Introduction
The purpose of this study was to determine whether ultrashort echo time magnetic resonance imaging technology could be used to image teeth with orthodontic appliances in place.
Methods
High-resolution 3-T ultrashort echo time magnetic resonance imaging was performed on 60 extracted premolars with fixed ceramic orthodontic appliances (Clarity, 3M Unitek, Monrovia, Calif; Radiance, American Orthodontics, Sheboygan, Wis; and Ice, Ormco, Glendora, Calif). The teeth were collected from the orthodontic clinic at the University of Alabama at Birmingham, and the institutional review board of the University of Alabama at Birmingham approved the study. Linear measurements of tooth morphology and orthodontic bracket dimensions were acquired with calipers and compared with virtual digital magnetic resonance imaging. The spin echo and high spatial resolution multi-slice turbo spin echo were only used for visual comparison with the ultrashort echo time slices. Both the caliper and the ultrashort echo time magnetic resonance imaging measurements were highly reliable and accurate. Comparisons between the 2 methods showed no statistically significant differences in any bracket or tooth dimensions, with a P value of >0.05. In general, the differences in the values ranged from –0.01 to 0.06 mm. A visual evaluation scale was used to assess the quality of the ultrashort echo time magnetic resonance images when assessing the delineation of dental hard tissues.
Results
The visual evaluation scale of the images showed that enamel, dentin, pulp, and ceramic orthodontic appliances could be subjectively delineated at a high level with the ultrashort echo time magnetic resonance imaging.
Conclusions
The following conclusions can be drawn from this prospective study. Ceramic orthodontic appliances, without metal components, cause no dental image distortions and are readily visible on the ultrashort echo time magnetic resonance imaging scans. The measurements comparing linear tooth measurements with virtual digital magnetic resonance images demonstrated that magnetic resonance imaging has statistically and clinically significant accuracy on external tooth and bracket measurements. Visual evaluation of the images showed that enamel, dentin, pulp, and ceramic orthodontic appliances could be subjectively delineated at a high level with ultrashort echo time magnetic resonance imaging. Metallic slots in ceramic appliances cause severe image distortions. These distortions are localized and should not affect surrounding tissues in full-volume magnetic resonance imaging.
Imaging in orthodontics has evolved rapidly because of 3-dimensional imaging modalities. After their development in the 1990s, custom-built craniofacial machines began to appear on the market in the early 2000s. Other sophisticated imaging, with a variety of applications to the maxillofacial dental and skeletal regions, has followed. Orthodontists currently can image their patients with a multitude of imaging modalities, which include computed tomography, cone-beam computed tomography, cephalometric headfilms, panoramic films, intraoral and extraoral photography, soft-tissue laser scanning, stereo-photogrammetry, and magnetic resonance imaging.
Although there have been many developments and significant research focusing on imaging modalities for skeletal structures and soft-tissue morphology, the use of magnetic resonance imaging is minor in comparison. The use of nonionizing magnetic resonance imaging has not been considered an imaging modality for routine orthodontic diagnosis in part because of its poor performance in imaging dental and skeletal hard tissues. Traditional magnetic resonance imaging is better adapted for imaging the soft-tissue components, including temporomandibular joint pathology, tumors, muscles, and attachments. This might be attributed to the higher water content in these tissues. However, recent studies have been published exemplifying the use of magnetic resonance imaging in dental hard-tissue applications, showing quality images without the exposure to ionizing radiation.
Magnetic resonance imaging began with the ideas of an Irish physicist, Sir Joseph Larmor, and the first in-vivo report of medical magnetic resonance imaging was published in 1978 by Koutcher et al. The magnet is the largest and most important part in a magnetic resonance imaging system. Most magnetic resonance imaging machines are graded on the strength of the magnet, measured in tesla units (T). To put these numbers into perspective, 1 T is the equivalent of 20,000 times the magnetic field strength of Earth. Currently, magnetic resonance imaging units are in the range of 1.5 to 3 T for in-vivo applications, but the magnetic strength can be much higher for research.
Magnetic resonance imaging uses hydrogen atoms in the body to capture an image. By aligning individual atoms with magnetization, the machine applies a radio frequency pulse to depolarize the atoms, capturing the signal emitted on excitation with a receiver coil. A basic depiction of magnetic resonance imaging technology can be seen in Figure 1 . Hydrogen is abundant in soft tissues but is lacking in most hard tissues. Because the head and neck regions are primarily dental and skeletal hard tissues, magnetic resonance imaging has been perceived to have limited applications. A chance finding during research of 4-dimensional cardiac magnetic resonance imaging was the discovery of a magnetic resonance imaging sequence capable of hard-tissue differentiation. Multiple magnetic resonance imaging sequences exist and are selected for each diagnostic procedure to help enhance the contrast of the tissue being studied. Each sequence consists of different radio frequency pulses and associated mathematic gradients. Along with these parameters, the timing of signal capture also plays a critical role when imaging hard tissues. With further testing and optimization of the sequence, it was realized that the new technique called ultrashort echo time magnetic resonance could maximize hard-tissue capture and, hence, differentiate enamel from dentin and pulpal tissues. Standard magnetic resonance imaging sequences are not capable of such differentiation; they characterize enamel, dentin, and skeletal tissues equally.
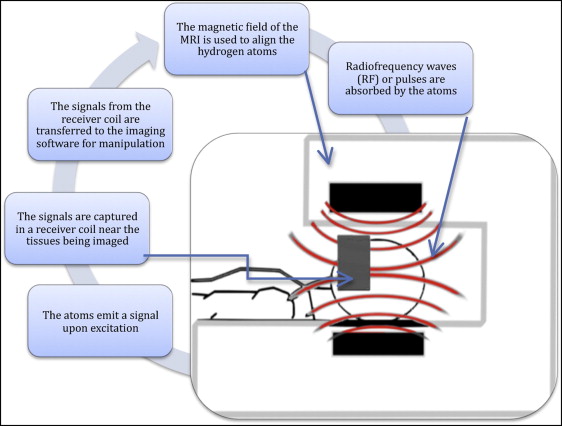
The application of magnetic resonance imaging in dentistry first appeared in 1981 in an article by van Luijk. Since then, the advancement of magnetic resonance imaging in dental applications has been relatively insignificant. Current applications include assessment of the temporomandibular joint and evaluation of soft-tissue tumors of the head and neck. Recent case reports have shown that magnetic resonance imaging technology in orthodontics can be used to take indirect impressions of the oral cavity, to assess impactions of the dentition, to detect root resorption, to detect demineralization and caries, and for 3-dimensional diagnosis and treatment planning. Unfortunately, the presence of orthodontic appliances has been shown to cause strong local image artifacts. Metallic materials can either amplify or weaken the magnetic field, depending on their magnetic properties. Metals, regardless of properties, cause an absence of spins in the image and thus result in signal voids where the image appears to be black. The metal also affects radio signals during image retrieval, potentially causing ring artifacts, shadowing, and geometric distortions. However, the use of ceramic appliances and the development of composite wires might prevent this concern in imaging in the future.
Magnetic resonance imaging has traditionally not been used clinically in dentistry and orthodontics because dental hard tissues have a relatively short time 2 (t2)/t2 relaxation time well below 200 μs. Bracher et al showed that ultrashort echo time has now made image acquisitions possible with echo times as low as a few microseconds. Enamel has a t2 of 70 μs, and dentin has a t2 of 150 μs in a 1.5-T field; with the use of ultrashort echo time, dental hard-tissue assessments with magnetic resonance imaging appear to be feasible.
Because magnetic resonance imaging is noninvasive and nondestructive, with no ionizing radiation, various craniofacial and dental magnetic resonance imaging applications and sequences have become a topic of interest and pioneering research over the last few years. Various forms of spin echo imaging have been performed on the dentition for caries research but can only achieve high-resolution visualization of moisture entering grossly decayed teeth. Unfortunately, it is also relatively slow, requiring capture times longer than an hour. The fast spin echo can capture the same image in about 10 minutes. Regrettably, these slower imaging techniques do not delineate the dental hard tissues, offering only an indirect appearance of the tooth surface. On the other hand, direct proton imaging sequences, also as known as solid-state imaging, have further developed the capability of magnetic resonance imaging to delineate dental hard tissues. Single-point imaging and stray-field imaging are 2 techniques capable of such delineation, but limitations, including long data collection times and high levels of acoustic noise associated with the scanning process, render them unsuitable for in-vivo applications.
Another imaging sequence with recent advancements in imaging dental hard tissues is sweep imaging with the Fourier transformation. Idiyatullin et al recently showed that with the sweep imaging with the Fourier transformation sequence, tissues with short t2 relaxation times such as dentin and enamel can be differentiated and that demineralized areas of the tissue appear as hyperintense areas in the image. Tymofiyeva et al suggested that sweep imaging with the Fourier transformation and the ultrashort echo time techniques show the most promising in-vivo results. Conversely, these direct proton-imaging techniques are inferior to the indirect technique at capturing the tooth surface. This would make a combination of direct and indirect imaging the most accurate technique for dental applications.
Accuracy of the minimum distance from the pulp and the carious lesion has also been demonstrated with magnetic resonance imaging. Tymofiyeva et al in separate studies demonstrated that carious detection was not limited to gross open decay but that it was also possible to directly use proton measurement with solid-state magnetic resonance imaging. Because magnetic resonance imaging shows the pulp, orthodontists can also have a better idea of pulpal-related pathology in treatment planning, including pulpal necrosis and internal resorption. Tymofiyeva et al also suggested the use of magnetic resonance imaging technology to image carious lesions under magnetic resonance imaging–compatible ceramic crowns; this could translate into the use of magnetic resonance imaging around an orthodontic bracket to visualize demineralization near magnetic resonance imaging–compatible orthodontic appliances. Reperfusion of transplanted teeth for orthodontic purposes might also be used for assessing the dental pulp in treatment.
In this study, we used both direct proton imaging and indirect imaging with a “signal providing” agarose gel. Using indirect methods of magnetic resonance imaging with intraoral contrast media and an intraoral prototype resonant frequency receiver coil, Tymofiyeva et al developed a magnetic resonance imaging–based technique for dental impressions. In the records process of orthodontics, alginate or polyvinyl siloxane impressions are a standard for obtaining a 3-dimensional model of the patient’s dentition; unfortunately, this impression process is a feared procedure for the patient. Some patients even have a strong enough gag reflex that impressions are removed from the records and diagnosis process altogether. Challenges include accurate enough impressions to fabricate orthodontic appliances. Fortunately, the literature has shown that, in vitro, the tooth surface can be reconstructed with a mean deviation less than 30 μm, and prosthodontic copings can be created with accuracy less than 100 μm. Although the impression material is replaced with a fluid in the patient’s mouth, patients seem to be more comfortable with the fluid because they are lying face down during the imaging process.
Magnetic resonance imaging has the potential to give orthodontists full-volume imaging with the elimination of ionizing radiation. This can be useful for evaluating growth, superimposing serial images to understand treatment changes with various mechanics, imaging the temporomandibular joint and related pathology, supervising eruption, and evaluating and determining impactions or ankylosis. Impacted canines can complicate orthodontic treatment and lengthen it. Localizing canines can be accomplished with standard periapical radiographs with tube shifts to locate canines with the buccal object rule as well as cone-beam computed tomography. Unfortunately, the ionizing radiation involved limits the diagnosis and supervision of the pediatric population we treat. Tymofiyeva et al found that magnetic resonance imaging can show a clear separation between impacted teeth and the surrounding tissues, and the positions and angulations of impacted teeth 3 dimensionally. Such a technology would allow orthodontists to periodically image a patient to monitor improvements in impaction and determine the appropriate timing for intervention. It has also been suggested that this technology would give adequate information to the orthodontist regarding root resorption and transposed or overlapping teeth.
It was the aim of this study to evaluate the applicability of 3-dimensional ultrashort echo time magnetic resonance imaging on dental morphology and its application to orthodontics with respect to appliance effects on morphology by an ex-vivo assessment of ceramic appliances on the natural dentition. No studies yet have investigated the accuracy of linear dental and orthodontic appliance measurements generated from a nonionizing imaging source compared with the true anatomic dimensions.
Material and methods
Sample teeth were collected from the orthodontic clinic at the University of Alabama at Birmingham. Ceramic brackets were donated by 3M Unitek (Monrovia, Calif), American Orthodontics (Sheboygan, Wis), and Ormco (Glendora, Calif). The sample included 60 premolars, and 15 Clarity (3M Unitek), 15 Ice (Ormco), and 15 Radiance (American Orthodontics) ceramic brackets.
The sample premolars had to meet the following criteria: no caries, no restorations, and closed apices.
The ceramic brackets had to meet the following criteria: designed for the same tooth and from the same manufacturer’s lot. High-resolution ultrashort echo time magnetic resonance imaging was performed on 60 extracted premolars with fixed ceramic orthodontic appliances. The maxillary right 18-slot premolar brackets included Clarity, Radiance, and Ice. Fifteen brackets from each manufacturer were bonded to the buccal surface of 15 teeth with Transbond XT (3M Unitek). All excess composite was removed before final curing. The teeth were then mounted in an agarose gel to replicate an oral-based medium that surrounded the surface of the dentition and the ceramic bracket, and to decrease air-surface interface artifacts. The agarose gel provided a good indirect image, or negative, of the external surface.
The magnetic resonance imaging images were completed on a 3-T whole-body magnetic resonance imaging system (Achieva; Phillips Medical Systems, Best, The Netherlands) equipped with gradient hardware capable of a maximum amplitude of 40 mT per meter with a maximum slew rate of 200 mT per millisecond. A prototypical 2 × 2 element carotid artery coil sized 120 × 50 mm (Phillips Research Europe, Hamburg, Germany) was used to acquire the data. Before this study, an optimization-calibration procedure of the 3-dimensional ultrashort echo time technique was carried out. A detailed overview of the magnetic resonance imaging acquisition parameters has previously been given. After a fast initial survey in the midsagittal direction along the long axis of the tooth, 3 high-resolution surveys were acquired. Each survey was planned in a sagittal orientation along the tooth’s long axis. The diagnostic scans were a standard spin echo, a high spatial resolution multi-slice turbo spin echo (TSE) acquisition, and a 3-dimensional ultrashort echo time scan. The series of scans of the tooth and bracket samples were completed in 1 hour and 15 minutes. To reduce research use of the clinical magnetic resonance imaging scanner, 2 samples were mounted in each sample vial ( Fig 2 ).
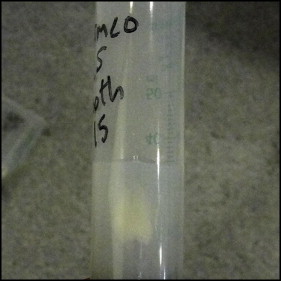
The magnetic resonance imaging images were analyzed on imaging software (OsiriX; Pixmeo, Geneva, Switzerland). The 3-dimensional magnetic resonance imaging data were multiplanar reformatted along the long axis of the tooth with the other axes from buccal to lingual, and mesial to distal. The same clinician (R.J.C.), who was trained on the software, made all measurements, and measurements were repeated 7 days later for intraexaminer reliability. The brackets were measured from mesial to distal, and occlusal to apical at the greatest diameter parallel and perpendicular to the occlusal plane and recorded to the nearest 0.01 mm as shown in Figure 3 . The teeth were measured from mesial to distal, buccal to lingual, and occlusal to apical at the greatest diameter parallel or perpendicular to the occlusal plane to the nearest 0.01 mm.
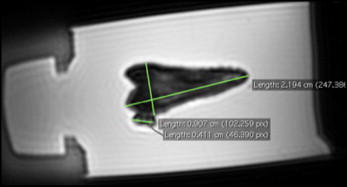
Real measurements of tooth and orthodontic appliance morphologies were made with digital calipers (500-196-20, Absolute Digimatic; Mitutoyo, Aurora, Ill) to the nearest 0.01 mm, measuring at the largest diameter as shown in Figure 4 . These linear measurements were compared with the “virtual” digital magnetic resonance imaging images.
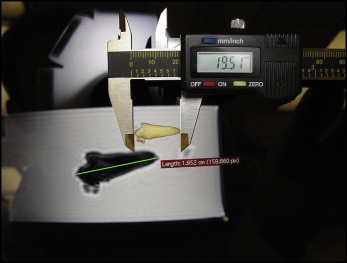
Statistical analysis
Reliability and accuracy were assessed by using intraclass correlations and paired Student t tests. P ≤0.05 was used to assign statistical significance.
Results
The imaging protocol was completed on all 60 sample teeth. Severe localized distortions from the metallic slots in ceramic brackets were observed in all 3 scans, including the standard spin echo, high spatial resolution multi-slice TSE acquisition, and 3-dimensional ultrashort echo scan. These distortion artifacts were limited to the coronal half of the tooth and would be localized in an in-vivo application. Therefore, the ceramic brackets with metallic slots from 3M Unitek had to be excluded from the study.
Real measurements of the orthodontic appliance and tooth morphologies compared with nonreal magnetic resonance imaging images were dimensionally accurate within 0.1 mm in all 3 planes of space. These results are shown in Tables I and II . Paired Student t tests were performed to compare the magnetic resonance imaging digital images with the linear caliper measurements in the mesial-distal and occlusal-gingival measurements of the brackets and the mesial-distal, buccal-lingual, and occlusal-gingival measurements of the teeth. Comparisons between the 2 methods showed no statistically significant differences in any bracket or tooth dimension ( P >0.05). The ultrashort echo time magnetic resonance imaging values tended to slightly overestimate the real values of the bracket measurements. These results are shown in Tables I and II . Comparisons of the linear measurements of the tooth samples with the virtual digital measurements can be seen in Table III . Therefore, the teeth and brackets are dimensionally accurate with the ultrashort echo time magnetic resonance imaging technology when compared with real measurements.
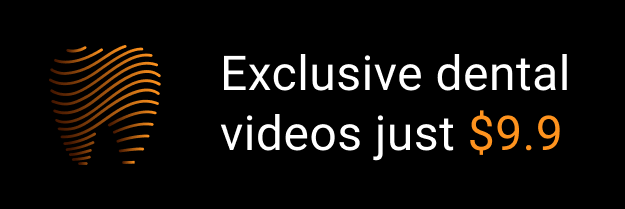