed. They only have the equivalent of dorsal and ventral horn grey matter where it is required; the grey matter thus occurs as discrete areas, the cranial nerve nuclei. The cranial nerve nuclei are explained more fully in Chapter 18.
Fig. 16.1 The spinothalamic pathway for temperature, simple touch, and pressure.
Thalamic projection neurons for the dorsal column pathway are located in specific dorsal column nuclei in the lower brainstem. Irrespective of the pathway, axons of thalamic projection neurons cross the midline, usually at the level of their synapse and ascend to the thalamus where they synapse with tertiary neurons. The axons of the tertiary neurons run through the posterior limb of the internal capsule and the corona radiata to reach the somatosensory area of the cerebral cortex in the post-central gyrus. As described in Section 15.4.2 and illustrated in Figure 15.16, sensory projections to the primary sensory cortex are somatotopically represented such that each part of the body is represented in a specific area of the cortex.
The three-neuron pathway outlined above is a simplification. Interneurons occur at the synaptic sites between the principal neurons of the sensory pathways in the spinal cord or brainstem and in the thalamus. These multiple synapse sites enable convergence, divergence, amplification, or inhibition of sensory information as outlined in Chapter 3.
The detailed structure of the two pathways and differences between will now be outlined.
Fig. 16.2 A cross section of the spinal cord to show the positions of the major sensory pathways.
16.2.1 The spinothalamic pathway (the anterolateral pathway)
This pathway conveys noxious, temperature, simple touch, and pressure stimuli to the CNS. It is called the spinothalamic pathway because the secondary neurons pass from the spinal cord to the thalamus. The alternative name indicates the position of this tract in a cross section of the spinal cord; as shown in Figure 16.2, it lies in the anterolateral white matter adjacent to the ventral horn. The spinothalamic pathway is illustrated in Figure 16.1; the pathway should be followed on the diagram as the description is read.
The skin and other tissues contain many types of sensory endings to which the peripheral process of primary sensory neurons connect. Different receptor types have a high degree of selectivity for different sensory modalities.
Nociception is the sensation that arises from stimuli that cause tissue damage. Noxious stimuli generate the unpleasant sensation or emotion that we call pain when they reach consciousness. Unencapsulated free nerve endings in the skin epidermis, other epithelia, and deeper tissues such as ligaments and joint capsules respond to noxious stimuli. Temperature receptors are also free nerve endings. There are both unencapsulated and encapsulated receptors for simple touch. Unencapsulated endings contact specialized cells in the basal layer of epithelia and surround hair follicles. Encapsulated endings of different types are located in the dermis, subcutaneous tissues, and in the capsule of many synovial joints. The processes from the receptors for simple touch and pressure are medium calibre and myelinated. As shown in Figure16.1, the cell bodies of all the types of sensory process already mentioned are located in the dorsal root ganglia of spinal nerves or the corresponding sensory ganglia of those cranial nerves that contain somatic sensory neurons, irrespective of the type of receptor the processes connect to. The sensory ganglia of cranial nerves are usually named after the nerve in question.
Continue tracing these pathways in Figure 16.1, noting that their central processes from cell bodies in the dorsal root ganglia form the dorsal roots of the spinal nerves. As they enter the spinal cord, they divide into ascending and descending branches which travel for one or two segments before entering the dorsal grey horn through the dorsolateral tract (see Figure 15.8).
The primary sensory neurons of the spinothalamic system synapse in different locations, depending on whether they are conveying noxious stimuli or touch and temperature stimuli. Nociceptive primary sensory neurons synapse in the superficial laminae of the dorsal horn directly with thalamic projection neurons that only respond to high threshold noxious stimuli. Neurons that convey thermal and touch information synapse with cells in lamina V of the dorsal horn (see Figure 15.9). These lamina V cells have conventional short dendrites within lamina V as you may expect, but they also have long dendrites that travel right back into lamina I. These cells may, therefore, be stimulated by either high threshold noxious stimuli synapsing in lamina I or low threshold tactile or thermal stimuli synapsing in lamina V referred to as wide dynamic range neurons. The dual stimulation of the thalamic projection cells in lamina V is important in pain localization (see Section 16.2.2.).
There are numerous interneurons between the terminals of primary neurons and the thalamic projection neurons. The primary sensory neurons also make synaptic connections for spinal reflex responses. The thalamic projection neurons receive numerous other inputs, notably from collateral branches of neurons responding to discriminative touch, neurons descending from the brainstem reticular formation, and parietal association cortex. All these connections probably play a role in the modulation of sensation passing through the spinal cord, especially pain.
Figure 16.1 illustrates that the axons of thalamic projection neurons cross the midline at the same level as their cell bodies and pass through the commissural areas to ascend in the contralateral spinothalamic tract located in the anterolateral part of the white matter. Some sources divide the spinothalamic tract into an anterior component carrying touch and pressure and a lateral part conveying noxious and thermal stimuli. The upward continuation of the spinothalamic tract in the brainstem is known as the spinal lemniscus which can be traced in Figure 16.1. Thalamic projection neurons conveying thermal and tactile information synapse in the ventroposterolateral nuclei of the thalamus with tertiary neurons; the processes of the tertiary neurons pass to the primary sensory cortex through the posterior limb of the internal capsule. In contrast, thalamic projection neurons conveying noxious stimuli synapse with tertiary neurons in the posterior nuclei of the thalamus. These tertiary neurons do not project to the somatosensory primary cortex, but to several areas of the cortex and other cerebral structures comprising the pain matrix (see Section 16.2.2.)
The trigeminal (fifth cranial) nerves convey most of the primary sensory neurons carrying nociceptive, thermal, simple touch, and pressure stimuli from the head; their cell bodies are located in the trigeminal ganglia. The glossopharyngeal (ninth) and vagus (tenth) nerves also have sensory functions in the head and neck and their sensory cell bodies are in ganglia on the corresponding nerves. The central processes of the primary sensory neurons of all these three cranial nerves synapse with thalamic projection neurons in the spinal part of the trigeminal sensory nuclear complex. The trigeminal sensory nuclear complex is described more fully in Section 18.6.
The processes of trigeminal thalamic projection neurons decussate and ascend in the trigeminal lemniscus of the opposite side as shown in Figure 16.1. They synapse in the ventroposteromedial nucleus of the thalamus and are thus kept separate from neurons ascending in the spinothalamic tract. Tertiary neurons project from this nucleus to the head region of the primary sensory cortex through the posterior limb of the internal capsule.
16.2.2 Pain
In the description of the spinothalamic tract, you will have noticed that nociceptive stimuli take different routes and make different connections from thermal and tactile information. There are many interesting facets to the transmission of nociceptive stimuli and the perception of pain which require further consideration. After all, one of the main functions of dental practitioners is to put their patients at ease through a combination of good interpersonal skills and pain management when required. Pain is an entire study in itself and many aspects of pain are outside the scope of this book.
The pathways from nociceptors are shown in Figure 16.3. This figure shows nociceptors connecting to the cell bodies of primary sensory neurons by small diameter unmyelinated C processes and finely myelinated Aδ processes. Aδ processes conduct action potentials faster than unmyelinated C processes. It is now generally accepted that the Aδ processes conduct the initial noxious sensation that is interpreted as sharp pain (often referred to as first pain) while the unmyelinated processes convey the wave of duller, but intensely disagreeable, pain which follows (second pain).
The incoming Aδ and C axons of primary sensory neurons make separate connections in the spinal cord as shown in Figure 16.3B. Aδ axons connect with lamina I and lamina V thalamic projection neurons as described in the main description of the spinothalamic tract whereas C axons only synapse with lamina I neurons. Furthermore, the thalamic projection neurons responding to incoming nociceptive information carried by C axons form their own spinoreticular tract in the anterolateral aspect of the spinal cord (see Figure 16.2). This tract appears earlier in the course of evolution than the spinothalamic tract and is also referred to as the paleospinothalamic tract to distinguish it from the spinothalamic tract or neospinothalmic tract. As its name implies, the spinothalamic tract or spinoreticular tract does not terminate directly in the thalamus but, as illustrated in Figure 16.3, has intermediate synapses in the medullary and pontine reticular formation. The thalamic projection components of this tract may, therefore, be subdivided into a first secondary neuron from the spinal cord to the reticular formation and a second secondary neuron from the reticular formation to the thalamus. When they reach the thalamus, their axons synapse in the intralaminar nuclei.
Fig. 16.3 The spinoreticular pain pathways.
Nociceptors served by both Aδ and C processes have wide receptive fields so localization of the stimulus is poor. The posterior and intralaminar nuclei of the thalamus where these stimuli are ultimately directed do not project to the primary somatosensory cortex so they have no somatopical representation which compounds the problem of localization. However, the dual connections of Aδ processes to high threshold nociceptive and wide dynamic range tactile thalamic projection cells enable pain to be localized accurately in most cases.
Central projection of noxious stimuli
If the intralaminar and posterior nuclei of the thalamus do not project to the primary somatosensory cortex, where do they send their tertiary neurons to? Both sets of nuclei connect to widespread areas of the cerebral cortex, including the insula and basal ganglia which is not terribly instructive about the terminal destination of nociceptive pathways. It should also be borne in mind that only about 10% of thalamic neurons relay sensory information of all types to the cerebrum. The other 90% are concerned with controlling how much information and what specific information is allowed through the thalamus. A substantial amount of modulation of noxious stimuli probably takes places here and it is believed that general anaesthetics exert their actions at the thalamic level.
Returning to the destination of nociceptive pathways, recent research involving brain scanning of volunteers has identified the pain matrix referred to earlier. The pain matrix is the term used to refer to the different cortical areas that are activated by painful stimuli. As Figure 16.3 shows, these include the secondary somatosensory cortex situated just below the primary somatosensory cortex on the underside of the lateral fissure, the insula, the anterior cingulate gyrus, the cerebellum, and supplementary motor areas. Taken together with lower connections made from the reticular formation, the role of some of these projections starts to make sense when we think about our reactions to pain. The reticular formation connects to cardiovascular and respiratory centres that can produce changes in blood pressure and respiratory activity in response to pain. The anterior cingulate cortex is part of the limbic system (Section 15.4.2) which is involved in the emotional response to pain. Other connections are made from the reticular formation to the limbic system which is believed to be involved in learning; through experience, we learn which events produce a noxious stimulus and pain and thus how to avoid them. The connections to areas concerned with motor activity planning may be concerned with the responses required to withdraw from the stimulus. The secondary somatosensory cortex and the insula are particularly interesting; the emotional response to pain is reduced if these areas are inoperative but discrimination is preserved. It would appear that several brain structures are required to detect and localize nociceptive stimuli and to perceive these stimuli as pain with all the uncomfortable and emotional ramifications that accompany it. Some aspects of pain peculiar to toothache are outlined in Box 16.1.
Pain modulation
Pain can be modulated or abolished by a variety of pharmacological agents such as analgesics and local and general anaesthetics. Sedation is valuable in the treatment of many over-anxious patients, the root of the anxiety for many being the prospect of potentially painful dental treatment. Pain can be also physiologically modulated by numerous neural mechanisms within the CNS. Hypnotherapy and acupuncture are also used by some dental practitioners with some success for some patients and may stimulate some of these neural mechanisms. One mechanism we are all familiar with is ‘rubbing it better’ when we receive a painful knock. Aβ sensory nerves carrying discriminative stimuli (see Section 16.2.3) are stimulated by rubbing the painful area. As they enter the dorsal columns, they give off collateral branches which act at high threshold synapses in lamina I of the spinal cord to decrease the likelihood of neurotransmission from primary to thalamic projection neuron and, therefore, the upward conveyance of the stimulus. Pathways descending from the cortex and reticular formation follow the spinothalamic tracts in the reverse direction. These pathways modulate pain and are implicated in the mechanisms underlying acupuncture. Unfortunately, although these mechanisms are present and have been shown to work in experimental situations, no one has yet discovered how to harness them for effective pain relief.
Patients with toothache often have some difficulty identifying the tooth that is giving them pain. They can usually indicate whether it is an upper or lower tooth, whether it is on the right or left side of the mouth, and whether it is a front or back tooth. However, they are usually unable to identify the exact tooth more precisely unless there are other cues such as a large cavity that they can feel with their tongue. Teeth are only innervated by Aδ and C neurons and the dual mechanism operating through Aδ processes most likely operates in the trigeminal nuclei and beyond to give some localization. However, as shown diagrammatically in Figure 16.4, there is often branching of peripheral nerve processes to adjacent teeth and considerable convergence of primary sensory neurons on to thalamic projection neurons in the trigeminal sensory nuclear complex that confuses localization.
Fig. 16.4 Peripheral branching and central convergence of dental sensory nerves.
Pain is a complex, but clinically important, subject and many aspects of it are outside the scope of an anatomy book. You should consult textbooks of neurophysiology, neurophysiology, or some of the texts on clinical pain management for more information.
16.2.3 The dorsal column–medial lemniscus pathway
This is the pathway for discriminative touch, proprioception, and vibration and is illustrated in Figure 16.5. Follow this figure as you read the description below and compare it with Figure 16.1 to appreciate the major differences between the dorsal column and the spinothalamic systems. The two pathways differ/>
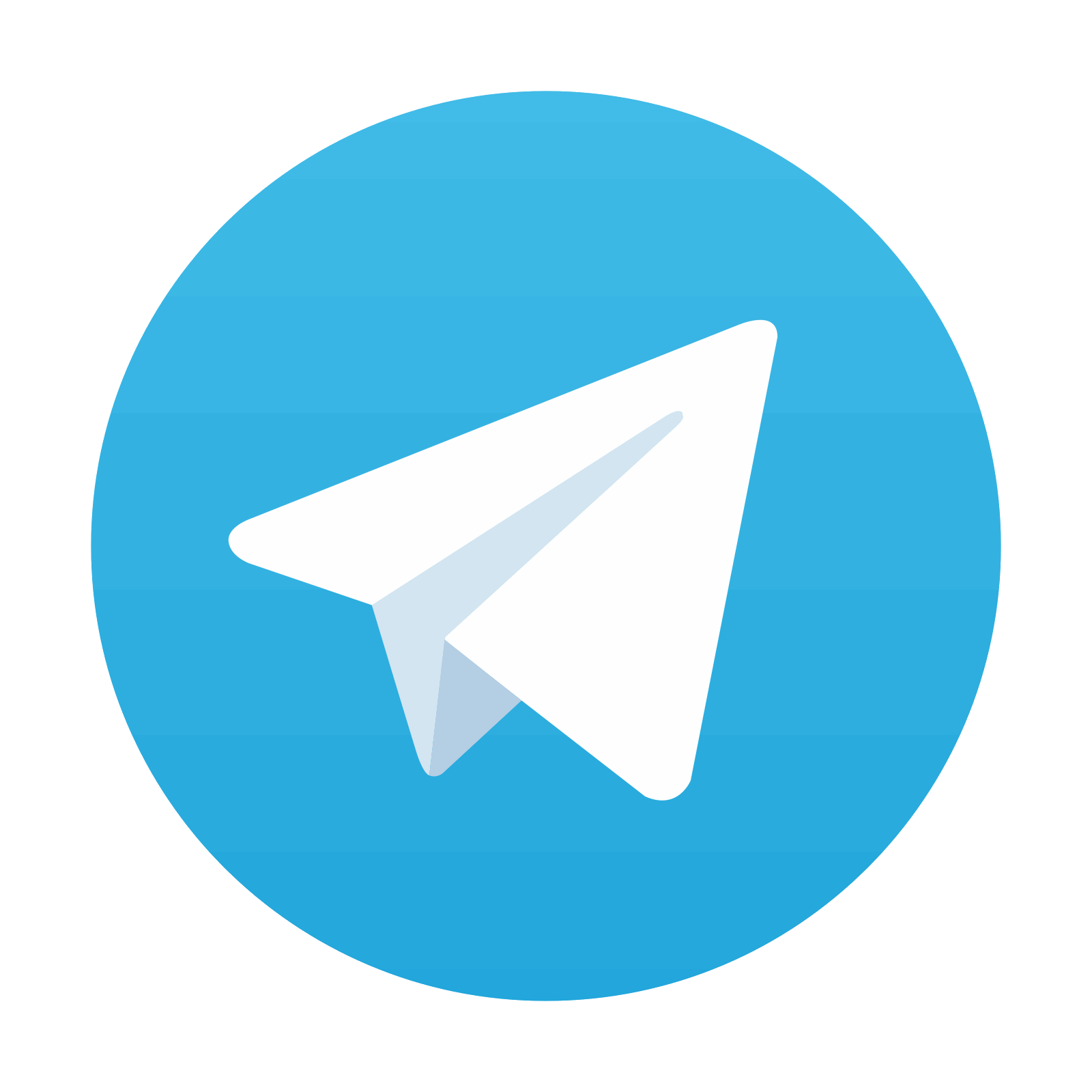
Stay updated, free dental videos. Join our Telegram channel

VIDEdental - Online dental courses
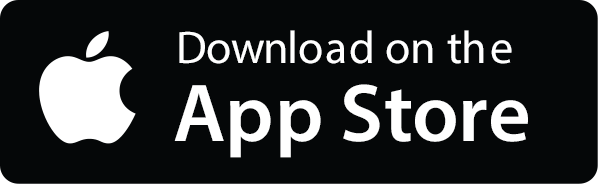
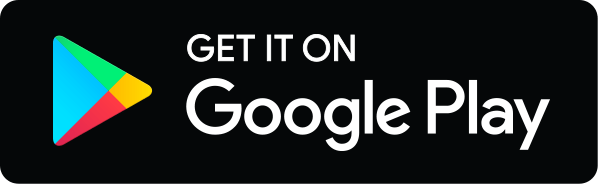