Fig. 1.1
Implants at time of placement
1.6 Does Previously Treated Periodontitis Influence Peri-implantitis?
Patients who have been previously treated for periodontitis and who are now receiving implants might be considered at increased risk of periodontitis compared with periodontally healthy patients. The bacteria associated with peri-implantitis are also present in periodontitis (Mombelli et al. 1987), and the teeth are the main reservoir of periodontal pathogens that subsequently colonize implants. It takes less than 2 weeks for the pathogenic bacteria associated with periodontitis to colonize the gingival sulci around implants when they are freshly uncovered for abutment connection (Quirynen et al. 2006). This occurs irrespective of the depth of the implant pockets or whether the patient rinses with chlorhexidine. But finding small numbers of potentially pathogenic bacteria within an implant pocket may have no clinical significance. It is the quantity of these periodontal pathogens not just their presence in the peri-implant sulcus that is clinically important. In a systematic review, it was concluded that patients with a history of treated periodontitis may suffer more implant loss, although caution was expressed about the high bias and lack of accounting for factors such as smoking in some of the studies (Ong et al. 2008). It is essential that patients with a previous history of treated periodontitis undergo comprehensive periodontal therapy following implant therapy and that implants with plaque-retentive or rough surfaces are avoided (Quirynen et al. 2007).
1.7 Do Systemic Factors Modify the Host Response to Plaque Around Implants?
Diabetes is known to exacerbate pre-existing periodontal disease and may be a risk factor in implant success. After 1 year, the failure rate of implants in diabetic patients is moderately high at 7.3 % (Shernoff et al. 1994), but the failures in this study were not controlled for type of implant, patient’s smoking status or other confounding factors. The success rate of implant treatment in diabetic patients can be improved by good glycaemic control with normalized serum glucose levels and glycated hemoglobin (or HbA1c) maintained at about 7 % (Marchand et al. 2012). A study of rough-surfaced implants placed by periodontology residents also showed that diabetes was associated with a moderately increased risk of failure (odds ratio of 2.59) (Zupnik et al. 2011). The heterogeneity of studies (with either short follow-up periods (Dowell et al. 2007) or small numbers of study participants (Farzad et al. 2002; Lambert et al. 2000; Cavalcanti et al. 2011)) has not permitted a meta-analysis (Bornstein et al. 2009).
Parafunctional habits and smoking are also known to exacerbate pre-existing periodontal disease and are a well-recognized risk factor in implant therapy. In a retrospective study undertaken 5 years after implant placement, 5.5 % of implants were found to have failed in 75 smokers, whereas only 2.9 % of implants failed in non-smokers (OR = 1.72) (Cavalcanti et al. 2011). Smoking is not related to a breakdown of osseointegration immediately following surgery but is rather associated with failure after uncovering the implant (Lambert et al. 2000). This would suggest that the deleterious outcome of smoking was the result of a toxic local effect, e.g. vasoconstriction, and therefore could be prevented by smoking cessation. The responsible agents may be the carbon monoxide, nicotine or other constituents of the particulate matter. A study involving 64 patients with at least 5 years of follow-up since they received their implants found greater marginal bone loss in smokers than non-smokers (Levin et al. 2008). The researcher examining the radiographs was unaware of the smoking status of the patients; however, there were only five who had smoked previously and six current smokers.
1.8 What Is the Role of Local Overload in Implant Failure?
Late implant failure is often associated with overload. Stress occurs to the upper part of the implant when lateral loads are applied (Klineberg et al. 2012). Many authors therefore recommend an occlusal scheme where loads are applied through the centre of the tooth as the patient closes into the intercuspal position. When finite element analysis was used to model a 5-unit fixed partial denture borne by 3 implants, it was found that group function produced excessive stresses compared with canine guidance (Gore and Evlioglu 2012). Therefore, the same occlusal principles described for fixed partial dentures involving teeth should be used for implant restorations.
1.8.1 How Do Osteocytes Respond to Loading?
At the cellular level, osteocytes may perform a mechanosensor role (“mechanostat”) and respond to strain in the bone by releasing cytokines. In this way the skeleton is able to adapt the bone form and size to its function in the most efficient manner. According to this hypothesis, low micro-strain is associated with bone resorption, whereas high micro-strain causes increased bone mass (Frost 1987). However, evidence to support this hypothesis has described raised cytokine levels in tissues surrounding failed knee prostheses, but this finding is related to the metal and polyethylene debris, and not to low-strain loading (Chiba et al. 1994). Based on in vitro data, one radical hypothesis suggested that the chemokine interleukin-8 is released into the gingival tissues by the contact of blood with the titanium implant surface and stimulates an additional inflammatory mucosal reaction which is independent of bacteria (Quabius et al. 2012). A study found no difference in the levels of interleukin-8 in the implant crevicular fluid of patients with peri-implantitis compared to those with healthy implants (Severino et al. 2011), but another study found increased levels (Luo et al. 2011). So far, research has not elucidated the mechanism which determines whether bone loss or formation occurs with applied load, and pharmacological manipulation of the process cannot therefore be used.
1.8.2 The Bruxist Patient: Are Additional Precautions Necessary?
The clinical experience of many clinicians has made them cautious in implant treatment planning for patients with severe bruxism. Whether the excessive occlusal load directly or indirectly causes bone loss and implant failure is controversial. Animal studies have shown that excessive dynamic loads can cause marginal bone resorption and crater-like defects around tibial implants (Duyck et al. 2001), whereas static loads had no effect. The loads were applied 6 weeks after implant insertion, and with the implant site in a healing phase, this may have influenced the response to applied load. However, the clinical implication of this result is that the static loads introduced as a result of small casting errors may be tolerated, whereas excessive dynamic loads in chewing cause bone micro-fractures. Cyclic loading of the radius of dogs with excessive loads has been shown to cause bone micro-fractures that were significantly associated with resorption spaces (Burr et al. 1985). Micro-damage of bone can therefore initiate resorption.
1.9 The Treatment of Compromised Teeth and Implants
Some authors recommend that periodontally compromised teeth be replaced with implants, but the alternative view is that these teeth should only be extracted as a last resort (Donos et al. 2012). Advanced periodontal disease can be successfully treated provided the patient can perform good plaque control. With regular maintenance care, extensive fixed cross-arch bridgework supported by a healthy but reduced amount of periodontal support (about 20 %) has been shown to be highly successful (Laurell et al. 1991). In a systematic review, meta-analysis showed that 92.9 % (95 % CI: 89.5–95.3 %) of fixed dental prostheses with a reduced but healthy periodontium survived (Lulic et al. 2007). This survival rate is similar to that of implant-supported prosthesis. In those situations requiring implant placement where teeth present a “hopeless” prognosis and require extraction as a result of periodontal disease, a vigorous maintenance programme should be instigated to prevent peri-implantitis. This is particularly so if the patient’s host response to plaque-induced disease makes them susceptible (see Chap. 4 which discusses the genetic background of implant failure).
A clear, universally accepted definition of peri-implantitis is necessary to allow comparisons between studies and to assess its prevalence. Peri-implant diseases are partially plaque-associated and so many of the classification systems used have similarities to those of gingivitis and periodontitis. A classification system for peri-implantitis has been proposed based on probing depth, the degree of bone loss around the implant and the presence of bleeding on probing (Froum and Rosen 2012). Implants can be lost with minimal evidence of associated gingival inflammation (Fig. 1.2).
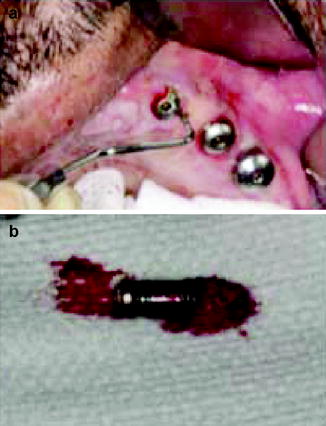
Fig. 1.2
(a) Deep pocket surrounding the implant despite the absence of gingival inflammation. (b) The implant required removal
Clinical parameters like probing depths greater than 6 mm, bleeding and/or suppuration on probing and radiographic evidence of bone loss can be utilized for diagnosing peri-implant diseases. Advanced peri-implantitis is classically associated with a trough of bone around the implant. The bone loss tends to increase with time and usually requires surgical treatment. Not surprisingly, patients who do not respond to periodontal maintenance therapy subsequently lose an increased number of implants (Fardal and Linden 2008).
1.9.1 Treatment of Peri-implant Disease: Nonsurgical Therapy
Many studies have found that the nonsurgical treatment of peri-implantitis does not produce predictable and sustained clinical improvement. In Fig. 1.3 and 1.4 continuous alveolar bone loss occurred despite regular implant surface debridement and oral hygiene instruction. The difficulty arises for the dentist in cleaning a moderately roughened implant surface using traditional hand instruments. Similar difficulties occur for the patient in cleaning sub-gingival restoration margins. Air-abrasive devices provide short-term (6 months) improvement in probing depth and clinical attachment level when treating moderate degrees of peri-implantitis (Sahm et al. 2011). Other studies have found no improvement in probing pocket depth and bone levels with sub-gingival debridement using ultrasonic devices and curettes (Karring et al. 2005). In a small, randomized controlled trial (Heitz-Mayfield et al. 2011), nonsurgical debridement had some effect in reducing bleeding on probing around implants (healing was successful in 38 % of treated implants at 3 months), but brushing with chlorhexidine gel had no additive effect. Another short-term, 3-month study found a similar conclusion: that mechanical debridement was effective in reducing mucosal inflammation when implant pocket depths were less than 5 mm, but that chlorhexidine gel provided no additional benefit (Porras et al. 2002). There is also a tendency of chlorhexidine to cause staining and an altered taste sensation, but chlorhexidine gel does not harm the titanium surface when used as disinfecting agents (Ungvari et al. 2010).
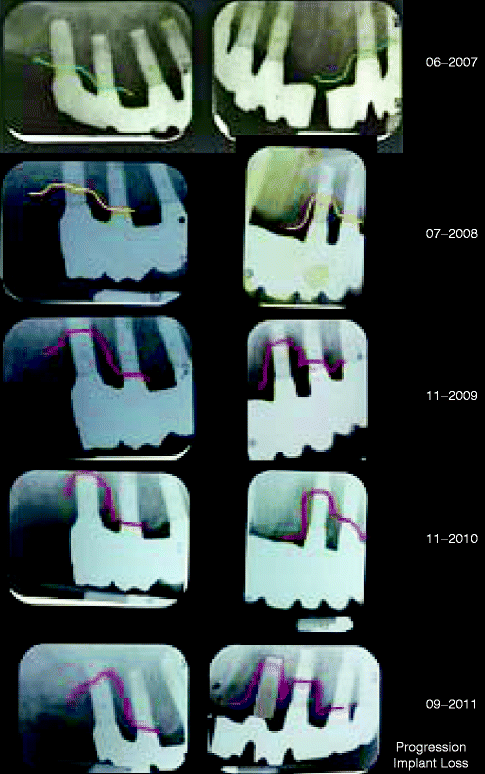
Fig. 1.3
Progression of implant bone loss over a 4-year period despite regular local debridement. The colored lines indicate the periodontal bone level
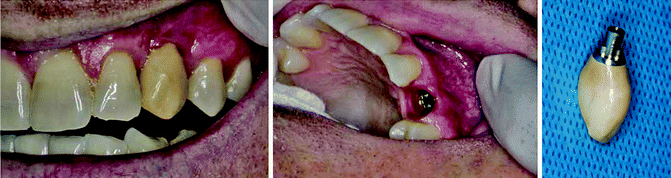
Fig. 1.4
Peri-implant gingival inflammation
In a 6-month oral hygiene programme, which recruited patients with mild peri-implantitis, an air-abrasive system was found to reduce the mean probing depth by about 0.5 mm (Sahm et al. 2011). These changes are not clinically significant and may result from the patient’s improved oral hygiene rather than the air-abrasive intervention. In peri-implantitis, one application of an air-abrasive device or an erbium-doped yttrium, aluminium, and garnet (Er:YAG) laser was ineffective at reducing bacterial counts after 6 months or in showing any significant improvement in clinical parameters (Persson et al. 2011).
Peri-mucositis can be prevented by twice-daily use of an antiseptic mouthwash (Listerine, Warner-Lambert Co., NJ) when used as an adjunct to toothbrushing. This was shown in a double-blind, randomized clinical study that compared the antiseptic with a similar-tasting placebo mouthwash (Ciancio et al. 1995). This measure is simple, effective and readily accepted by patients. Listerine, hydrogen peroxide and chlorhexidine all have an antibacterial effect on oral biofilm microorganisms adhering to a titanium surface (Gosau et al. 2010), but their value in treating established peri-implant disease is in doubt. A 3 % hydrogen peroxide applied for 5 min or chlorhexidine gel solution applied for 5 min does not harm the titanium surface (Ungvari et al. 2010), but high-fluoride, acidic gels do cause corrosion of titanium (Stajer et al. 2008). These antiseptic solutions are supplemental measures because plaque must be mechanically removed, as failure to do so will result in bacterial recolonization of the biofilm.
1.9.2 Surgical Treatment of Peri-implantitis
Nonsurgical therapy does not provide successful results in the treatment of severe peri-implantitis but does have a place in establishing optimal gingival tissue health prior to surgery. Residual pockets tend to remain and these usually require surgical elimination, but resective surgery can cause aesthetic problems in the anterior region of the mouth. A Cochrane review was unable to determine which technique was the most effective treatment for peri-implantitis (Esposito et al. 2006). This was because of problems in the reported clinical trials with small sample sizes, short follow-up periods, analysis based on implants and not patients and failures to report the initial severity of the peri-implantitis. In the absence of clear guidance from the available literature as to which treatment of peri-implantitis is superior, the clinician should use that intervention which has minimal side effects and cost.
In a study that undertook plaque control, pre-surgical antibiotics, surgical pocket elimination and bone recontouring, half of the patients (48 %) had no bleeding on probing after a short period of follow-up (up to 2 years) (Serino and Turri 2011). Treatment was more successful (74 %) in those implants with minor levels of initial bone loss compared with only 50 % for those implants with an initial bone loss of 5–6 mm. Treatment success is mainly dependent on the severity of bone loss around the implant. If bone loss around the implant is limited (at less than 2 mm), a nonsurgical treatment approach is recommended (Okayasu and Wang 2011). Peri-implant bone loss can be treated successfully with flap surgery, removal of granulation tissue and effective cleaning of the implant surface, resulting in successful repair in the majority of patients. One study reported a median defect depth reduction of 6.2 mm using this protocol (Behneke et al. 2000). However, re-osseointegration following surgery is unlikely. A histological study in dogs found that following guided tissue regeneration, “fine fibrillar material” formed adjacent to the implant surface (Schupbach et al. 1994). Treated implants become stabilized and are well adapted to new bone but are not osseointegrated. In a systematic review which included 17 articles, it was concluded that the most likely outcome from using bone grafting techniques and membranes is an incomplete filling of the bony defect (Sahrmann et al. 2011).
Preventing further peri-implant bone loss is challenging, especially when it is accompanied by pocket depths >5 mm. Reports have documented the successful use of antimicrobial therapies combined with growth factors and guided tissue-regenerative membrane therapies (Froum et al. 2012). Membranes are used in surgery to stabilize the bone graft around the peri-implant defect. However, incorporating a membrane inevitably increases the complication rate in regenerative surgery. One study reported a loss of the membrane in 5 cases out of 42 augmentations due to infection, with further complications from membrane dehiscence (Gaggl and Schultes 1999). Membrane instability, exposure of the membrane or a failure to adequately remove bacterial contamination from the implant surface at surgery may be responsible for augmentation failure.
Many studies have reported the use of an air-powder cleaning device or citric acid to clean the implant surface. Air-powder systems and resin curettes result in far less surface alteration than using steel curettes or ultrasonic devices designed for cleaning teeth (Meschenmoser et al. 1996; Brookshire et al. 1997). Short, 5-s exposures to the air-abrasive system did not cause harmful changes to the implant surface, but longer 15-s exposures did roughen the implant surfaces (Chairay et al. 1997). It is difficult to make general clinical recommendations as different studies used different operating parameters, e.g. the air pressure of the device and the nature and particle size of the powder.
The use of antibiotics as the sole treatment of peri-implantitis has little support in the literature. A variety of different antibiotic regimes have been recommended but with unpredictable outcomes. A systematic review found no evidence in favour of any particular antibiotic treatment protocol (Klinge et al. 2002). As with the treatment of chronic periodontitis, the resolution of peri-implantitis requires local debridement or curettage and regular plaque control, with antibiotics playing a less important role (Ericsson et al. 1996). Recolonization of the peri-implant pocket and further bone loss are inevitable without debridement.
It would be prudent for a clinician to start with the minimum treatment to debride the pocket and prevent further bone loss rather than with a more aggressive treatment that may be unpredictable.
1.10 Future Research Trends in Peri-implantitis
New clinical guidelines and antibacterial strategies need to be developed to manage peri-implantitis and its associated implant loss (Fig. 1.5). The biofilm that develops in peri-implantitis generally resembles that found in chronic periodontitis and mainly consists of gram-negative, anaerobic organisms. However, Staphylococci, E. coli, and Candida species are not normally associated with periodontitis but are found in a high proportion of peri-implantitis lesions (Leonhardt et al. 1999). Some of these organisms are resistant to antibiotics and antiseptics, and this may explain the inconsistent results that are found with various treatments of peri-implantitis. Paradoxically, while manufacturers have designed implant surfaces to be an attractive environment for connective tissue, epithelial and bone cells, these surfaces are also easily colonized by bacterial cells. The result is that once a pathogenic bacterial biofilm becomes established, it is difficult to eliminate and treatment can often be unpredictable and unsatisfactory.
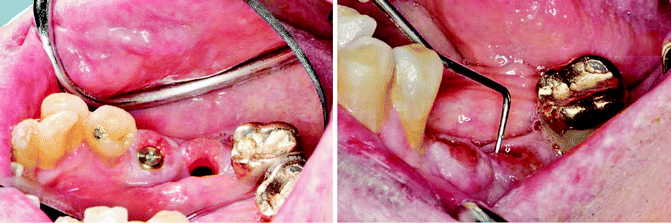
Fig. 1.5
Peri-implant inflammation and bone loss (10-mm probing depth) and eventual implant loss
If the titanium oxide surface of the implant is modified to contain crystalline anatase, the surface has a reduced amount of bacterial adhesion (Del et al. 2005). Similarly, titanium surfaces modified with fluorine ions had a decreased growth of P. gingivalis and A. actinomycetemcomitans compared with the unmodified titanium surface (Yoshinari et al. 2001). The fluorine-modified surfaces had no deleterious effect on the proliferation of fibroblast cells in vitro. Fluoride ions were not released to cause an inhibitory effect; rather it was the metal fluoride surface that caused the antibacterial activity. However, the effect of this coating on osseointegration is untested.
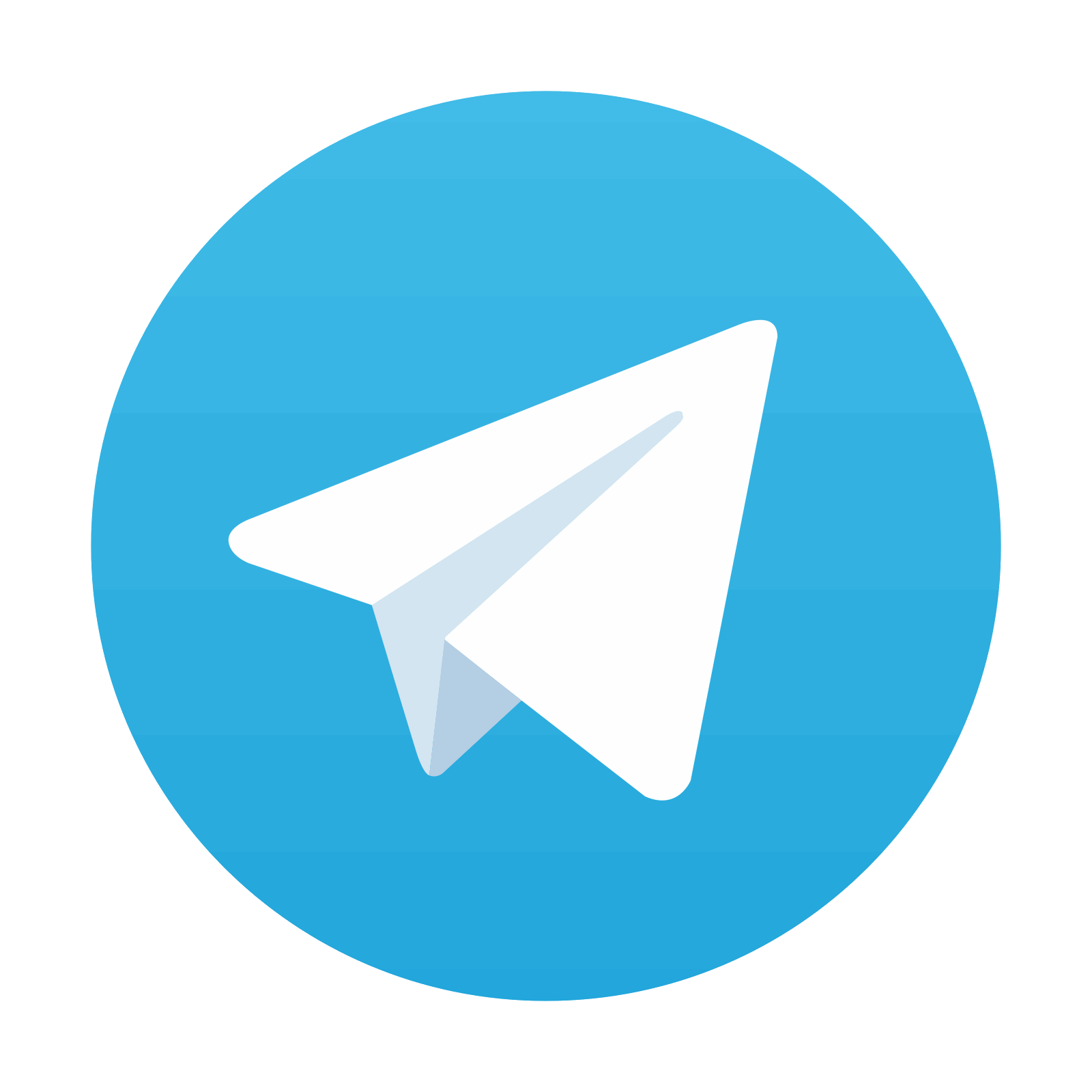
Stay updated, free dental videos. Join our Telegram channel

VIDEdental - Online dental courses
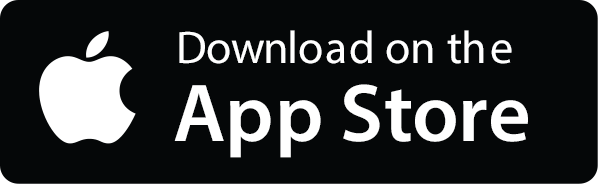
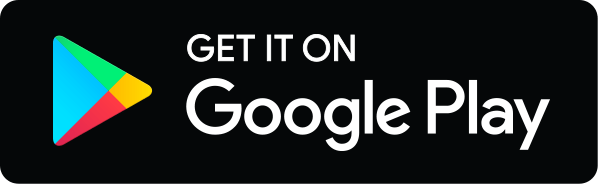