Abstract
Narrow-diameter implants (NDIs) traditionally have been associated to higher rates of failure in comparison with regular-diameter implants (RDIs) and wide-diameter implants (WDIs), since they generate a more unfavorable stress distribution in peri-implant bone. However, it is well known that the load sharing effect associated with prostheses supported by multiple implants (also called splinted prostheses) affords mechanical benefits. The present study involves finite element analysis (FEA) to determine whether the risks linked to NDIs could be mitigated by the mechanical advantages afforded by the splinting concept. For this purpose, a three-dimensional (3D) model of a real maxilla was reconstructed from computed tomography (CT) images, and different implants (NDIs, RDIs and WDIs) and prostheses were created using computer-aided design (CAD) tools. Biting forces were simulated on the prostheses corresponding to three different rehabilitation solutions: single-implant restoration, three-unit bridge and all-on-four treatment. Stress distribution around the implants was calculated, and overloading in bone was quantified within peri-implant volumes enclosed by cylinders with a diameter 0.1 mm greater than that of each implant. The mechanical benefits of the splinting concept were confirmed: the peri-implant overloaded volume around NDIs splinted by means of the three-unit bridge was significantly reduced in comparison with the nonsplinted condition and, most importantly, proved even smaller than that around nonsplinted implants with a larger diameter (RDIs). However, splinted NDIs supporting the all-on-four prosthesis led to the highest risk of overloading found in the study, due to the increase in compressive stress generated around the tilted implant when loading the cantilevered molar.
1
Introduction
In recent years, dental implants have been used as surgical components in a wide variety of oral restoration scenarios, including single-tooth replacements or multiple implant-supported bridgeworks replacing various missing teeth. The success of the treatment depends on a number of biological and mechanical factors. Even when implants are fully osseointegrated, there is a risk of bone resorption in the peri-implant regions, which can be activated by bacterial infection or overloading due to masticatory forces . Overloading is often the result of inefficient stress distribution generated by inappropriate occlusion, implant design or positioning. In this context, an increased area of bone-to-implant contact (BIC) may reduce the risk of overloading in peri-implant bone by improving osseointegration and achieving a more balanced stress distribution. Among the options in pursuit of this objective, increasing the implant diameter has proven to be a successful strategy .
The term narrow-diameter implants (NDIs) usually refers to implants with a diameter <3.4 mm . Such diameters are reportedly large enough to provide satisfactory long-term outcomes. In some clinical situations, NDIs represent an option for restoring narrow alveolar ridges and sites with limited space for the insertion of standard diameter implants (SDIs), reducing the need for bone augmentation procedures . Although some follow-up studies have reported similar survival rates for NDIs and SDIs , it has been stated that the resistance of NDIs is 25% less than that of SDIs , and that the risk of fatigue fracture increases when using small diameters . Furthermore, some studies based on finite element analysis (FEA) have concluded that narrow diameters involve higher stress and strain levels at peri-implant bone level when compared with conventional diameters, anticipating a higher rate of bone loss . These concerns explain the fact that NDIs traditionally have been restricted to concrete situations such as the rehabilitation of incisors but are not commonly used in areas exposed to high masticatory forces such as the molar regions .
On the other hand, the term splinting (or stabilization) refers to binding teeth or implants together to increase the stability of the structure as a whole. The rationale behind splinted implant prostheses is to achieve favorable distribution of non-axial forces and increase the total area receiving the load. Some authors have reported that splinting implies biomechanical advantages and leads to load sharing . However, research on the clinical use of splinted NDIs is still limited . Similarly, FEA studies are generally focused on single-unit restorations or fixed prostheses supported by multiple SDIs, but FEA-based studies evaluating multiple NDI-supported prostheses are still scarce. Although splinted mini-implants (1.8 mm in diameter) compared with single mini-implants clinically show a similar bone loss , splinting of implants reduces the tensile stress in the posterior area of short fixed bridges . Accordingly, the present study used FEA to determine whether the risks associated with NDIs could be decreased to a significant degree by the load-sharing effect resulting from the splinting concept.
2
Materials and methods
In this study, the biomechanical benefits associated with splinted NDIs were investigated by FEA. In order to allow quantitative comparisons between different implant diameters, the study was extended to regular-diameter implants (RDIs) and wide-diameter implants (WDIs). Three prosthetic solutions including nonsplinted and splinted implants were three-dimensionally (3D) modeled as shown in Fig. 1 . Firstly, the InVesalius 3.0 software ( http://www.cti.gov.br/invesalius/ ) was used to reconstruct a maxilla from DICOM images of an upper jaw belonging to a 72-year-old edentulous male. The reconstructed maxilla was imported to Solidworks© (Dassault Systèmes Solid Works© Corp., Concord, USA), and two different regions were generated: an inner volume simulating cancellous bone and an external layer representing cortical bone. The thickness of the cortical layer in the different regions of the maxilla was selected in accordance with other authors . Two different maxillary bone segments were generated: one half of the maxilla without the sinuses (for single-implant restorations and three-unit bridges) and a “full” maxilla conserving the maxillary sinuses (for all-on-four treatments).

Three-dimensional implants and superstructures were designed via CAD with Solidworks© and 3D modeling software. The implants were based on commercially available components (ADIN© Dental Implant Systems, Ltd., Afula, IL, USA). A total of 6 implants were created: implants with three different diameters (NDI – 3.0 mm; RDI – 3.5 mm; and WDI – 4.3 mm) of two different lengths (10 mm and 13 mm). Straight and 30°-angled abutments based on the same brand were also generated. On the other hand, three single dental structures (supported by individual implants) and a splinted three-unit bridge (supported by two implants) replacing the first premolar, second premolar and first molar were modeled. A splinted full arc bridge, characteristic of the all-on-four concept , was also designed. In the case of the single-implant rehabilitation and the three-unit bridge, 10 mm long implants and straight abutments with a transmucosal height of 3 mm were used. For the all-on-four concept, posterior 13 mm long implants were attached to angled abutments with a transmucosal height of 3 mm, and anterior 10 mm long implants were connected to straight abutments with a transmucosal height of 2 mm. On the basis of other studies , cratering effects were modeled around the abutment to simulate the well-known bone resorption that occurs in the areas close to the implant neck.
The models were transferred from Solidworks® to COMSOL Multiphysics® (COMSOL, Inc., Burlington, USA) to carry out static linear elastic simulations. The material properties assigned to each domain are given in Table 1 . A zero-displacement boundary condition was defined for the flat faces of the maxillary segments. Three different loading scenarios were considered for each of the three rehabilitation solutions: first premolar loaded (250 N), second premolar loaded (300 N) and first molar loaded (300 N). The loads were uniformly distributed in the occlusal regions, and their magnitudes were similar to experimental single tooth bite forces in healthy young male adults . The angle between the implants axes and the forces was 15° . The direction of the force vector was based on the maximum forces analyzed in previous studies . Total osseointegration of the implants was considered, since node-to-node correspondences at the interface between the implants and bone were assumed. After a convergence test, the models representing the single-implant restoration, the three-unit bridge and the all-on-four rehabilitation were meshed with approximately 0.7, 1.2 and 3 million elements, respectively. Finally, the solutions were computed and the data of specific volumes of interest (VOIs) were extracted for quantitative evaluation of the results. Such volumes (shown in Fig. 1 ) included the fraction of peri-implant cancellous and cortical bone enclosed by cylinders of different diameters (3.1, 3.6 and 4.4), depending on the implant diameter (3.0, 3.5 and 4.3, respectively).
Component and material | Elastic modulus (GPa) | Poisson ratio |
---|---|---|
Cortical bone | 13.7 | 0.3 |
Cancellous bone | 0.5 | 0.3 |
Implants and abutments: Ti-6Al-4V | 110 | 0.35 |
Superstructures: Cr-Co alloy | 218 | 0.33 |
In accordance with similar studies , overloading of cancellous and cortical bone was considered to occur when the maximum or minimum principal stresses exceeded their uniaxial tensile or compressive strength, respectively. This consideration was made on the basis of the maximum normal stress criterion, usually linked to brittle materials. The strength of cortical bone was assumed to be 100 MPa under tension and 170 MPa under compression. In cancellous bone, a strength of 5 MPa was adopted for both tension and compression . Therefore, in the present paper the overloading risk in bone for the different prosthetic solutions was measured through the quantification of the partial volume overloaded under tension (OV T ), where the maximum principal stress exceeds the tensile strength of bone, and the partial volume overloaded under compression (OV C ), where the minimum principal stress exceeds the compressive strength of bone, with the total overloaded volume (OV) being the sum of both contributions: OV = OV T + OV C .
3
Results
Fig. 2 shows, as a comparative example of the stress field around splinted and nonsplinted implants, the distribution of maximum and minimum principal stress in the models corresponding to the single-implant rehabilitation and the three-unit bridge, specifically when loading the first premolar. The reason for distinguishing between minimum and maximum principal stress is to identify the areas where overloading occurs due to either compressive or tensile stress, respectively, according to the maximum normal stress criterion defined above. The cross sections in this figure were obtained by selecting planes containing the direction of the force applied.

Fig. 2 offers a comprehensive view of the effect of the implant diameter on the stress distribution in bone. A decrease in size of the areas affected by stress levels above or close to the physiological limits occurs when the implant diameter is increased. Furthermore, on comparing the single-implant rehabilitation with the two-implant-supported bridge, a reduction in stress levels around the first premolar (P1; where the load is applied) is noticed. This is obviously a result of the splinting concept and its subsequent load-sharing effect. Such observations can be verified in quantitative terms in Fig. 3 , which depicts the mean principal maximum and minimum stress in the VOIs around the implants supporting the single restoration and the bridge, together with the magnitude of the total overloaded volume within these VOIs (i.e. OV). It should be remembered that OV encompasses both the partial overloaded volume under tension (OV T ; i.e. those regions in which the maximum principal stress exceeds the uniaxial tensile strength of bone) and the partial overloaded volume under compression (OV C ; i.e. those regions where the minimum principal stress exceeds the uniaxial compressive strength of bone).
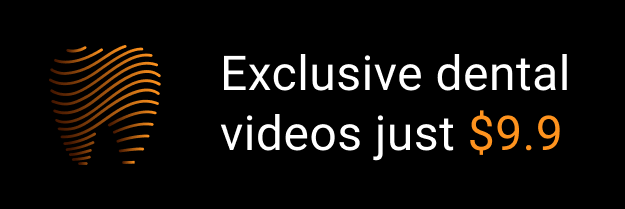