Abstract
Objective
Agglomeration is a common problem facing the preparation and application of nanomaterials, and whether nano-hydroxyapatite (nano HA) can modulate oral microecology left to be unclear. In this study, nano HA was disaggregated by sodium hexametaphosphate (SHMP) and ultrasonic cavitation to observe whether agglomeration would affect its effect on oral bacterial biofilm.
Methods
Dynamic light scattering (DLS) and scanning electronic microscope (SEM) were used to observe the treatment solutions. Single-species biofilms and multi-species biofilms were treated with 10% nano HA, 10% disaggregated nano HA, 10% micro hydroxyapatite (micro HA) and deionized water (DDW) for 30 min and analyzed via MTT assay, lactic acid measurement, SEM and confocal laser scanning microscope (CLSM). Real-time polymerase chain reaction was performed to analyze the biofilm composition.
Results
Ultrasonic cavitation combined with SHMP could significantly reduce the degree of agglomeration of nano HA. Disaggregated nano HA could inhibit bacterial growth and reduce the ability of bacterial biofilm to produce lactic acid and extracellular polysaccharides. There was no significant difference on composition of multi-species biofilms between nano HA and disaggregated nano HA.
Significance
The disaggregated nano-hydroxyapatite could inhibit the metabolism and acid production of oral bacterial biofilm, but did not significantly affect the composition of multi-species biofilms.
1
Introduction
Dental caries is the most common and widespread oral disease, which is a chronic progressive bacteria infectious disease on dental hard tissue . According to the global burden of disease data released by the Lancet in 2017, the prevalence of permanent teeth caries ranked No.1 among the 358 major diseases in the world . When oral microecology is disturbed, aciduric and acidogenesis bacteria such as Streptococcus mutans ( S. mutans ) predominate in the dental plaque . As acid being produced continually, the low-pH condition disrupts the homeostatic mineral balance toward demineralization, which then results in the development of dental decay . Hence there are two main strategies for the prevention of dental caries: Firstly, to modulate the enamel demineralization toward the salutary remineralization; Secondly, to inhibit bacterial growth and acid production . So, anti-caries agents with double benefits should be ideal materials to prevent dental caries.
Hydroxyapatite (HA) has attracted attention due to its broad range of applications in dentistry and medicine in recent years . The chemical formula and crystal structure of HA are quite similar to those of main inorganic constituent of human bones and teeth. It is one of few materials that can facilitate bone in-growth and osseo-integration, when applied in orthopedic and dental applications for repairing or substituting hard tissues . Nano-hydroxyapatite (nano HA) refers to the hydroxyapatite particles of which particle size ranges from 1 to 100 nm . This nano-material can be integrated into native enamel and infiltrate the collagen structure of demineralized dentin . Therefore, the remineralization of enamel and dentin by nano HA is widely used in previous studies . To date, many products containing nano HA have been developed such as toothpaste and sports drinks, which have a certain effect on inhibiting tooth demineralization . However, a few studies have been performed to investigate the antibacterial effect of nano HA. Zhang et al . immersed biofilm of S. mutans in nano HA suspension and found no significant difference in colony-forming units between the experimental group (nano HA) and the control group (water) . Another study found that nano HA could effectively adsorb bacteria and inhibit the production of acids by microorganisms . However, there is still a lack of unified conclusions and comprehensive reports on whether nano HA can modulate oral microecology.
Agglomeration is a common problem in the process of preparation and application of nanomaterials . Nanomaterials have small particle size and large surface energy, resulting in the materials in an energy unstable state . Hence they are inclined to attract each other and group into several hundred nanometer clusters. Interparticle aggregation may lead to the loss of nano HA’s unique properties which are ascribed to their nanometer level size. Lots of methods had been applied to disaggregate nanoparticles, such as washing by organic matter, surface modification, chemical dispersant, and ultrasonic cavitation . Previous studies have elucidated that enhancing the dispersion properties of nanomaterials ensures their transparency, low surface roughness and high interface area, which are critical for optical applications and composites synthesis . Nevertheless, to our knowledge, whether dispersion state affects the antibacterial effect of nano HA has not yet been reported. However, disaggregated nano HA is considered as a promising strategy to prevent dental caries. Besides, previous studies did not elucidate the agglomeration status of nano HA during the process of experiments, which may be the underlying reason for obtaining controversial experimental results . Therefore, ultrasonic wave was used in this study as a physical dispersion method. Considering that nanoparticles may re-agglomerate back into large sized clusters shortly after ultrasonic cavitation, sodium hexametaphosphate is also added to against reagglomeration . Sodium hexametaphosphate (SHMP) is a chemical dispersant that characterizes good biocompatibility without bactericidal effect, which has been proofed by previous study .
Therefore, the objectives of this study were to: (1) investigate the efficiency of ultrasonic cavitation combined with SHMP for deagglomerating; (2) investigate whether agglomeration of nano HA would affect its antibacterial properties; and (3) test the effects of hydroxyapatite of different particle sizes on dental plaque biofilms.
2
Materials and methods
2.1
Bacterial strains and growth conditions
The strains used in this study were Streptococcus mutans ATCC 700610 ( S. mutans UA159), Streptococcus sanguinis ATCC 10556 ( S. sanguinis ), Streptococcus gordonii ATCC10558 ( S. gordonii ), which were provided by the State Key Laboratory of Oral Diseases (Sichuan University, Chengdu, China). They were routinely grown overnight on brain heart infusion (BHI) fluid mediums (BD, America) in anaerobic incubator (90% N 2 , 5% CO 2 , 5% H 2 ) at 37 °C.
2.2
Biofilm model
Biofilms were formed on the glass slides on the bottom of 24-well plates. Briefly, sterile glass slides were put into a 24-well plate. For development of the single-species biofilms, each slide was inoculated with approximately 10 6 CFU/mL of S. mutans UA159 in 2 mL BHI solution containing 1% sucrose. The bacterial suspensions cultured overnight were microscopically examined. After the morphology of the strains were determined normal, the concentration of the bacterial suspensions were adjusted to 0.33 McFarland with a turbidimeter (Biored, America). Then the bacterial suspensions were diluted 10 5 times, and 100 μL of the solutions were pipetted and inoculated on BHI agar plate (BD, America). The number of colonies was counted after cultured in anaerobic incubator (90% N 2 , 5% CO 2 , 5% H 2 ) for 48 h at 37 °C. When the concentration of the bacterial solution is 0.33 McFarland, the CFU of each species is: S. mutans was 3.71 × 10 8 CFU/mL, S. sanguinis was 1.39 × 10 8 CFU/mL, and S. gordonii was 3.04 × 10 8 CFU/mL. For multiple-species biofilms of S. mutans UA159, S. sanguinis and S. gordonii , the proportion of each bacteria was adjusted to 1:1:1 (when the concentrations were adjusted to 0.33 McFarland, took 1 mL of S. mutans solution, 2.67 mL of S. sanguinis solution, 1.22 mL of S. gordonii solution. The concentration of each bacteria was approximately 10 6 CFU/mL) and the mixed bacterial liquid was inoculated to 24-well plate. The plates were cultured in the anaerobic chamber at 37 °C for 24 h so that biofilms were formed on the glass slides.
2.3
Samples preparation
The treatments of biofilms included sterile deionized water (DDW), 10% (w/v) micron hydroxyapatite (micro HA; Sigma–Aldrich, America) suspension and 10% (w/v) nano HA (Sigma–Aldrich, America) suspension were prepared in sterile deionized water. Then 10% (w/v) nano HA was disaggregated by ultrasonic cavitation combined with SHMP together (nano HA 1). Untreated nano HA is labeled nano HA 2. Nano hydroxyapatite suspensions were subjected to dynamic light scattering (DLS) test at 25 °C using a Zetasizer DLS analyzer (Malvern, UK) to determine the particle size distribution. For scanning electronic microscope analysis, 10 μL of the treatment solutions were added to the 24-well plate, dried in a cool place, and observed under a scanning electronic microscope (SEM; Olympus, Japan).
Four groups of treatment solution (nano HA 1, nano HA 2, micro HA and DDW) were added into the new 24-well plate. Each group had 6 wells and each well had 1 mL solution. The samples with biofilms were taken out from the old 24-well plate, and gently rinsed with phosphate buffered saline (PBS) solution in order to remove unattached bacteria. And then they were put into 24-well plate with treatment solution. After a 30-min immersion, the samples were removed from treatment solutions and rinsed with PBS to remove remaining treatment material. All samples were freshly made for subsequent test and analysis.
2.4
Biofilm metabolic activity analysis
Biofilm samples immersed in 1 mL of 3-(4,5-Dimethylthiazol-2-yl)-2,5-diphenyltetrazolium bromide (MTT, Sigma-Aldrich, America) solution (MTT concentration: 5 mg/mL) was added into a new 24-well plate. The plate was incubated in the anaerobic chamber for 1 hour at 37 °C. Then 1 mL of Dimethyl sulfoxide (DMSO; Sigma–Aldrich, America) was added to each well in a new 24-well plate, and the samples were transferred to the new plate. The new plate was shaken slowly at room temperature for 20 min in aerobic conditions. Finally, the content of each well was transferred to a 96-well plate and its absorbance was measured at 540 nm using a microplate reader (ELIASA E-1309, Beckman, America).
2.5
Lactic acid production assay
Biofilms were treated as described above and transferred to a new 24-well plate containing 1.5 mL Buffered peptone water (BPW; Sigma-Aldrich, America) solution plus 0.2% sucrose (w/v). The plate was incubated anaerobically for 3 h at 37 °C to allow the biofilms to produce lactic acid . After that, BPW solutions were collected to analyze the lactate content using an enzymatic method . A microplate reader (ELIASA E-1309, Beckman, America) was used to measure the absorbance at 340 nm. Standard curves were prepared using a lactic acid standard (Supelco Analytical, Bellefonte, PA, USA).
2.6
Biofilm structure detected by scanning electronic microscope
The architecture of multi-species biofilms after treatment was examined by SEM. Briefly, biofilm was washed with PBS, and then immersed in 2.5% glutaraldehyde (v/v) under 4 °C overnight. Then washed twice with PBS and dehydrated for 10 min using a graded ethanol solutions series; 30%, 50%, 70%, 80%, 85%, 90%, 95%, 100% (v/v). Finally, biofilm was rinsed for 2 times with hexamethyl-disilazane and observed after gold spraying under SEM (Quanta 200, FEI, Hillsboro, OR, USA) to examine the structure of biofilm.
2.7
Extracellular polymeric substance (EPS) of biofilm observed by confocal laser scanning microscope (CLSM)
For extracellular polymeric substance (EPS) staining, dye Alexa Fluor 647 (Molecular Probes, Invitrogen, America) was added at the beginning of biofilm construction at proportion of 1:1000 and then cultured in the dark. The biofilm harvested after 24 h was immersed in the treatment solution for 30 min. Then the biofilm was washed with PBS to remove residual treatment materials and dried. Fifty μL of SYTO 9 nucleic acid dye (Molecular Probes, Invitrogen, America) was added to each sample, which was placed in dark for 15 min. After that, deionized water was used to remove the excess dye, and the samples were dried and observed under a confocal laser scanning microscope (CLSM, BX61, Olympus, Tokyo, Japan) with 60 × oil immersion objective lens. The live bacterial cells were labeled by SYTO 9 to produce green fluorescence (514/488 nm). EPS was stained with Alexa Fluor 647 to produce a red fluorescence (570/543 nm). The three-dimensional reconstruction of biofilms was performed with IMARIS 7.0 (Bitplane, Zürich, Switzerland).
2.8
DNA extraction and real-time polymerase chain reaction
For DNA extraction, the biofilm was washed in PBS solution gently and scraped off with a sterile blade. DNA in the biofilm was extracted and purified using the bacterial genomic DNA kit (TIANamp Bacteria DNA Kit, Tiangen Biotech, China) according to the instructions. The concentration and purity of the extracted DNA were analyzed using NanoDrop 2000 spectrophotometer (Thermo Fisher Scientific, America). The sequences of primers and probes were designed ( Table 1 ). The reaction proceeded in CFX96 Touch™ fluorescence quantitative PCR detection system (Bio-Rad, Hercules, America). The data were analyzed by CFX -Manager software (Bio-Rad, Hercules, America).
Primer/Probe | Sequence (5’-3’) |
---|---|
Primer | |
S. mutans -f | 5’-GCCTACAGCTCAGAGATGCTATTCT-3’ |
S. mutans -r | 5’-GCCATACACCACTCATGAATTGA-3’ |
S. gordonii -f | 5’-GGTGTTGTTTGACCCGTTCAG-3’ |
S. gordonii -r | 5’-AGTCCATCCCACGAGCACAG-3’ |
S. sanguinis -f | 5’-GAGCGGATGGCCAATTATATCT-3’ |
S. sanguinis -r | 5’-CCGGATGATGTCGGCAATA-3’ |
Probe | |
S. mutans | 5’-FAM-TGGAAATGACGGTCGCCGTTATGAA-TAMRA-3’ |
S. gordonii | 5’-FAM-AACCTTGACCCGCTCATTACCAGCTAGTATG-TAMRA-3’ |
S. sanguinis | 5’-FAM-TGTTCGGGCTCATGATA-Eclipse-3’ |
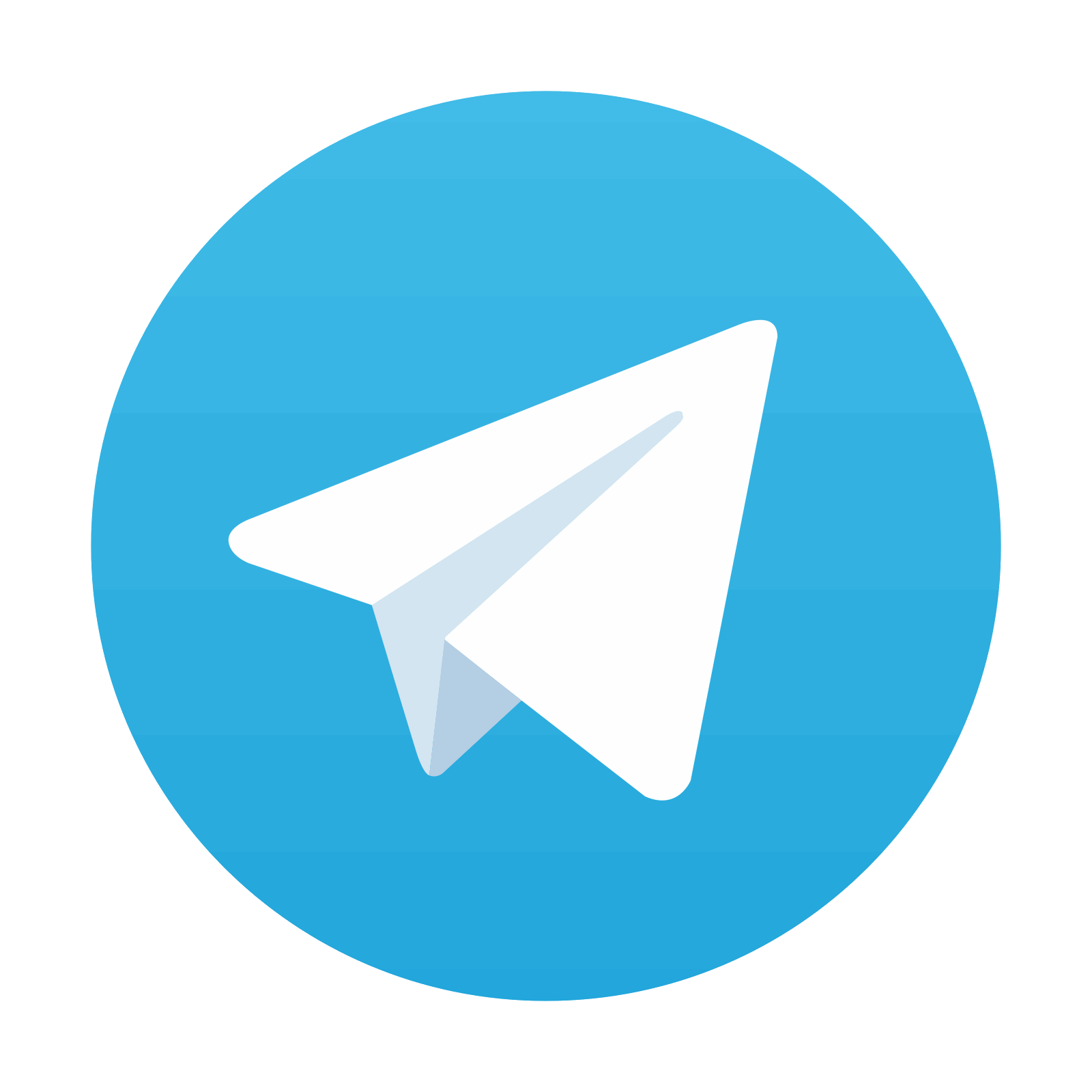
Stay updated, free dental videos. Join our Telegram channel

VIDEdental - Online dental courses
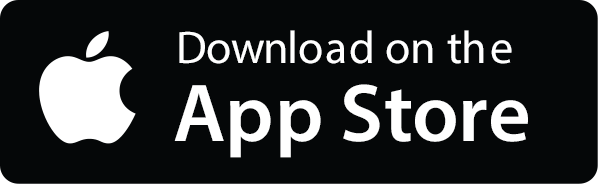
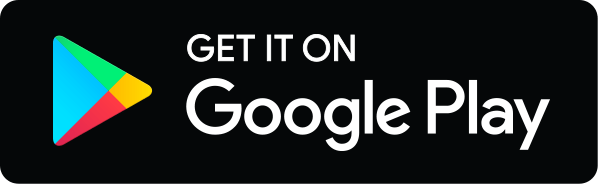
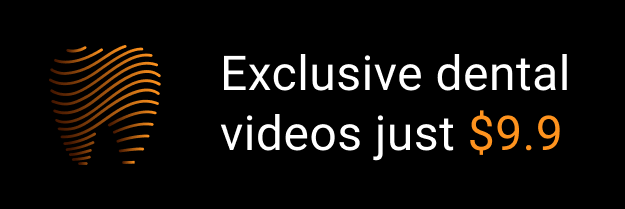