The transfer of materials across epithelial cell layers – secretion and absorption
Publisher Summary
This chapter describes the transfer of materials across epithelial cell layers—secretion and absorption. The greater volume of the extravascular extracellular fluid is separated from the blood plasma by the endothelial walls of the capillaries only. The cerebrospinal fluid fills the ventricles of the brain and the central canal of the spinal cord. The brain has its own tissue fluid in equilibrium with the cerebrospinal fluid but it has no lymphatic drainage as such. The ventricles communicate with each other and also with the space between the inner two of the membranes covering the brain, the pia mater and the arachnoid mater. Because the cerebrospinal fluid is formed continuously, there must be some route by which it can drain back into the bloodstream after its circulation. Many joints, including the temporomandibular joint, are enclosed in a fibrous capsule lined by an inner membrane termed the synovium or synovial membrane. Single epithelial cells or aggregations, often into distinctive structures, are involved in the production of specialized fluids, termed secretions, which are transported to the body surface. The organic components of most secretions are either mucins or enzymes.
Movement of substances across cell membranes has so far been considered in the context of single cells or in relation to exchanges taking place between the major compartments of the body fluids. However, in epithelial cell layers, movements across the cell membrane may be become localised and specific, so that a net flow of particular substances, including water, occurs in a particular direction across a whole cell layer. This polarisation and modification of the activity of the cells can be seen in the formation of the transcellular fluids in their epithelium-lined compartments; in the production of the exocrine secretions (fluids of controlled composition directed by epithelial cells towards the surface of the body), and endocrine secretions (biochemical agents passed into the bloodstream to be carried to distant target organs); in the formation of urine in the kidney, as substances are added or removed; and in the absorption of selected nutrients, salts, and water across the walls of the intestinal tract.
Transcellular fluids
Cerebrospinal fluid (CSF)
The cerebrospinal fluid fills the ventricles of the brain and the central canal of the spinal cord. The brain has its own tissue fluid in equilibrium with the cerebrospinal fluid, but it has no lymphatic drainage as such (Fig. 2.1). Since the activities of nerve cells are critically dependent upon the composition of the fluid around them, this must be very carefully controlled. The relatively large volume of the cerebrospinal fluid, and the fact that no part of the brain is more than 2 cm away from it, render it a very effective stabiliser of the external environment of the brain cells, in addition to its other important role of providing a protective cushion of fluid between the brain and the skull.
Figure 2.1 Diagram to show the relationships between the fluid compartments of the brain. The arrows indicate the direction of fluid secretion in the choroid plexuses, and of fluid reabsorption in the arachnoid granulations. The bidirectional arrows indicate exchange between compartments.
The ventricles communicate with each other and also with the space between the inner two of the membranes covering the brain, the pia mater and the arachnoid mater (Fig. 2.2). This space expands into the so-called cisternae where the pia mater extends into the grooves in the surface of the brain (the sulci) whilst the arachnoid mater bridges over them. The foramen of Magendie in the roof of the fourth ventricle communicates with the cisterna magna, and the two foramina of Luschka in the lateral walls of the fourth ventricle communicate with the cisternae at the base of the brain between the pons and the medulla oblongata. Cerebrospinal fluid is formed continuously from the blood plasma by special structures in the ventricles called the choroid plexuses. It passes through the foramina to circulate through the subarachnoid spaces of the spine, the cerebrum and the cerebellum, and is finally reabsorbed back into the bloodstream through the arachnoid granulations in the large venous sinuses of the dura mater (Fig. 2.3). The total volume of the fluid in man is around 140 ml, the ventricles themselves containing some 28 ml.
Figure 2.2 Arachnoid granulations in the venous sinuses of the brain. Arrows indicate the direction of flow of cerebrospinal fluid.
Figure 2.3 Cerebrospinal fluid: location and circulation: (a) the ventricles of the brain and their connections; (b) the fluid around the brain, in the spinal canal and in the ventricles. The meninges and the arachnoid granulations are exaggerated to make them visible.
Formation
The composition of cerebrospinal fluid (Table 2.1) differs from that of interstitial fluid – sodium and chloride concentrations are higher and potassium and hydrogen carbonate concentrations lower. Equally the fluid is not an ultrafiltrate of plasma – the concentrations do not match with the distribution which might be expected from a Gibbs Donnan equilibrium. Study of the ependymal cells shows that they restrict simple diffusion and they actively transport ions in both directions. Sodium and chloride are actively transported into the cerebrospinal fluid and it is this which generates the osmotic gradient necessary for the formation of the fluid by the movement of water. Calcium concentrations are lower than in plasma because very little protein is able to pass from the plasma into the cerebrospinal fluid and about half of the calcium in plasma is protein-bound. Glucose is known to be transported into the fluid but is used rapidly by the brain cells and so its final concentration is lower than that in plasma. Urea is able to diffuse and balance across the ependymal membrane. The halides, with the exception of chloride, are actively transported out of the fluid. The low concentration of potassium probably reflects its relative exclusion due to the high concentration of positive sodium ions.
Resorption
Since the cerebrospinal fluid is formed continuously there must be some route by which it can drain back into the bloodstream after its circulation. This is provided by the arachnoid granulations in the venous sinuses of the brain (Fig. 2.2), whose villi enclose tiny canals through which the cerebrospinal fluid flows back to the blood. The cells of the villi are so constituted as to operate in a valve-like manner and allow the cerebrospinal fluid to pass into the sinus when its pressure exceeds the venous pressure there.
The fluids of the eye
The eye contains two major fluids, the aqueous humour around and in front of the lens, and the vitreous humour behind the lens (Fig. 2.4). The vitreous humour, although gelatinous in nature, contains some 99% water and this solution is in equilibrium with the aqueous humour. The total volume of the vitreous humour is just under 4 ml whilst that of the aqueous humour is about 0.5 ml, of which 0.3 ml are in the anterior chamber. The aqueous humour occupies the space between the vitreous humour and the corneal epithelium; the space is divided into an anterior and a posterior part or chamber by the iris. The function of the aqueous humour is to provide nutrition to the non-vascular lens, which lies in the posterior chamber. The lens is suspended by the ciliary body, made up of the ciliary muscle and connective tissue, with a rich blood supply. The iris also has a rich blood supply. The posterior surface of the iris and the ciliary body are covered by a specialised epithelial layer which actively transports sodium ions, aminoacids and glucose into the posterior chamber. The transport of sodium ions into the posterior chamber produces a gradient of charge along which chloride ions move and a gradient of osmotic activity along which water follows. Like the ependyma, this membrane actively removes other halides from the fluid formed. The vitreous humour is not separately formed but maintains its composition by equilibrating with the aqueous humour. The aqueous humour drains back into the bloodstream through the anterior surface of the iris, which has no covering epithelial layer, and through the canal of Schlemm. Some 2.75 μl/min are formed, and this gives an internal pressure in the eye of around 3.3 kPa. The composition of the aqueous fluid is given in Table 2.2; that of the vitreous humour differs slightly because the cells around it secrete hyaluronate. This glycosaminoglycan, with a molecular weight of 2-10 × 106, exists as long polymeric chains which, because of their extensive overlapping, form very viscous solutions. It is the hyaluronate content which gives vitreous humour its viscosity.
Synovial fluid
Many joints, including the temporomandibular joint, are enclosed in a fibrous capsule lined by an inner membrane termed the synovium, or synovial membrane (Fig. 2.5). The space within the capsule, around the joint surfaces, is filled by the synovial fluid, whose main function is that of a lubricant for the articular surfaces. The outer capsule of the joint is made up of collagen fibres with some blood vessels. There may be some fat cells in the deeper layers. The lining cells are somewhat variable both in number and morphology, looking rather like fibroblasts, but they are larger, stain more readily with basophil dyes and have many vacuoles in the cytoplasm. There are two main types, one with an abundant Golgi system and many vacuoles, the other with an abundant rough endoplasmic reticulum, fewer vacuoles and a much less extensive Golgi system. The first type contains pinocytotic vesicles; they have fine branched filaments in the cytoplasm; and cell processes extend from them into the cavity. They appear to function, at least to some extent, as macrophages. The second type resembles those mature cells of cartilage and bone which are actively engaged in protein secretion. There is no evidence of tight junctions or of a basement membrane; so it would appear that the synovial fluid constituents reach the fluid by intercellular routes and not by active transport.
The lining cells synthesise hyaluronate and secrete it into the synovial fluid, rendering it highly viscous. The concentrations of ions are similar to those in plasma (Table 2.3). The protein content of synovial fluid is less than that of plasma and all the plasma proteins present are small, suggesting that the tissue fluid has been further filtered. In addition to the plasma proteins there are some proteins probably derived from bone or cartilage. Lymphatic vessels are present in the capsule and it has been shown that there is reabsorption of protein from the synovial cavity, with a flux of about 40% of the protein in 24h. The fluid also contains a few cells, mainly monocytes, although in the capsule itself the predominant extravascular white cell is the mast cell.
Exocrine secretions
Secretion of organic components
The organic components of most secretions are either mucins or enzymes. The lubricant secretion referred to as mucus is actually a mixture of glycoproteins, with a small fluid component. The secretory products of a secretory cell are synthesised within the cell and enclosed in vesicles (Fig. 2.6). A distinction is made between eccrine (or merocrine) secretion, in which the contents of the vesicle are lost when it fuses with the surface membrane and opens to the exterior; and apocrine secretion, in which the whole vesicle is lost, including its bounding membrane. This distinction may not be as absolute as was once thought. Sometimes whole cells appear to disintegrate and lose their contents: this is termed holocrine secretion.
Figure 2.6 Typical secretory cells: (a) a protein-secreting cell; (b) a cell secreting glycoproteins. Note the extensive basal endoplasmic reticulum in the protein-secreting cell, and the secretion-filled vesicles in both.
Enzymes and the protein part of mucoproteins are synthesised by the ribosomes on the rough endoplasmic reticulum; a characteristic of enzyme-secreting cells is the extensive endoplasmic reticulum at the base of the cell (Fig. 2.6). The proteins then enter the cisternae of the rough endoplasmic reticulum and from there pass to the Golgi saccules proximal to the nucleus (Fig. 2.7). The Golgi apparatus adds any necessary carbohydrate to the protein molecules, and sends them on in vesicles from the distal saccules to pass to the cell surface. It is thought that microtubules define a path from the Golgi apparatus to the particular face of the cell membrane from which exocytosis will occur: however, in most secretory cells there is an accumulation of secretory vacuoles or vesicles beneath the membrane. This often forces the nucleus to the basal side of the cell. The processes of synthesis and storage usually take place continuously and are divorced from the process of secretion, which occurs only in response to a secretory stimulus. The stimulus to secretion is either a neurotransmitter substance, released as a result of a nerve impulse, or a hormone. Such stimuli can cause the intracellular production of a second messenger substance, although the most characteristic and important effect may be the release of calcium ions in the cell. The calcium ions render the vesicles able to fuse with the plasma membrane, possibly by binding to the vesicle membrane and changing its charge. The vesicles then rupture, and expel their protein and fluid contents to the external environment. The vesicle may reform and pass back into the cell; more usually it simply extends the membrane temporarily, and then, at a later time, pinocytotic vesicles are formed and the membrane reverts to its former size. One unusual gland in this respect is the mammary gland: its secreting cells maintain a fairly constant perimeter because the increase due to the eccrine secretion of casein is balanced by the loss of membrane due to the apocrine secretion of milk fat globules.
Figure 2.7 The process of secretion of large molecules. After synthesis by the rough endoplasmic reticulum, the secretory product (x) is packaged by the Golgi apparatus into vesicles and lost from the cell by exocytosis. The membrane material of the vesicles is recycled.
The secretion of organic components is seen in the isolated mucous cells of the upper respiratory tract, in the mucous cell aggregations of the digestive tract, and in the individual cells of the tubular glands of the stomach (Fig. 2.8). The enzyme-secreting cells of the salivary and pancreatic glands behave similarly, despite being units of much more complex structures.
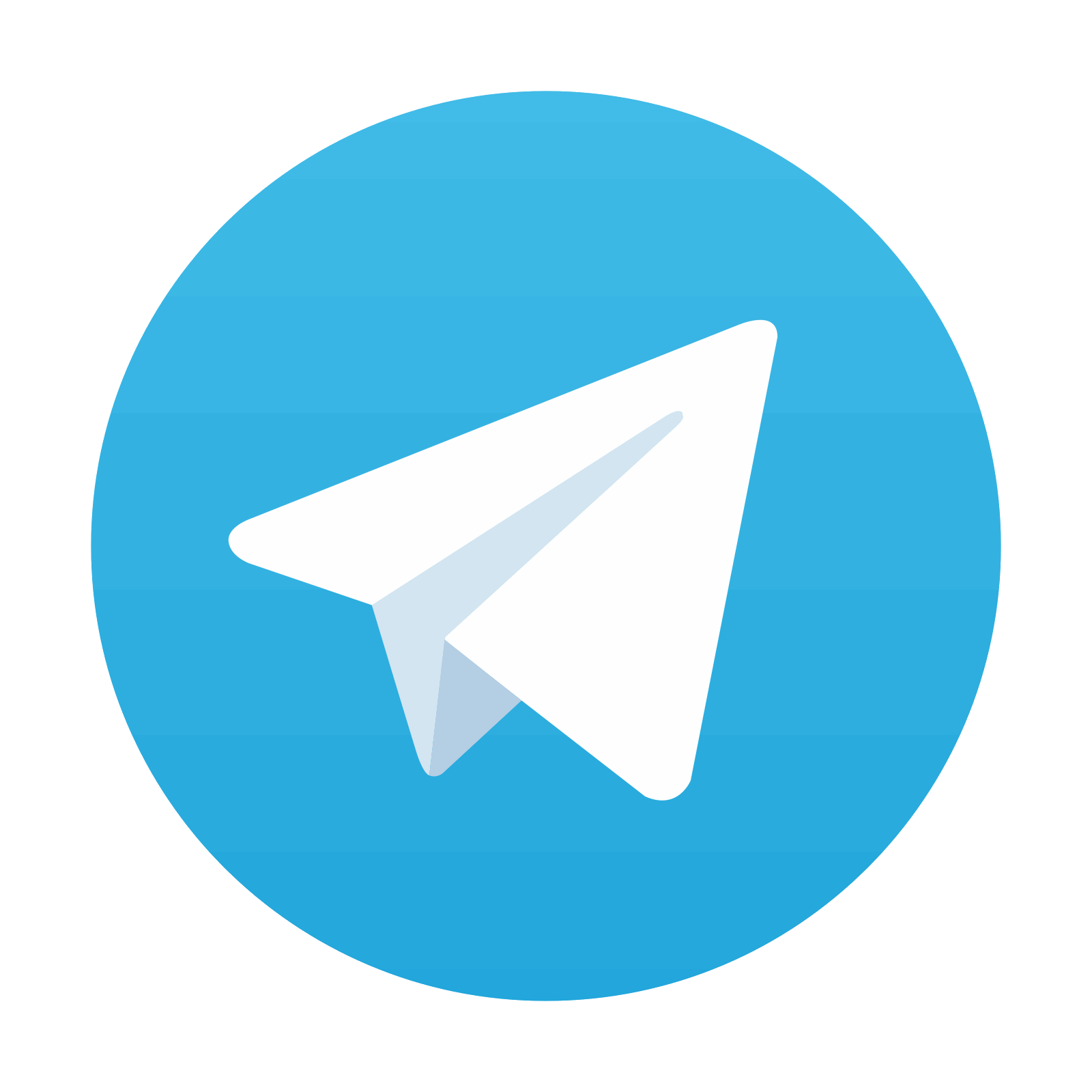
Stay updated, free dental videos. Join our Telegram channel

VIDEdental - Online dental courses
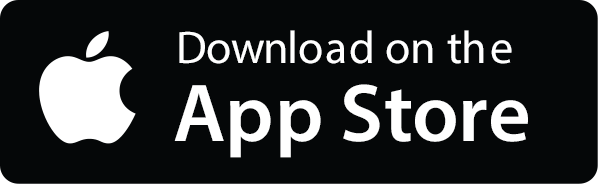
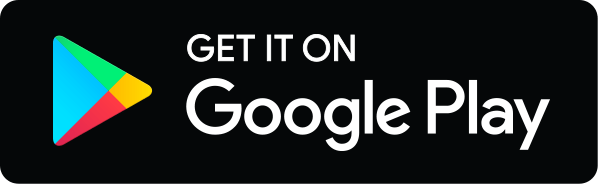