CHAPTER 10 Drugs Affecting Nicotinic Receptors*
The discovery of curare4 led to developments in two different directions: to drugs that affect transmission at nicotinic cholinergic receptors and to drugs that interfere with the mechanisms of skeletal muscle contraction. These two topics are the subjects of this chapter.
DRUGS AFFECTING GANGLIONIC TRANSMISSION
Ganglionic Transmission
Nicotinic receptors (see Chapter 1) play a crucial role in the transmission of autonomic impulses across the ganglionic synapse. As described in Chapter 5, acetylcholine (ACh) is the primary neurotransmitter at sympathetic and parasympathetic ganglia, where it is released by preganglionic neurons and stimulates postganglionic neurons by activating nicotinic NN receptors. Although it is sometimes convenient to think of autonomic ganglia as simple relay stations between the central nervous system (CNS) and effector tissues, the existence of other receptors and neurotransmitters within the ganglia indicates that some modulation of the primary nervous inputs may occur. It is also evident that transmission is not the same in sympathetic and parasympathetic ganglia even though NN receptors are the primary receptors in both cases.
Various pharmacologic and electrophysiologic studies on sympathetic ganglia have led to models of ganglionic transmission that involve at least four classes of receptors: cholinergic nicotinic, cholinergic muscarinic, α-adrenergic, and peptidergic.8 Muscarinic and peptidergic receptors mediate slow and late slow excitatory postsynaptic potentials, which seem to facilitate the transmission of high-frequency impulses through the primary nicotinic receptor pathway. Catecholamine-containing (dopamine or norepinephrine) interneurons have been proposed for sympathetic ganglia12 but are not found in parasympathetic ganglia.21 As shown in Figure 10-1, these interneurons may be stimulated by preganglionic muscarinic activity to release catecholamines that hyperpolarize the postganglionic neuron, producing an inhibitory postsynaptic potential. These secondary events of ganglionic transmission only modulate the primary depolarization, by making it more or less likely to occur. Conventional NN receptor antagonists can inhibit ganglionic transmission completely, but muscarinic antagonists, α-adrenergic antagonists, and peptidergic antagonists cannot do so.
Ganglionic Stimulating Drugs
Nicotine
Nicotine, as indicated in Chapter 8, is the principal psychoactive ingredient in tobacco products. As a selective depolarizing drug at nicotinic receptors, this alkaloid stimulates transmission at autonomic ganglia and at nicotinic synapses in the CNS. It also activates various sensory fibers equipped with nicotinic receptors, including mechanoreceptors in the lung, skin, mesentery, and tongue; nociceptive nerve endings; and chemoreceptors in the carotid body and aortic arch. Stimulation of nicotinic receptors in skeletal muscle is easily shown in the laboratory, but it is not evident normally in humans because initial stimulation is soon followed by inhibition at these nicotinic sites. Nicotine has a dual effect on ganglionic transmission—initial stimulation and subsequent depression (see later).
Acute overdose of nicotine causes nausea and vomiting, abdominal pain, dizziness and confusion, and muscular weakness. If untreated, death may ensue from cardiopulmonary collapse. Nevertheless, the primary health issues regarding nicotine stem from the chronic use of tobacco products. An increased incidence of cancer and cardiovascular and pulmonary disease has been well documented.20 In dentistry, tobacco use has been linked to oropharyngeal carcinoma, leukoplakia, acute and chronic periodontal disease, delayed wound healing, halitosis, and tooth staining.5
The only therapeutic use of nicotine is as an adjunct in tobacco cessation programs. Nicotine is administered in multiple forms (Table 10-1) to maintain pharmacologic concentrations of the alkaloid and to prevent tobacco cessation from triggering an acute withdrawal syndrome, which includes irritability, anxiety, sleep disturbances, and cognitive impairment. It also dissociates the self-administration of nicotine from the social, tactile, and oral and olfactory components of tobacco smoking, weakening the psychological link between satisfaction of the nicotine craving and the physical actions of tobacco use. The nicotine dose is reduced in a stepwise fashion over several months, during which time the patient ideally receives continued counseling and motivational assistance to remain abstinent.
Because of the deleterious effects of smoking and smokeless tobacco on oral health, the dentist is encouraged to participate actively in helping patients quit tobacco use.5 Such participation may include—in addition to prescribing a nicotine product—procedures to promote fresh breath and tooth bleaching to remove tobacco stains from teeth, which may provide additional positive psychological feedback to encourage abstinence from tobacco use.
Ganglionic Blockers
Between 1895 and 1926, numerous compounds having the generic structure shown in Figure 10-2 and termed methonium compounds were synthesized. In 1915, Burn and Dale described the ganglionic blocking action of tetraethylammonium. In the 1940s, an entire series of diiodide and dibromide derivatives of these methonium compounds were synthesized; in 1946, Acheson and Moe published a systematic and extensive pharmacologic study of tetraethylammonium. Interest in these drugs arose because they could be used as pharmacologic tools for exploring various aspects of autonomic pharmacology and because, at least at first, they offered the promise of being useful therapeutic agents in the treatment of hypertension, peptic ulcers, and other diseases that seemed to have an autonomic component and that had not yet yielded to therapeutic measures then available.
Classification
Pharmacologic effects
The pharmacology of the ganglionic blocking drugs is predictable because all parasympathetic and sympathetic ganglia are blocked by most of the available agents. Ganglia are not equally sensitive to the blocking drugs, however, and some effects are easier to block than others. The effects of ganglionic agents are profoundly influenced by the background tone; that is, the effect of blocking a ganglion is proportional to the rate of nerve transmission through that ganglion at any given time. If vascular tone is high, as it would be in a standing individual, the ganglionic blocking agents would produce a profound decrease in blood pressure, much greater than they would in a recumbent individual, in whom vascular tone would be lower. Finally, as is shown in Table 10-2, because these drugs block sympathetic and parasympathetic actions, the direction and magnitude of their effects are related to which autonomic division provides the dominant baseline control for a given organ.
TABLE 10-2 Usual Predominance of Sympathetic or Parasympathetic Tone at Various Effector Sites, with Consequent Effects of Autonomic Ganglionic Blockade
SITE | PREDOMINANT TONE | EFFECT OF GANGLIONIC BLOCKADE |
---|---|---|
Arterioles | Sympathetic (adrenergic) | Vasodilation, increased peripheral blood flow, hypotension |
Veins | Sympathetic (adrenergic) | Vasodilation, peripheral pooling of blood, decreased venous return, decreased cardiac output |
Heart | Parasympathetic (cholinergic) | Tachycardia |
Iris | Parasympathetic (cholinergic) | Mydriasis |
Ciliary muscle | Parasympathetic (cholinergic) | Cycloplegia |
Gastrointestinal tract | Parasympathetic (cholinergic) | Reduced tone and motility, constipation, decreased gastric and pancreatic secretions |
Urinary bladder | Parasympathetic (cholinergic) | Urinary retention |
Salivary glands | Parasympathetic (cholinergic) | Xerostomia |
Sweat glands | Sympathetic (cholinergic) | Anhidrosis |
Genital tract | Sympathetic and parasympathetic | Decreased stimulation |
From Taylor P: Agents acting at the neuromuscular junction and autonomic ganglia. In Brunton LL, Lazo JS, Parker KL, editors: Goodman & Gilman’s the pharmacological basis of therapeutics, ed 11, New York, 2006, McGraw-Hill.
DRUGS AFFECTING NEUROMUSCULAR TRANSMISSION
Neuromuscular Transmission
Nervous control of skeletal muscle contraction is mediated by ACh. In response to a motor neuron action potential, ACh is released from the terminal region of the nerve fiber. The transmitter diffuses across the junctional cleft and binds with the nicotinic NM receptor on the postjunctional membrane (end plate) of the muscle fiber. As with other nicotinic receptors the NM receptor has two binding sites for cationic ligands, and the binding of two molecules of ACh to the receptor brings about an increase in the cation permeability of the end plate membrane and a consequent depolarization (excitatory end plate potential) of the junctional region of the muscle fiber. Under normal conditions, the depolarization is sufficient to trigger an action potential in the electrically excitable muscle fiber membrane, and muscular contraction follows.10 Figure 10-3 shows the physiologic events that occur in a nerve, neuromuscular junction, and skeletal muscle that lead to contraction of muscle and indicates points along the pathway at which drugs can block these events.
Neuromuscular Junction Blockers
Nondepolarizing agents
Nondepolarizing, or competitive, neuromuscular blocking drugs include tubocurarine (d-tubocurarine) and several other benzylisoquinolines (e.g., atracurium, cisatracurium, and mivacurium); aminosteroids such as pancuronium, rocuronium, and vecuronium; and a few unrelated drugs.19 Tubocurarine, rocuronium, and vecuronium are monoquaternary amines with a second nitrogen that is partially ionized at physiologic pH; the other clinically available drugs are bisquaternary compounds. Commonly, these drugs incorporate two cationic nitrogen sites into a rigid molecular structure (Figure 10-4). The rank order of potency at the NM receptor correlates highly with the clinical dose needed to produce 50% twitch depression of the adductor muscle of the thumb (adductor pollicis).
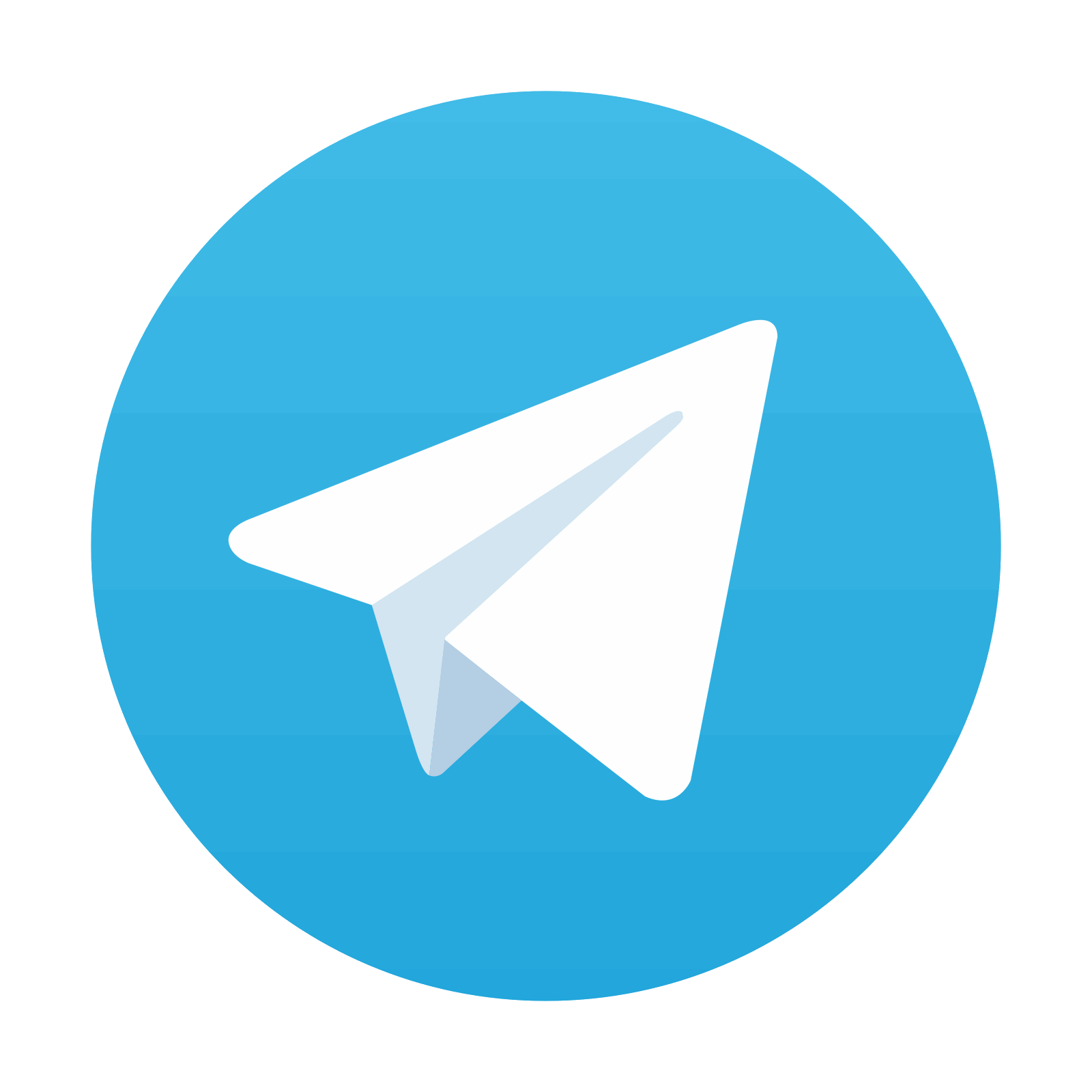
Stay updated, free dental videos. Join our Telegram channel

VIDEdental - Online dental courses
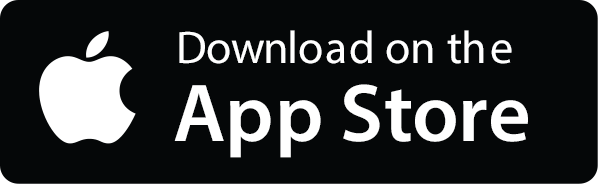
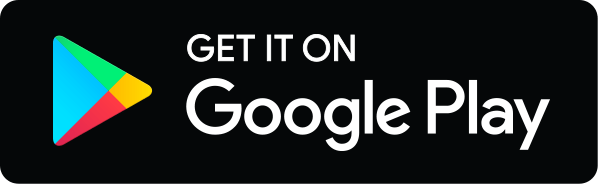