CHAPTER 19 Introduction to Antinociceptive Drugs
Pain has always been a barrier to dentistry, serving as a continuing motivation for the use of drugs to prevent, block, or attenuate pain in the perioperative period. Despite the efficacy of local anesthesia, many procedures can result in substantial postoperative discomfort and edema, limiting mouth opening for several days. Poorly controlled pain in the perioperative period also contributes to anxiety about future dental therapy, leading to postponed or canceled appointments.2,10,16 Effective control of orofacial pain facilitates the delivery of care, reduces anxiety about dentistry, and may even improve dental health by promoting preventive and routine dentistry as an alternative to general neglect with episodic care for acute problems. Emerging basic and clinical studies have shown that the use of antinociceptive drugs to attenuate perioperative pain can prevent the development of hyperalgesia manifesting as increased pain hours to days after a procedure. The availability of safe, effective antinociceptive drugs now permits effective prevention and management of perioperative pain for the mutual benefit of the patient and the dental practitioner.
The multiplicity of pain mechanisms and the difficulty of separating nociceptive processing from physiologic pain perception represent significant barriers to improving pain therapy. In addition to the molecular events associated with tissue injury, inflammation, development of sensitization, and activation of ascending and descending pathways, it is now recognized that gender and genetic factors also contribute to individual variation in pain perception, processing, and evaluation of nociceptive input. Because of this mechanistic complexity, use of analgesic drugs in fixed doses that have been validated in a relatively homogeneous patient sample may not result in effective therapy when used in a patient population with wide genetic diversity in pain processing and drug metabolism. Although individual variation in the human pain experience is traditionally explained by various factors such as cultural or psychological influences, more recent studies in animal and human pain models show phenotypic differences in pain sensitivity that may result from genetic factors.6,22,26,38,44 This genetic variation among patients, along with other sources of variation, provides a physiologic rationale for individualizing pain treatment.
The management of chronic pain has a long history of therapeutic misadventures, including the misuse of drugs for symptomatic relief of chronic orofacial pain. There is still no generally accepted agreement about the cause of chronic orofacial pain; its natural history; the need for aggressive treatment; and the effectiveness, safety, and indications for most clinical practices. Differences of opinion on these issues are often fostered by a lack of appreciation of the difference between clinical observations, which may form the basis for therapeutic innovation, and the need to verify the efficacy and safety of treatments in studies that control for factors that can mimic clinical success. Drug classes for the treatment of pain associated with temporomandibular joint (TMJ) disorders range from short-term treatment with nonsteroidal anti-inflammatory drugs (NSAIDs) and muscle relaxants for pain of presumed muscular origin to long-term administration of antidepressants and anticonvulsants for less well-characterized pain (see Chapter 23). The management of pain associated with TMJ disorders rests on the same principles that apply to the use of all drugs: demonstrated efficacy for the indication, an acceptable incidence of adverse reactions for the condition being treated, and safety when used in numerous patients for prolonged periods.
PATHWAYS OF OROFACIAL PAIN
Pain Transduction and Transmission in the Periphery
Noxious stimuli, which can produce tissue damage, are detected by the terminal endings of two major classes of nociceptive (pain-detecting) afferent nerve fibers (Figure 19-1). These nociceptors are distributed throughout the skin, oral mucosa, and tooth pulp. Aδ fibers are fast-conducting, lightly myelinated neurons responding primarily to noxious mechanical stimuli. Aδ fibers are thought to mediate the initial sensation of pain, which has a sharp perceptual quality. The second group of nociceptive fibers comprises C fibers, which are slowly conducting unmyelinated neurons that respond to thermal, mechanical, and chemical stimuli. C fibers likely mediate secondary pain, which occurs after the initial sharp, pricking pain and is generally described as having a dull, aching, or burning perceptual quality. There are approximately three to five times more C fibers than Aδ fibers.29 Other classes of cutaneous fibers have been described but are not as well characterized.
The detection of noxious stimuli in the orofacial region and the encoding of pain are conveyed primarily by nerves of the trigeminal system. The trigeminal nerve, or fifth cranial nerve, is the largest of the cranial nerves; its three branches (ophthalmic, maxillary, and mandibular branches) innervate most of the face and anterior scalp. The trigeminal nerve also innervates the mucous membranes and gingivae of the mouth; the teeth and jaws; the anterior two thirds of the tongue; the nasopharynx, nasal cavities, and sinuses; and a portion of the meninges. The facial (seventh cranial) nerve encodes pain from the skin of the mastoid region and the external auditory meatus; most of the sensory function of this nerve is involved in taste sensation. The glossopharyngeal (ninth cranial) nerve innervates the back of the tongue, tonsillar region, tympanic cavity, and antrum and oronasal portions of the pharynx. The vagus (tenth cranial) nerve innervates the larynx and parts of the pharynx, ear, and external auditory meatus. These cranial nerves provide the peripheral innervation necessary for the detection of orofacial and dental pain.19
Pain Modulation in the Brainstem
The Aδ and C fibers from the orofacial region transmit nociceptive signals primarily by the branches of the trigeminal nerve to the trigeminal nucleus caudalis; noxious information from additional regions is conveyed by other cranial nerves. Most clinical and laboratory data indicate that the nucleus caudalis is the principal brainstem relay site for trigeminal nociceptive information.36 The nucleus caudalis is located in the medulla; its laminated structure, types of cells, and function in processing pain signals are similar to the area on the dorsal aspect of the spinal cord termed the dorsal horn.
As previously stated, the small-diameter afferents carrying nociceptive information from the various craniofacial tissues predominantly terminate in laminae I, II, and V of the nucleus caudalis. By contrast, primary afferent A fibers conducting low-threshold mechanosensitive (tactile) information terminate primarily in the most rostral components of the trigeminal brainstem complex and in laminae III to VI of the nucleus caudalis. More recent studies have also revealed increases in immunocytochemical markers of neuronal activity in caudalis neurons after noxious stimulation of craniofacial tissues.36 Additionally, numerous studies using microelectrode recording have shown that many neurons in the nucleus caudalis are activated by cutaneous nociceptive input from the craniofacial region. For these reasons, the nucleus caudalis has been termed the medullary dorsal horn.37
Pain Perception and Modulation by the Cerebral Cortex
Two major projections carrying noxious information from the medullary and spinal dorsal horns are the trigeminothalamic tract and the spinothalamic tract. These tracts are composed of axons from the wide dynamic range and nociceptive neurons. These axons cross to the contralateral side of the medulla or spinal cord and ascend rostrally to the thalamus. From the thalamus, additional neurons convey this information to the cerebral cortex (see Figure 19-1).
The fourth component of the dorsal horn is composed of the terminal endings of descending neurons. These neurons form an important component of the endogenous pain modulatory system. Because the cerebral cortex is an important center for integrating all perceptual modalities together with higher functions, such as expectation and recall of previous events, it is not surprising that the cortex is involved in pain perception and response. Evidence suggests that the cerebral cortex is involved with the sensory discriminative aspect of pain and that it may serve as the most rostral activator of the endogenous analgesic system.19
PERIPHERAL MECHANISMS OF PAIN AND ANALGESIC DRUG ACTIONS
Activation of Nociceptors
Most noxious stimuli are transduced into electrical activity at the peripheral terminals of Aδ and C fibers by specific receptors or ion channels sensitive to heat, mechanical stimuli, protons, or cold. Ligand-gated channels expressed on nociceptive neurons include excitatory amino acid receptors, GABA receptors, nicotinic acetylcholine receptors, serotonergic (tryptaminergic or 5-hydroxytryptamine) receptors, and adenosine triphosphate P2X receptors. These receptors permit primary nociceptive neurons to respond to a wide range of mediators.28
Among the many channel types that modulate the passage of charged ions across cell membranes, Ca++ channels are particularly important in cellular homeostasis and activity, and the surface of each cell holds thousands of these channels that precisely control the timing and entry of Ca++. Small conformational changes cause these channels to open, allowing more than 10 million ions per second to flow through each channel. The opening of Ca++ channels is the crucial link between cell depolarization and Ca++ entry, which can result in local intracellular Ca++ concentrations up to 100 µmol/L. The subsequent binding of Ca++ to intracellular molecules can lead to many significant responses, including triggering of neurotransmitter release, the activation of second messenger systems, and Ca++ spikes (action potentials in which the depolarizing current is carried predominantly by Ca++).28
Transient receptor potential (TRP) channels are the vanguard of sensory systems, responding to temperature, touch, pain, osmolarity, and other stimuli. TRPV1, also known as the vanilloid receptor 1, is a Ca++-permeable channel that is opened by heat (>43° C) and decreased pH. TRPV1 contributes to acute thermal nociception and hyperalgesia after tissue injury. TRPV2, which is 50% identical to TRPV1 in primary structure, may mediate high-threshold (>52° C) noxious heat sensation, perhaps through lightly myelinated Aδ nociceptors. The ankyrin-like protein with transmembrane domains 1 channel is a Ca++-permeable, nonselective channel distinguished by approximately 14 amino-terminal ankyrin repeats. It is activated by noxious cold temperature (<15° C) but bears little similarity to the menthol-sensitive TRPV8 channel. It is found in a subset of nociceptive sensory dorsal root ganglion neurons in the company of capsaicin-sensitive TRPV1 channels.4
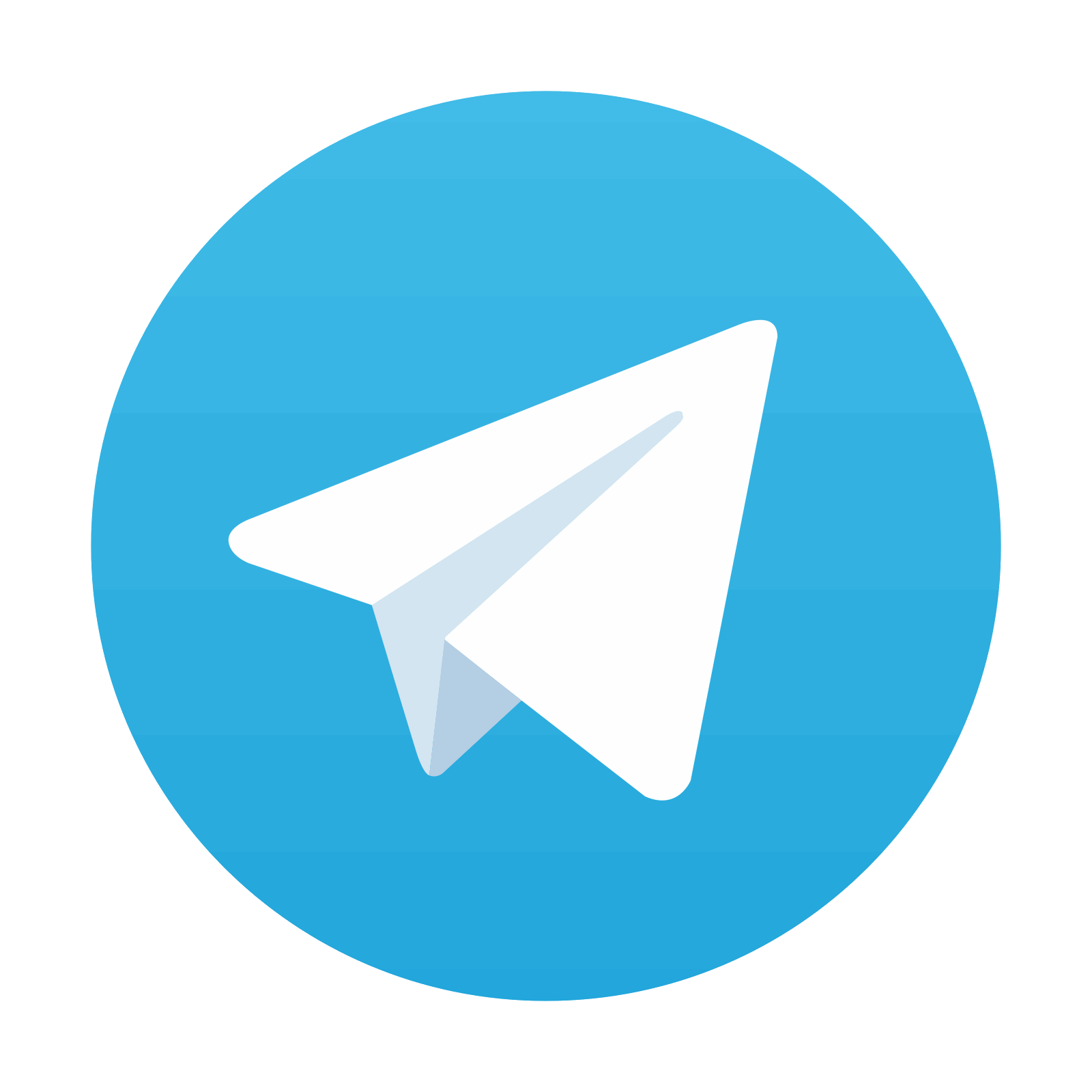
Stay updated, free dental videos. Join our Telegram channel

VIDEdental - Online dental courses
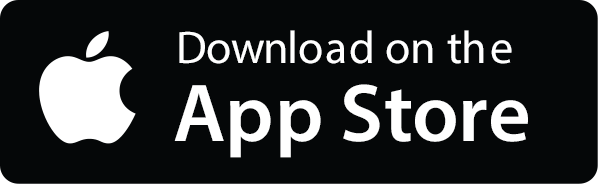
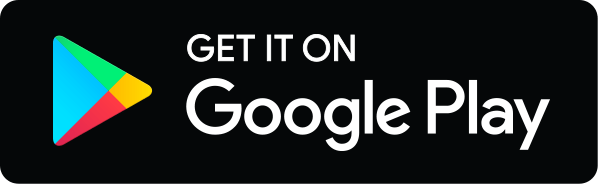