© Springer International Publishing Switzerland 2016
Barbara Zavan and Eriberto Bressan (eds.)Dental Stem Cells: Regenerative PotentialStem Cell Biology and Regenerative Medicine10.1007/978-3-319-33299-4_5
Dental Stem Cells and Growth Factors
(1)
Centre for Integrative Biology (CIBIO), University of Trento, Trento, Italy
(2)
Department of Neurosciences, University of Padova, Padova, Italy
Keywords
MSCDPScBone regenerationBMPGrowth factors
Stem Cells, Scaffolds and Growth Factors
The aim of the regenerative medicine and tissue engineering is to regenerate and repair the damaged cells and tissues in order to establish the normal functions [1]. The regenerative medicine involves the use of scaffolds, growth factors and stem cells [2]. Regeneration of the tissues exists naturally due to the presence of stem cells with the potential to self-regenerate and differentiate into one of more specialized cell types [3].
Stem cells are immature and unspecialized cells with the ability to renew and divide themselves indefinitely through “self-renewal” and able to differentiate into multiple cell lineages [4]. In the last decade, several improvements have been produced in the comprehension of stem cells properties in view of the fact that these cells have an important role in the repair of every organ and tissue [3].
In general, the stem cells are divided into three main types that can be utilized for tissue repair and regeneration: (1) the embryonic stem cells derived from embryos (ES) [5, 6]; (2) the adult stem cells that are derived from adult tissue [7]; and (3) the induced pluripotent stem (iPS) cells that have been produced artificially via genetic manipulation of the somatic cells [8]. ES and iPS cells are considered pluripotent stem cells because they can develop into all types of cells from all three germinal layers. On the contrary, adult stem cells are multipotent because they can only differentiate into a restricted number of cell types. Adult stem cells, also termed postnatal stem cells or somatic stem cells, are discovered in a particular area of each tissue named “stem cell niche” [3].
Different type of postnatal stem cells resides in numerous mesenchymal tissues and these cells are at the same time referred to as mesenchymal stem cells (MSCs) [7, 9]. Different studies have showed that MSCs can be isolated from different tissues, such as peripheral blood, umbilical cord blood, amniotic membrane, adult connective, adipose and dental tissues [10–14].
Today, every cell population which has the following characteristics independently of its tissue source, is usually referred as MSCs: (1) they adhere to plastic and have a fibroblast-like morphology; (2) they have the capacity of self-renewal and could differentiate into cells of the mesenchymal lineage such as osteocytes, chondrocytes and adipocytes. In addition, MSCs also can also differentiate, under appropriate conditions, into cells of the endoderm and ectoderm lineages such as hepatocytes and neurons, respectively [15, 16].
Research related to MSC from oral origin began in 2000 [17] and every year numerous investigations have demonstrated that oral tissues, which are simply available for dentists, are a rich source for mesenchymal stem cells [18, 19].
Today numerous types of MSCs have been isolated from teeth: in 2000 MSCs were first isolated by Gronthos et al. from dental pulp (DPSCs) [17, 19]. Subsequently, MSCs have been also isolated from dental pulp of human exfoliated deciduous teeth (SHEDs) [20]. The periodontal ligament is another adult MSCs source in dental tissue, and periodontal ligament stem cells (PDLSCs) were isolated from extracted teeth [21]. Moreover, MSCs have been also isolated from developing dental tissues such as the dental follicle (DFPCs) [22] and apical papilla (SCAPs) [23].
These cells all share a number of features with the well-studied bone marrow-derived mesenchymal stem cells, including their ability to differentiate, albeit with varying efficacy, into cells of the major mesenchymal lineages: osteoblasts, chondrocytes, and adipocytes. However, these cells seem to differ in their propensity to differentiate into functional odontoblasts [24]. Further, some of these cells, such as the SCAPs, are isolated from specific developmental stages (in this case the apex of a developing tooth), and thus, do not serve as practical sources of cells for autologous cell-based transplantation [25].
Dental pulp stem cells (DPSCs) , stem cells from human exfoliated deciduous teeth (SHEDs) , SCAPs, and PDLSCs have all been successfully isolated and characterized both in vitro and in vivo. Because of the nature of the deciduous teeth, guiding the development of permanent teeth and eventual replacement by the permanent teeth, deciduous teeth may act as a very practical and easily accessibly reservoir for autologous stem cells and hold the most value in stem cell therapy [20].
Much excitement has recently surrounded the identification and application of these stem cells with therapeutic value, but equally important is the development of delivery methods for transplantation of these cells and the promotion of their efficient engraftment. Traditionally, stem cells are introduced into the body via injection into the site of interest or into the circulation. However, the efficacy of this methodology is questionable, since studies have shown poor cell survival, engraftment, and unpredictable differentiation in vivo [25].
The potential efficacy of stem cell delivery and differentiation may be improved with the adoption of tissue-engineered scaffolds for cell delivery and structural support. Delivery of dental stem cells can potentially be supported by scaffolds that provide both mechanical and molecular (growth Factors, integrin receptor engagement) cues for differentiation [26].
Properties of scaffolds include providing cell adhesion, enabling cell proliferation and differentiation, and mimicking microenvironment observed in natural tissues and organs. Unlike injection-based delivery, scaffolds allow for superior control for stem cell delivery and allow for impregnation with time-release growth factors, modulation of stiffness, pore size, and cell–substrate interaction. Some evidence suggests that in addition to directly participating in tissue regeneration, transplanted stem cells can also regulate tissue regeneration by secreting trophic factors [27]. These two properties of stem cells suggest that the proper local distribution of stem cells is critical, making bioscaffold-based delivery one of the most promising strategies in cell-based regenerative dentistry [25].
Apart from stem cells and an appropriate scaffold, regenerative medicine and tissue engineering also require the use of growth factors and ECM molecules that induce specific differentiation pathways and maintain the cellular phenotype.
Incorporation of growth factors and/or cytokines has been shown to increase the efficacy of cell-based regeneration, both in the context of exogenous cell delivery, and recruitment of patients’ endogenous stem cells from the local environment in vivo [25]. Growth factors enhance dental stem cell activities by promoting the migration of endogenous cells and the subsequent proliferation, differentiation, angiogenesis and neuronal growth. Growth factors and cytokines are either autocrine or paracrine in nature and modulate cellular behavior by mediating intracellular communication [25]. Kim et al. recently discussed the effects of some growth factors in pulp-dentin regeneration [28]. These growth factors include platelet-derived growth factors (PDGF), transforming growth factor β (TGFb) family, vascular endothelial growth factor (VEGF), bone morphogenic proteins (BMPs), fibroblast growth factor (FGF) and etc. When delivered alone or with stem cells to sites of interest, they share common properties of promoting dentinogenesis, cellular proliferation and odontoblastic differentiation. PDGF, for example, normally released by platelets, induces chemotaxis and proliferation of stem cells by promoting angiogenesis and cell proliferation [29]. Rizk and Rabie demonstrated chondrogenesis of DPSCs transplanted into nude mice on poly-l-lactic acid/polyethylene glycol electrospun fiber scaffolds. These DPSC transplants were more efficient at chondrogenesis when transduced with TGFb3 virus, further supporting the notion that codelivery of growth factors can stimulate basal levels of stem cell differentiation upon transplantation in vivo [30].
Definition and Mechanism of Action of Growth Factors (GFs)
The term growth factors denominates a group of polypeptides of approx. 6–45 kD which have a role in controlling biological processes, such as cell growth, proliferation, differentiation and morphogenesis of tissues and organs during embryogenesis, postnatal growth and adulthood. GFs cannot pass through a cell’s membrane; they must bind to high-affinity cell receptors in order to take effect. Many GFs stimulate several cell populations, while others are less versatile and specific to a particular cell line [31].
Growth factors can act as mitogenes in that they enhance proliferation of certain cell types. Some growth factors are also morphogenic in that they change the phenotype of their target cells. Growth factors act in an autocrine manner by directing the secreting cell itself and in a paracrine fashion by affecting neighbouring cells. For some growth factors, an endocrine effect is assumed or even established because of elevated serum levels. Many growth factors are deposited in the extracellular matrix where they are released during matrix degradation and act as part of a complex network of signals with mutual effects during tissue remodelling and regeneration. The effect of growth factors is mediated through surface receptors on the target cells by activating intracellular phosphorylating enzymes, which in turn induce an intracellular signalling pathway by aggregation of co-factors and other proteins, which migrate to the nucleus. Together with other transcription factors they activate a set of genes, which then exert the specific changes in cellular activity or phenotype. In vivo, the effect of growth factors is regulated through a complex system of feed back loops, which involve other growth factors, enzymes and binding proteins [31].
Postnatal therapeutic application of growth factors for repair of damaged tissue or an organ is used to accomplish regeneration or generation of tissue by reinducing the developmental process that had created this organ or body part during foetal or postnatal growth [31]. Several authors have described the importance of growth factors in tissue repair processes, in fact, they are important elements for new tissue production, moreover, they perform feedback controls on inflammatory processes within the tissue graft, in cases of regenerative surgery [32–35].
As growth and development are subject to a complex regulation that is also essential for the suppression of malignant growth, there are concerns that unreflected enhancement of isolated signals in this complex system might initiate a process of uncontrolled growth. This is particularly true for factors that are also associated with the development of malignant tumours. Consideration of the use of growth factors, therefore, also has to include aspects of growth control and interactions with suppressing and activating co-factors [31].
Dental Stem Cells and Growth Factors
Human Recombinant and Autogenous Growth Factors
The identification of the genetic code of growth factors has enabled the production of human recombinant proteins more than 20 years ago. This has repeatedly raised hopes that effective clinical application is just around the corner. Unfortunately, despite extensive research and successful preclinical testing, the clinical use of human recombinant growth factors has not yet reached a level of application that would reflect the numerous successful experimental reports that have been published for more than 15 years [36]. Costs and regulatory issues as well as the complexity of mimicking physiological dosage levels at the site of delivery have rendered the introduction of human recombinant growth factors into clinical use more difficult than it has been anticipated [36, 37].
An alternative strategy that has been applied more frequently than the application of recombinant proteins is the use of patients’ own growth factors. Autogenous growth factors are present in rather large quantities in platelets and are easily available from platelet enriched centrifugation products of whole blood. Different concepts and preparation methods have been developed, that vary in the content of platelets, leukocytes and fibrin and hence the condition of application (liquid vs. gel) [38].
Platelet concentrates (PCs) contain a combination of growth factors. The concentration may vary between individual patients and according to the method of production [39–41]. The growth factors are contained in the alpha granules of the platelets. They can be released by addition of calcium chloride, allogeneic thrombin or by autologous fibrin depending on the preparation technique [38, 39, 42–45]. Other than individual recombinant growth factors, PCs convey a number of signals that may vary in their relative strength according to the dose levels present in the individual preparations. According to the nature of the growth factors contained in PCs, they are supposed to enhance mesenchymal and epithelial proliferation as well as angiogenesis.
Whitman [46] and Marx [47] published the first studies on the use of growth factors contained in platelet gel, called Platelet-Rich Plasma (PRP) . Thanks to Marx’s studies, it was possible to verify that the platelet concentrate is a very effective tool for the modulation of wound healing and tissue regeneration [3].
However, the PRP showed a number of disadvantages, such as the need of having to run a complex and expensive protocol for its production. To overcome some of these problems, the PRGF (Plasma Rich in Growth Factors) was introduced in the list of platelet concentrates. The PRGF is considered an evolution of the PRP and it allows a higher concentration of growth factors in platelet preparation [41, 48].
Among the advantages of the PRGF, we can cite the lesser amount of blood taken for the preparation and a procedure relatively faster, while, among the disadvantages we can mention the rapid clot formation, which require speed in its surgical use [3].
In 2001, Choukroun et coll. have instead proposed an alternative technique: the PRF (Platelet Rich Fibrin). PRF is derived from a simple preparation protocol that does not require alteration of the blood; it is a platelet concentrate rich in GFs that contains a three-dimensional matrix of autologous, elastic and flexible fibrin [36].
Dohan et al. have shown that platelet cytokines (PDGF, TGFbeta1 and IGF-1) are present in three-dimensional fibrin matrix derived from these platelet concentrates; moreover, PRF matrix traps glycosaminoglycans such as heparin and hyaluronic acid, which have considerable affinity with some peptides present in the bloodstream and therefore show strong ability of chemotaxis and diapedesis, useful for the healing of tissue damaged, for example, by trauma [3, 49].
Effect of Growth Factors on Dental Stem Cells
Fundamental to our understanding of regenerative medicine is the knowledge of growth factors that effect on a broad range of cellular activities including migration, proliferation, differentiation and apoptosis of cells, including stem/progenitor cells. Growth factors and cytokines may act as signaling molecules that modulate cell behavior by mediating intracellular communication. Growth factors are polypeptides or proteins that bind to specific receptors on the surface of target cells [51, 52]. They can initiate a cascade of intracellular signaling and act in either an autocrine or paracrine manner [53]. Cytokines are typically referred to as immunomodulatory proteins or polypeptides [54]. Cytokines are often used interchangeably with growth factors because many cytokines share similar actions as growth factors. As opposed to systemic effects by hormones on target cells, growth factors or cytokines typically act locally on target cells [28]. The effects of various growth factors on dental stem cells and how they may participate in dental pulp-dentin regeneration will now follow.
Platelet-Derived Growth Factor (PDGF)
Platelet derived growth factor (PDGF) is released by platelets, and has potency in promoting angiogenesis and cell proliferation [28, 55–58]. PDGF is a highly basic dimeric glycoprotein of 30 kD consisting of two disulphide bonded polypeptides encoded by different genes [30]. There are three isoforms characterized by the combination of A- and B-chains featuring two homodimeric (PDGF-AA and PDGF-BB) and one heterodimeric isoform (PDGF-AB) [59–61]. PDGF-BB and PDGF-AB are systemically circulating isoforms contained in alpha granules of platelets from where they are released after adhesion of platelets to injured sites of vessel walls, whereas PDGF-AA is secreted by unstimulated cells of the osteoblastic lineage [60–62]. PDGF stimulates specific target cells by binding to cell surface receptors with tyrosine kinase activity. PDGF plays an important role in the development of many tissues and organs in the growing embryo. It is essential not only for mesoderm induction and mesenchymal-epithelial interactions during organ development but also for development of the neural crest and patterning of limbs and myotomes in early embryogenesis [30, 63, 64].
The chemotaxis and proliferation of mesenchymal stem cells can be induced by PDGF in the injury site. In trauma, hemorrhage is followed by blood clot formation in dental pulp. Platelets in the blood clot release α-granules containing PDGFs and attract neutrophils and macrophages [56]. These cells play key roles in early wound healing by producing other signaling molecules for the formation of granulation tissues. However, PDGFs appear to have little effects on the formation of the dentin-like nodule in dental pulp stem cells isolated from rat lower incisors although PDGF-AB and -BB isoforms stimulate the expression of dentin sialoprotein (DSP) [65]. PDGFs stimulate cell proliferation and dentin matrix protein synthesis but appear to inhibit alkaline phosphatase (ALP) activity in dental pulp stem cells in culture [66–68]. DSP expression is inhibited by PDGF-AA, but enhanced by PDGF-AB and PDGF-BB although the mineralized tissue formation is inhibited, suggesting diverging effects of PDGFs on odontoblastic differentiation depending on dimeric form [65]. PDGF senhance the proliferation of fibroblasts in human dental pulp [66]. PDGF-BB may increase the expression of VEGF in osteoblasts and promotes angiogenesis at the site of dental pulp injury [69]. In vivo, PDGF promotes de novo formation of dental-pulp-like tissues in endodontically treated human teeth that are implanted in rats [28, 70].
Transforming Growth Factor-β (TGFβ)
Transforming growth factor β (TGFβ) is among the most widespread and versatile cytokine and plays an important role in the formation and development of many types of tissue [30]. There are more than 30 proteins, which belong to the TGFβ-superfamily such as TGFβ itself, bone morphogenetic proteins (BMPs), growth and differentiation factors (GDFs), Anti-Mullerian hormone (AMH), activins and nodal [28, 71, 72].
TGFβ is composed of ~390 amino acids, which are released mainly from platelets, macrophages, and bone [73]. This inactive polypeptide undergoes proteolytic cleavage to create the active C-terminal 112-amino acid form. The active form of TGFβ dimerizes to form 25 kD homodimers [74]. The name transforming growth factor is derived from the fact, that TGFβ belong to a group of growth regulators, that were originally detected in tumour extracts and thought to induce or maintain the neoplastic or transformed cell type [75]. Although TGFβ suppresses proliferation of epithelial cells, it is overexpressed in a number of epithelial malignancies.
The three isoforms present in mammals, which are TGFβ1, TGFβ2, TGFβ3, are detected also in human dentin [76].
The effect of TGFβ is highly variable and dependent on the type of cells and tissues. TGFβ1 regulates a wide range of cellular activities, such as cell migration, cell proliferation, cell differentiation, and extracellular matrix synthesis [28, 77–81]. TGFβ1 has been shown to increase cell proliferation and production of the extracellular matrix in dental pulp tissue culture [81], and promotes odontoblastic differentiation of dental pulp stem cells [82]. The effect of TGFβ1 can be synergistically upregulated by fibroblast growth fatocr-2 (FGF2), as evidenced by the increased ALP activity, the formation of mineralized nodule, and the expression of DSP and dentin matrix protein-1 [82]. The dentinogenic ability of dental pulp stem cells in the mechanically exposed dental pulp of dog teeth is shown to be induced by exogenous TGFβ1 [83]. TGFβ is chemotactic on dental pulp stem cells in vitro [84] TGFβ1 also plays an important role in the immune response during the dental pulp injury [28, 85, 86].
Bone Morphogenetic Protein (BMP)
Bone morphogenic proteins (BMPs) are homodimeric proteins of approx. 30 kD with the two identical strands linked by a cysteine binding group [30]. Nearly 20 modifications of BMPs with slightly different modifications in small secondary structure elements [87] have been identified so far [88], among which growth differentiation factors (GDFs) were included. The content of BMPs in bone has been estimated to be 1 μg/g bone tissue[30]. BMP2–BMP9 belong to the TGFβ superfamily with a high degree of homology with the TGFβs [30]. TGF β and BMP have a common scaffold with the cysteine knot motif and two double-stranded beta sheets [87]. BMPs activity is mediated through a transmembraneous heteromeric receptor complex on the cell surface that is identical to that of TGFβ. The difference in signalling pathway is characterized by the SMAD proteins that are activated by BMPs [30].
BMPs play important roles in the development and remodeling of the bone, and they can promote the chemotaxis and aggregation of cells into osteogenic site in different ways and facilitate the differentiation into osteoblasts. In addition, these proteins can also promote the angiogenesis, regulate the activity of some growth factors and affect the production of these growth factors, which is helpful for the osteogenesis. BMPs have been considered as the most potent growth factors that can promote the bone regeneration. To date, more than 20 BMPs have been identified and BMP-2, -4, -6 and -7 [osteogenic protein 1 (OP1)] have found to the osteogenic potential [89–93]. BMP9 is also known as growth differentiation factor 2 (GDF-2) and mainly expressed in the liver [94]. BMP9 can induce and maintain the cholinergic differentiation of embryonic neurons, regulate the metabolism of glucose and fatty acid, modulate the dynamic balance of iron and exert other important biological functions [95–97]. However, the role of BMP9 in the osteogenesis and bone regeneration is poorly understood.
Human recombinant BMP2 stimulates the differentiation of dental pulp cells into odontoblasts [98], inducing mRNA expression of dentin sailophosphoproteins (DSPPs) and higher ALP activity upon BMP2 application, but no effect on cell proliferation. DSPP expression and odontoblastic differentiation are regulated likely via BMP2-induced nuclear transcription factor Y signaling [99]. BMP2 also stimulates the differentiation of dental pulp stem cells into odontoblasts in vivo and in vitro [100]. Bovine dental pulp cells treated with BMP2 and BMP4 differentiate into preodontoblasts [101]. BMP7, also known as osteogenic protein-1, promotes dentin formation when placed over amputated dental pulp in macaque teeth [102, 103]. The dentinogenic effect of BMP7 on amputated dental pulp has been shown in several animal models including rats [104], ferrets [105], and miniature swine [106]. Dental pulp cells transfected with BMP11, also known as GDF11, yields mineralization [107]. Dentin matrix protein 1, ALP, DSPP, enamelysin, and phosphate-regulating gene are highly expressed in BMP11-tranfected cells [107]. Transplantation of BMP11-transfected cell pellets induces formation of dentin-like tissue on amputated dental pulp in dogs [107]. Ultrasound-mediated gene delivery of BMP11 stimulates odontoblastic differentiation of dental pulp stem/progenitor cells in vitro and reparative dentin formation in vivo [108].
Detection of alkaline phosphatase (ALP) and calcium deposition showed dental follicle stem cells transfected with BMP9 gene could significantly promote the osteogenesis. In addition, SB203580 and PD98059 were employed to block the p38 mitogen-activated protein kinase (p38MAPK) and extracellular signal-regulated kinase (ERK1/2), respectively. Detection of ALP and calcium deposition revealed the BMP9 induced osteogenic differentiation of dental follicle stem cells depended on MAPK signaling pathway [109].
BMP9 can effectively induce odontogenic differentiation of the stem cells from mouse apical papilla (SCAPs). Using a reversible immortalization system expressing SV40 T flanked with Cre/loxP sites, it was demonstrated that the SCAPs can be immortalized, resulting in immortalized SCAPs (iSCAPs) that express mesenchymal stem cell markers. BMP9 upregulates Runx2, Sox9, and PPARc2 and odontoblastic markers, and induces alkaline phosphatase activity and matrix mineralization in the iSCAPs. The in vivo stem cell implantation studies indicate that iSCAPs can differentiate into bone, cartilage, and, to lesser extent, adipocytes upon BMP9 stimulation. Results demonstrate that the conditionally iSCAPs not only maintain long-term cell proliferation but also retain the ability to differentiate into multiple lineages, including osteo/odontoblastic differentiation [110].
Fibroblast Growth Factor (FGF)
Fibroblast Growth Factor (FGF) plays key roles in cell migration, proliferation and differentiation during embryonic development [111] and wound healing [112]. Currently 22 members have been identified in humans [113], of which FGF2 appears to be significant in regeneration of the pulp-dentin complex [28]. FGF2 is a basic FGF (bFGF), while FGF1 is acidic. Basic FGF (bFGF) is known to be a critically important factor for maintaining the self-renewal ability of human embryonic stem (hES) cells in cultures and is a potent mitogen for cultured neural progenitors [114, 115].
bFGF dramatically induces the mRNA expression of dentin sialophosphoprotein (DSPP) and bone sialoprotein (BSP) in immature adult rat incisor dental pulp cells [116], suppresses alkaline phosphatase (ALP) activity and osteogenesis differentiation in human dental pulp cells (hDPCs), and inhibits osteogenesis in mouse adipose tissue-derived stromal cells, whereas bFGF sustains their proliferative and osteogenic potential state [117–119]. bFGF induces the migration of dental pulp stem cells [120]. Using a transwell migration assay, significantly more dental pulp cells are recruited by bFGF (FGF2) into a 3D collagen gel than controls without cytokines and BMP7 [120]. bFGF also stimulates the proliferation of dental pulp stem cells without differentiation, whereas bFGF combined with TGFβ1 induces differentiation of dental pulp stem cells into odotoblast-like cells, and synergistically upregulates the effect of TGFβ1 on odontoblast differentiation [82].
bFGF stimulation in the osteogenic differentiation period decreased the in vitro osteogenic differentiation ability of DPSCs. One week pre-treatment with bFGF increased the in vitro osteogenic differentiation ability of DPSCs, whereas 2 weeks pre-treatment with bFGF decreased the in vitro osteogenic differentiation ability of DPSCs. The pre-treatment period was vital for the osteogenic differentiation of DPSCs in vitro. The in vivo results were similar to the in vitro results. bFGF affected the osteogenic differentiation of DPSCs in a treatment-dependent manner both in vitro and in vivo [121].
In vitro studies showed that different timing of the presence of bFGF might either suppress osteogenic/dentinogenic differentiation of SCAP or enhance osteogenic/dentinogenc differentiation [122]. These findings indicate that the effects of bFGF are differentiation stage specific and that bFGF might modulate cell differentiation by acting at distinct stages of cell maturation [123]. Under certain conditions, therefore, bFGF enhances SCAP stemness by up-regulating stem cell gene expression, increasing proliferation ability, and potentiating differentiation potency [122]. Results showed that short-term bFGF treatment enhanced OCT4, REX1, and NANOG mRNA expression as well as colony forming unit ability in stem cells isolated from human exfoliated deciduous teeth (SHEDs) . The FGFR inhibitor pretreatment was able to attenuate the influence of bFGF on pluripotent stem cell marker expression, confirming bFGF function. In addition, cells cultured in high passage number had decreased in cell proliferation, colony forming unit capacity, and pluripotent stem cell maker mRNA expression. However, bFGF supplementation in culture medium enhanced both pluripotent stem cell marker expression and colony forming unit capacity in later passage, though the effect was not robust. Together, these results indicate that high passage number may attenuate pluripotent properties of SHEDs and bFGF supplementation could be the beneficial approach to maintain SHEDs’ stemness properties [124].
Insulin-Like Growth Factor (IGF)
Insulin-like growth factors (IGFs) are single chain peptides that exist in two isoforms (IGF-I (70 amino acids) and IGF-II (67 amino acids)). IGFs have approximately 40–50 % homology between themselves and with insulin. Despite this significant homology between insulin and IGFs, all three have unique binding sites to their receptors [125]. In contrast to many other growth factors, which act merely on a local or regional level, IGFs are also factors with a general both metabolic and growth promoting activity in many cell and tissue types. IGFs , comprising IGF-1 and IGF-2, contribute to odontogenesis and dental tissue repair by cell proliferation and differentiation. [28, 126]. Of the two isoforms, IGF-1, also known as somatomedin C, has potency in growth and differentiation of dental pulp cells [127]. IGF-1 induces proliferation and differentiation of dog dental pulp cells into odontoblast-like cells in serum-free medium [127]. IGF-1 with PDGF-BB has a synergistic effect on the proliferation of dental pulp cells in vitro [128]. IGF-1 and IGF-1R have a higher level of expression in dental pulp tissue from teeth with complete root development than teeth with incomplete root formation, suggesting that IGF-1 stimulates mineralization and cell differentiation [128–130]. IGF-1 could promote proliferation and osteogenic differentiation of human dental pulp stem cells (DPSCs) via mTOR pathways, which might have clinical implications for osteoporosis [131].
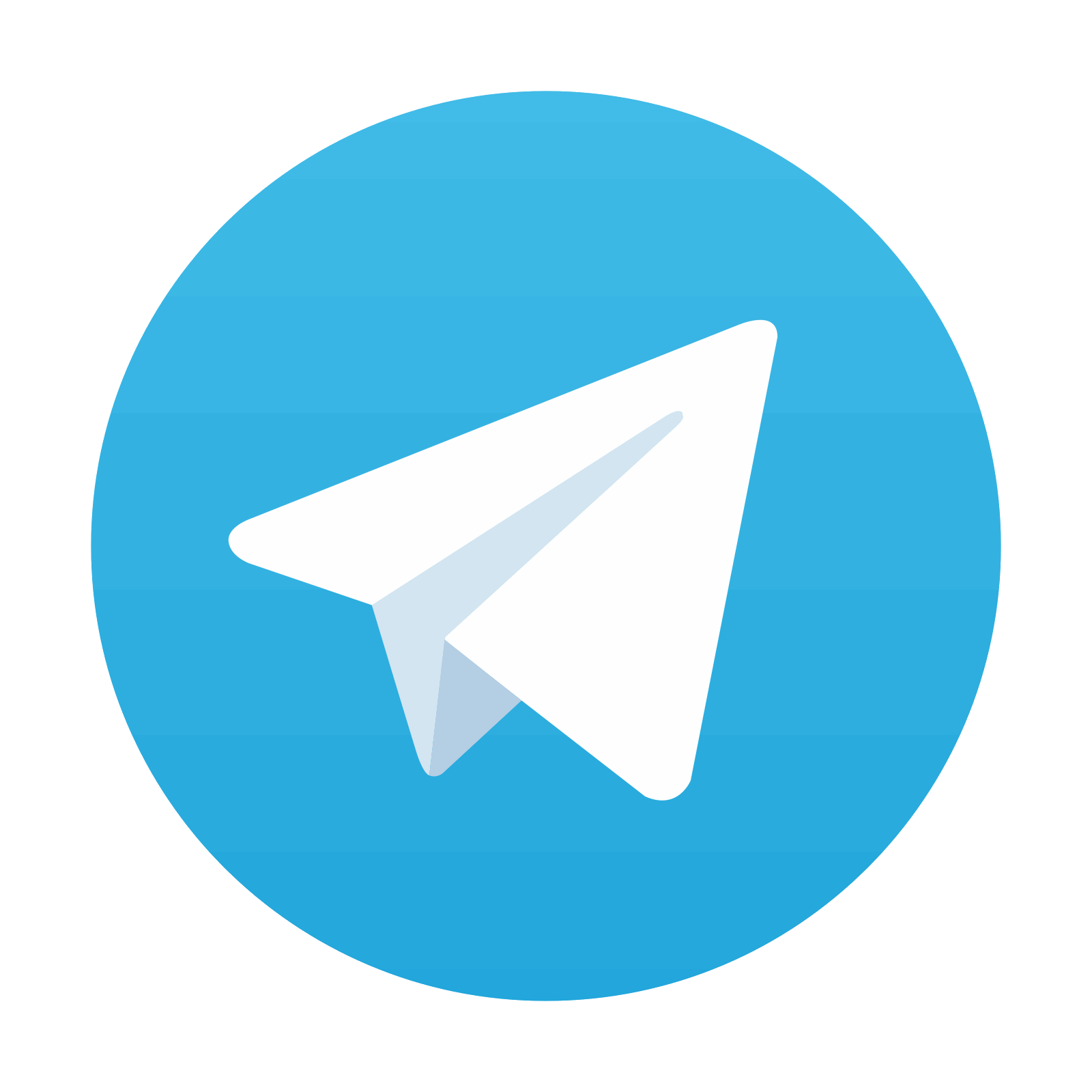
Stay updated, free dental videos. Join our Telegram channel

VIDEdental - Online dental courses
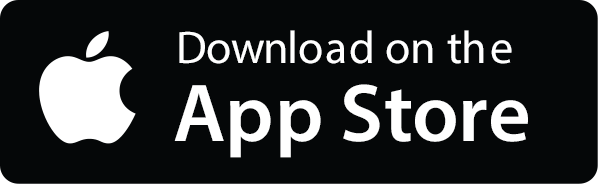
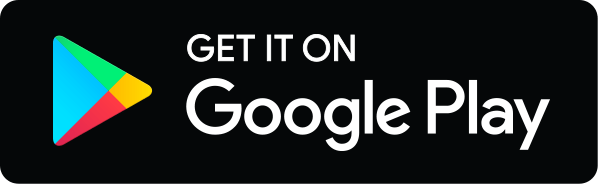
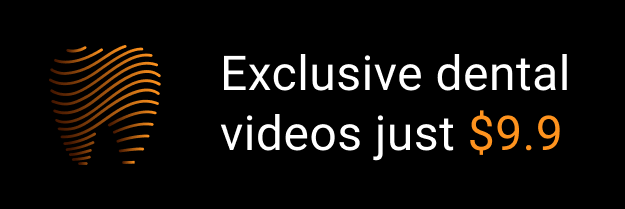