© Springer International Publishing Switzerland 2016
Barbara Zavan and Eriberto Bressan (eds.)Dental Stem Cells: Regenerative PotentialStem Cell Biology and Regenerative Medicine10.1007/978-3-319-33299-4_9
Stem Cells for Periodontal Regeneration
(1)
Private Practice, Via Lamarmora 29, 50121 Florence, Italy
(2)
University of Milan, Milan, Italy
Keywords
Periodontal diseasePeriodontalTissue engineeringDental stem cellsPeriodontal ligament stem cells
Background
The Importance of Maintaining an Adequate Oral Health Status
A poor oral health status has been associated with systemic diseases, such as diabetes and risk factors for oral diseases, such as tobacco use, are shared with other systemic diseases. Poor oral health also impacts diet and nutrition and affects social activities, such as school and work [1]. Significant disadvantages are consequently associated with oral diseases and tooth loss.
As an indirect indicator of oral health conditions, we know that since the 1970s, fewer adults have experienced tooth loss and the prevalence of complete edentulism among adults has been consistently declining; however, important variations remain among subgroups of the population. Age is strongly related to tooth loss, gender is not related after adjustment for age, and race-ethnicity is consistently after adjustment for age and gender [1]. While the prevalence of edentulism continues to decline, the rate of aging of the western civilization and the longer life expectancy will keep increasing the need for dental treatment in order to prevent tooth loss.
Periodontal Diseases
Periodontal diseases include a variety of pathologies affecting the supporting structures of teeth and eventually leading to tooth loss. Periodontal disease affects almost half of the population in western countries [2] and severe periodontitis is the sixth most prevalent disease of mankind [3]. More than 70 % of the population over 65 years old is affected by periodontal disease. The prevalence is higher in males than females and highest in Mexican–Americans than in African–Americans and in African–Americans in relation to Caucasians. In smokers the prevalence of periodontal disease is 64.2 %. Research has shown that periodontal disease is associated with other chronic inflammatory diseases as diabetes and cardiovascular disease [2]. Periodontitis is also one of the main causes of tooth loss followed by tooth decay [4–6].
Chronic Periodontitis, Aggressive Periodontitis, Periodontitis as a Manifestation of Systemic Diseases, Necrotizing Periodontal Diseases, Abscesses of the Periodontium, Periodontitis Associated With Endodontic lesions and Other Developmental and Acquired Deformities are the main groups of pathologies possibly affecting the attachment apparatus [7]. All these conditions may have different etiology and contributing factors, but they all share loss of clinical attachment as the main characteristic bringing them together. Once the etiological and contributing factors are controlled the clinician often finds himself in the difficult position of correcting the deformities caused by the disease in order to facilitate oral hygiene maintenance and reducing the chance for disease recurrence and tooth loss [8].
Challenges in Periodontal Tissue Regeneration
The tooth attachment apparatus is a complex organ including cementum, periodontal ligament (PDL) and boundle bone. Embryologically speaking, all these structures have ectodermal origin making them different in relation to bone tissues normally originating from the mesoderm, later on we’ll discuss on the possible importance of this different origin. The cementum is a thin layer of a mineralized tissue covering the tooth root dentin and in which the PDL fibers are inserted. The PDL is the tooth shock absorber system. It is made of collagen fibers called Sharpey’s fibers but it also includes vessels and nerves bringing nourishment and Proprioceptivity to the surrounding structures. The boundle bone is the layer of bone, which is closer to the root surface. Sharpey’s fibers are inserted in it, which make it different in relation to the alveolar bone. Many teeth positioned outside the alveolar bone housing are covered by boundle bone only and not any alveolar bone [9].
When attachment loss occurs, the PDL and boundle bone are resorbed by tissue enzymes and the cementum is left of the tooth surface and covered by bacterial plaque and calculus [10, 11]. Removal of plaque and calculus results in the closure of the periodontal pocket via formation of a long junctional epithelium [12]. Other traditional surgical approaches showed different repair models.
In 1976 Melcher [13] hypothesized that the repair model was dictated by the proliferation speed of different cell populations. In other words once the surgical trauma occurred and bacterial plaque was removed, a competition between soft tissue cells and hard tissue cells would take place. Being epithelial cells the fastest they would colonize the periodontal defects first.
Nyman and coworkers decided therefore to exclude soft tissue cells from this competition by attempting the first human case of guided periodontal tissue regeneration using a Millipore filter between the flap and the previously diseased root surface [14]. They showed, for the first time in humans, the regeneration of all of the three attachment apparatus structures: cementum, a functionally oriented PDL and boundle bone. After their pioneering work several authors have researched on guided tissue regeneration procedures.
Following studies shifted the attention from the competition theory to the importance of blood clot stabilization: that is that if the adhesion between the blood clot and the root surface is preserved epithelial cells would not infiltrate between the clot and the root surface resulting in periodontal regeneration [15, 16].
Periodontal Regeneration: State of the Art
Modern approaches to periodontal regeneration demonstrated better clinical results when adopting surgical techniques minimizing surgical invasiveness by reducing flap extension and mobilization and preserving the soft tissues as much as possible. Papilla preservation techniques were first introduced in 1985 [17] and then refined in 1995 [18], 1999 [19] and 2001 [20]. Later on surgical techniques using a single flap approach were introduced [21–24] and other flaps boosting the biological performance of blood clot stabilization have been developed [25].
Biomaterials in Periodontal Regeneration
Following Nyman’s first guided tissue regeneration case with a Millipore filter several biomaterials have been evaluated with the intent of enhancing the clinical performance of periodontal regeneration surgeries.
Barrier membranes have been developed attempting to maintain soft tissue separated from the underlying structures during wound healing. Non-resorbable e-PTFE membranes were widely adopted either with or without a titanium frame reinforcement. Soon after resorbable membranes were introduced to overcome the need for a second surgical intervention to remove the e-PTFE membrane. Synthetic materials as polylactic acid, polyglycolic acid and combinations or xenogenic collagen membranes with different resorption rates have been used and evaluated widely in recent decades. Other than the barrier effect, membranes maintain the capability to support and stabilize the underlying tissues thus improving the clinical outcome of the regeneration procedure.
Autogenous bone and bone substitutes as synthetic materials, xenogenic bone substitutes and allogenic bone substitutes such as freeze-dried bone allograft (FDBA) and demineralized freeze-dried bone allograft (DFDBA) have also been thoroughly evaluated. Autogenous bone has been considered the gold standard as it maintains all the characteristics required for a grafting material: a scaffolding effect, an osteoconductive effect, presence of inductive proteins as the Bone Morphogenic Proteins capable of inducing the differentiation of neighboring undifferentiated cells toward the osteoblast pathway (osteoinduction) and the presence of cells which could directly initiate bone formation (osteogenic capability). Other bone substitutes have demonstrated good osteoconductive capabilities but only DFDBA has shown limited osteoinductive capabilities.
The clinical efficacy of membranes and biomaterials including autogenous bone has been widely documented when using extended flaps. With the advancements in flap manipulation and surgical techniques the use of biomaterials have been questioned in contenitive defects as their impact on clinical performance may be limited [26, 27]. Biomaterials are still considered important when treating larger non-contenitive defects. Moreover, tissue engineering approaches are currently evaluated in order to facilitate, enhance and speed up periodontal regeneration and possibly make it more predictable even when treating more challenging defects.
Tissue Engineering in Periodontal Tissue Regeneration
Tissue engineering is a broad term that describes very different applications. In a comprehensive manner MacArthur and Oreffo defined it as the understanding of the principles of tissue growth, and its application to produce functional replacement tissue for clinical use [28]. In its common view though tissue engineering is closely associated with the repair or regeneration of portions or whole tissues. In the medical field its weight is directly related to the importance that the ability of regenerating an organ or a tissue has to the survival or quality of life of an individual. In dentistry tissue engineering techniques find practical applications in the regeneration of lost bone, periodontal ligament, dental pulp or keratinized mucosa.
Regenerative strategies can be categorized in three major classes: Conductive approaches utilize biomaterials in a passive manner to facilitate the growth or regenerative capacity of existing tissue (guided tissue regeneration in periodontal dentistry is an example of this approach). Another tissue engineering strategy is Induction. The original concept lying behind this mechanism was explored with the discovery of bone morphogenetic proteins (BMPs) , molecules that are able to promote the differentiation of uncommitted mesenchymal cells through the osteoblastic pathway inducing the formation of bone even in an ectopic (nonmineralizing) tissue. Cell transplantation is the third class of tissue engineering strategies. The collaboration of surgeons and bioengineers is critical for the delivery of cells that will interface with the host cells for the regeneration of the new natural tissue [29].
As previously mentioned most bone substitute materials used nowadays are adopted for their conductive capability in the regeneration of periodontal tissues; other inductive approaches as protein therapy and gene therapy and of cell transplantation have been evaluated and are currently studied in the attempt to generate better grafting solution for periodontal regeneration.
Protein Therapy: Growth Factor Delivery
The incorporation of bioactive molecules as Growth Factors (GFs) into scaffolding materials has been used to stimulate precursor cells and enhance wound healing and bone formation. The concept lying behind the use of GFs is related on the knowledge about cell-to-cell and platelets-to-cells biochemical interactions during wound healing.
A variety of factors have been studied that we can mainly group into two categories: growth factors act primarily through increasing cell proliferation (mitogenic) and recruitment of cells (chemotactic) while morphogens act through the alteration of cellular phenotype. BMPs are an example of morphogens; they have one of the most outstanding inductive properties in tissue regeneration: the ability of induce the differentiation of stem cells into bone formatting cells in a process known as osteoinduction [30]. We will now focus on proteins that have shown the most promising result in periodontal and implant related tissue engineering.
Platelet-Rich Plasma
The first attempt of using growth factors in tissue regeneration was by the use of platelet-rich plasma commonly known with its acronym: PRP.
Platelets are one of the body’s best sources of growth factors. When activated they release α-granules containing, between others, Platelet Derived Growth Factor (PDGF) , Transforming Growth Factor-β (TGF-β) Epidermal Growth factor (EGF) and Endothelial Cell Growth Factor (ECGF) . These factors initiate several pathways during wound healing in all tissues. PRP was originally used for treatment of diabetic ulcers, decubitus ulcers, treatment of sternal wounds after cardiac surgery, non-healing fractures and it was then introduced its use in the dental field in 1998 [31]. PRGF is a technique similar to the production of PRP using although only one centrifugation and depleting the plasma from all red blood cells [32]. Other techniques have been described with significant variations in centrifugations protocols and final product indications.
A review by Boyapati and Wang critically evaluated the available literature on animal and human application of PRP. The authors concluded that due to the paucity of critical scientific data regarding the effects of PRP in sinus augmentation procedures and the poor study design of the available trials the use of PRP cannot be supported in sinus augmentation procedures [33]. A systematic review from Plachokova et al. came to similar conclusions about the materials and methods of the analyzed articles. When considering periodontal tissue regeneration although the results seem to be a little more encouraging [34].
With centrifugation techniques increases in platelet concentration between 2 and 10 times can be achieved. Protein therapy approaches have been introduced which are able to provide growth factors concentrations of thousands times higher than that carried by the blood.
Platelet Derived Growth Factor (PDGF )
Platelet-Derived Growth Factor (rh PDGF-BB) is one of the numerous growth factors, or proteins that regulate cell growth and division. In particular, it plays a significant role in blood vessel formation (angiogenesis), the growth of blood vessels from already existing blood vessel tissue. Mitogenic and chemotactic effects have been shown on calvarial osteogenic cells, periosteal cells from long bones, trabecular bone cells and bone marrow stromal cells. PDGF also increases expression of angiogenic molecules such as VEGF, hepatocyte growth factor/scatter factor, and of inflammatory factors as IL-6. PDGFs also increase osteogenic cells’ responsiveness to BMPs [30].
The use of PDGF-BB in combination with other growth factors as the insulin-like Growth Factor-1 (IGF-1) has shown increased bone density and alveolar bone formation when compared to controls in periodontal defects and increased bone to implant contact (BIC) when used around implants immediately placed in extraction sockets [35–38]. When used in combination with allograft it has also shown encouraging results on the treatment of Class-II furcations and infrabony interproximal pockets [39, 40]. Recombinant human platelet-derived growth factor (rhPDGF-BB) mixed with a synthetic beta-tricalcium phosphate (beta-TCP) has been favorably used in the treatment of periodontal osseous defects [41, 42].
Although good results have been showed in eliciting bone regeneration some authors have hypothesized that suboptimal tooth-supporting tissue regeneration is related to the short half-life of PDGF in vivo. This could be a result of proteolytic degradation, rapid diffusion, or the solubility of the delivery matrix within periodontal lesions [43]. Increasing the substantivity of the molecule in vivo may result in improved periodontal regeneration.
Bone Morphogenetic Proteins (BMPs)
Bone morphogenetic proteins (BMPs ), members of the transforming growth factor-β superfamily, have been shown to be responsible for post-fetal bone induction, including normal bone remodeling healing and repair. BMPs have been shown to be able to induce heterotopic bone formation [44]. Although all BMPs are involved in bone and cartilage formation by stimulating cellular events and mesenchymal progenitor cells, only a subset of BMPs (i.e. BMP-2, -4, -7 and -9) has osteoinductive properties [45]. New bone growth following use of BMPs results from the differentiation of pluripotent mesenchymal cells along osteoblastic pathways [46]. RhBMP-2 in combination to an absorbable collagen sponge have been tested in maxillary sinus elevation and for local alveolar ridge preservation or augmentation [36, 37, 46–48]. Lee et al. have tested ceramic barriers impregnated with BMP-2 greatly to improve craniofacial bone regeneration in vivo [49]. Bone repair has been documented in periodontal defects when using rhBMP-2/ACS although ankylosis was a frequent observation and only occasionally was a regeneration with functionally oriented PDL fibers observed [50]. Bone morphogenetic protein-7 (BMP-7), is a multifunctional member of the BMP family with multiple effects on bone formation and regeneration. BMP-7 stimulates bone regeneration around teeth [51], around endosseous dental implants [52], and in maxillary sinus floor augmentation procedures [53, 54]. Wikesjo reported in an animal study that while rhBMP-2/ACS is able to induce more robust bone regeneration rhBMP-12/ACS can induce more proper PDL regeneration with periodontal fibers inserting perpendicularly to the root surface into the newly regenerated cementum and supporting bone [55].
One of the limitations related to the use of BMPs in oral applications is the superphysiological dose of BMPs implanted in the wound site in certain trials resulting in a dose-dumping of potent morphogens [47, 48, 54]. There is a concern that this delivery method could lead to systemic toxicity in example by diffusion of potent morphogens through the bloodstream. The effect and safety of BMPs in vivo may benefit from a more controlled delivery method able to provide optimal concentration of these molecules through an adequate period of time.
Gene Therapy
In vivo growth factors have a transient effect since the half-life of these molecules is in the order of minutes to hours. Therefore, the induced production of growth factor via gene transfer is could potentially increase the effect of GFs by releasing them in a sustained manner. The latter is called Gene therapy and consists of the insertion of genes into individual’s cells either directly or indirectly with a matrix to promote a specific biological effect. Gene therapy typically aims to supplement a defective mutant allele with a functional one in a therapeutic approach but can also be used to induce a more favorable host response. Targeting cells for gene therapy requires the use of vectors or direct delivery methods to transfect them [56]. Tissue engineering of alveolar bone using gene therapeutic approaches may offer potential for optimizing the delivery of growth-promoting molecules such as bone morphogenetic proteins (BMPs) at implant osteotomy sites [43, 57].
The most commonly employed vectors for gene transfer are viruses as retrovirus, lentivirus, adenovirus or adeno-associated virus [58]. A possible concern of the use of viral vectors is the possibility of an accidental generation of replication competent virus during vector production and packaging, the mobilization of the engineered vector by endogenous retrovirus present in the human genome and the random insertion or mutagenesis of the vector leading to cancer or germ cell alteration resulting in vertical inheritance of the acquired genes [59].
Non-viral vectors include plasmid DNA and synthetic or natural polymers. These vectors are safer than the viral vectors but they have low and unpredictable gene-transfer efficiency and allow only a limited size of DNA insert to penetrate in the targeted cells.
Both viral and non-viral vectors can be used in in vivo or ex vivo. In vivo approaches require the delivery of the vector directly into the host where the gene-transfer will happen. This method is less safe, more unpredictable but less invasive than ex vivo approaches. In ex vivo applications the gene-transfer happens in vitro of previously harvested host cells or in cell lines. The efficiency of the gene-transfer can be controlled and the infected cells are then re-implanted in the host. Ex vivo gene therapy has been widely adopted in combination to stem cell therapy.
In vitro studies have shown promising results when using recombinant adenoviral vectors encoding for the PDGF-A gene on cloned cementoblasts [60]; Chen and Giannobile evaluated the effects of PDGF on cell signaling via gene therapy when applied to tissue engineering of skin and periodontal wounds. The results of this study showed a sustained activation of the transfected genome by ligand binding resulting in a prolonged production of PDGF-AA and a sustained stimulation of several signaling pathways [61]. Syngenic dermal fibroblasts (SDFs) transduced ex vivo with adenoviruses were transplanted into large mandibular defect in a rat model with significantly improved results compared to controls [62]. Sustained and targeted delivery of BMP-7 to large alveolar bone defects associated with dental implant fixtures in a rat model also demonstrated that in vivo gene transfer promotes bone regeneration [53].
In a rat model periodontal defects where treated with either a collagen matrix alone or with a matrix containing adenoviruses encoding luciferase, PDGF-A (PDGF-1308) or (4) PDGF-B. At 14 days the control groups showed limited bone bridging while a fourfold increase in bridging bone and sixfold increase in tooth-lining cemental repair was observed the Ad-PDGF-B-treated sites that presented greater evidence of vascularization [63].
Chang et al. demonstrated that the use of adenovirus encoding the PDGF-B gene (AdPDGF-B) delivered in a collagen matrix to periodontal lesions in rats exhibited acceptable safety profiles; AdPDGF-B was well contained within the localized osseous defect area without viremia or distant organ involvement [64]. The same group evaluated the efficacy of the gene therapy approach in two concentrations of Ad-PDGF-B, a control Ad encoding luciferase or the protein therapy with rhPDGF-BB. Bone repair was found to be accelerated by gene and protein therapy compared with control, with the high dose of Ad-PDGF-B more effective than the low dose. Interestingly the protein therapy approach was found to be more effective than the gene therapy approach. Typically, high concentrations of growth factors are required to promote tissue regeneration [65]. A possible explanation for this unexpected result is that the concentrations of growth factor released during healing in the Ad-PDGF-B treated sites remained below the effective levels of the rhPDGF-BB treatment.
Cell Therapy
To summarize a few concepts described above we can identify the needs for tissue regeneration in the following categories: cells, growth factors, scaffold, and new vessels [56]. In conventional regeneration therapies cells need to reach the area where the new tissue has to be created from the periphery of the grafted site. Scaffolds are used both as a support for their migration and as a frame for cells to start building up the tissue matrix. The migration and the differentiation of the cells is regulated and stimulated by growth factors that modulate the cellular activity and provide stimuli to cells to differentiate and produce matrix toward the developing tissue. GFs also stimulate the production of angiogenic signals inducing the formation of a new vascular network, which is essential for working cells as a source of nutrients and oxygen and as a pathway for the removal of catabolites.
Cell therapy approaches provide an additional source of cells in the area of interest with the intent to be used as grafted cells (which will integrate into the patients body) or, when not intended for integration, as a source of growth factors.
Both cultivated somatic cells and stem cells have been studied and tested in different branches of contemporary medicine and periodontology. Dogan et al. seeded cells derived from R to promote the regeneration in artificial furcation defects of a dog model and found this method to be superior than controls [66]. Cultivated fibroblasts have also been safely used for the treatment of interdental papillary insufficiency following a papilla priming procedure by McGuire and coworkers with satisfactory results [67]. Cell therapy has also been tested in mucogingival surgery [68–73]. Cloned cementoblasts have also demonstrated potential in periodontal therapy [74, 75]. Skin fibroblasts transduced by the BMP-7 gene promoted the tissue engineering of periodontal bone defects including new bone, functional PDL and tooth root cementum [62].
Stem Cells
Stem cells are cells that maintain an increased potential for renewal and differentiation in relation to cultivated cells. The benefit in the use of stem cells is that once transplanted in the grafted site they may differentiate in strictly osteogenic cells as well as in “supportive osteogenic cells”. Supportive osteogenic cells are defined, as cells that do not directly create bone but that facilitate bone depositions creating structures that are needed to allow this process (i.e. vascular network) [76, 77]. Mesenchymal stem cells also play a role in preventing inflammation in the grafted site [78] allowing faster regeneration of new tissues.
Mesenchymal stem cells (MSCs) were first discovered in bone marrow and then in other adult tissues such as liver, adipose tissue, and muscle, but the marrow stroma remains the most widely used sources of stem cells [79]. They are characterized by elevated renewal potency and the ability of growing bone, cartilage, midollar adipocytes, miocytes, fibrous tissues from a single colony forming unit-fibroblast (CFU-F) when transplanted in vivo [80]. In the differentiation pathway, limitative or inductive differentiation stimuli may lead to cells progressively characterized by lower renewal capacity and by an augmented degree of differentiation [79]. This path can be reversed so that an adult adipocyte may de-differentiate back to levels with higher generative capabilities and then differentiate through the osteogenic pathway [81].
Given the inconvenience of obtaining stem cells from adult tissues including the use of invasive harvesting techniques, researchers have succeeded in harvesting mesenchymal stem cells from dental-derived tissues. Dental Stem Cells (DSCs) are adult stem cells derived from dental-related tissues such as the periodontal ligament of extracted teeth [82], gingiva [83], dental follicles [84], dental pulp [85], apical papilla [86], and human exfoliated deciduous teeth [87, 88].
Dental stem cells populations still maintain similar properties as bone marrow derived stem cells [85, 89–96]. Although they are reported to derive from the neural crest during tooth development [90], in mature individuals they demonstrate stem cells properties similar to MSCs rather than neural crest cells [97]. Jo and coworkers isolated stem cells from dental pulp, periodontal ligament, periapical follicle, and the surrounding mandibular bone marrow and found that they were able to differentiate into osteoblasts, adipocytes, and other kinds of cells with varying efficiency [98]. Because of their MSCs-like characteristics DSCs have been used in the regeneration of distant tissues and have been suggested as excellent candidates for cell-based therapy to treat liver diseases [99].
It has to be considered though that despite having similar properties each single stem cell type is different to the other. One important feature of dental pulp stem cells (DPSCs), in example, is their odontoblastic differentiation potential making them ideal to be seeded onto dentin and for dentin regeneration strategies. In endodontic therapy interesting results come from the autogenous transplantation of the BMP2-treated pellet culture of pulp progenitor/stem cells onto the amputated pulp. The treatment stimulated reparative dentin formation in a dog model [100]. Stem Cells from Human Exfoliated Deciduous Teeth (SHED) proliferate faster with greater population doublings than DPSCs and BMMSCs; unlike DPSCs, SHED are unable to regenerate a complete dentin-pulp-like complex in vivo. They showed to maintain the ability to differentiate into osteoblasts and induce bone formation, generate dentin and dental pulp [92, 101, 102]. Their ability to differentiate into bone-forming cells is not a property attributed to DPSCs following transplantation in vivo [90]. Similar to DPSCs and SHED, ex vivo expanded Stem Cells from Apical Papilla (SCAP) can undergo odontogenic differentiation in vitro. SCAP also demonstrate the capacity to undergo adipogenic differentiation following induction in vitro and show positive staining for several neural markers even without neurogenic stimulation [90, 103] and they are able to differentiate into functional dentinogenic cells. Periodontal Ligament Stem Cells (PDLSCs) seem to be the most promising dental derived stem cells for periodontal regeneration purposes as they can differentiate into either cementum-forming cells (cementoblasts) or bone-forming cells (osteoblasts) [104, 105]. They still demonstrate osteogenic, adipogenic, and chondrogenic characteristics under defined culture conditions [72, 73, 89, 106] but their capacity to form all of the PDL-related structures in vivo [82] makes of them the ideal candidates in periodontal regeneration [107]. In recent studies evaluating the osteogenic activity of different stem cell populations, the expression of RUNX2—a gene playing a crucial role in osteoblast differentiation [108]—peaked in BMSCs at day 14, while DPSCs and GSCs peaked at day 21 and PDLSCs peaked at day 28. The authors concluded that a possible explanation for the early RUNX2 expression in BMSCs may be that the embryological niche in the mesoderm area is more conductive for mineralization as compared to the ectodermal origin of PDLSC, GSC and DPSC [109, 110]. Moreover it has been shown that hPDLSC produce negative regulators of osteogenesis such as chordin and PDL-associated protein-1 or aspirin. In particular chondrin specifically binds to BMP-2 suppressing its osteoinductive capacity [111–115]. Despite this piece of knowledge may seem conflicting with the PDLSC task of regenerating a damaged periodontium, a physiological explanation may be hypothesized. Should a damaged PDL come in contact predominantly with bone MSCs in an osteoinductive environment ankylosis and root resorption have been documented [116]. The PDLSC ability to suppress osteoinduction may be needed to maintain normal tooth tissues homeostasis and prevent remodeling of the root.
From the information provided above it seems clear that despite their plasticity and flexibility not every stem cell is the same but one particular stem cell population may be more suitable to a particular task than another. Other cells showing capacity to form periodontal tissues are the Dental Follicle Precursor Cells (DFPCs) : progenitor cells that form the periodontium, i.e., cementum, PDL, and alveolar bone which have been isolated from human dental follicles of impacted third molars. Kémoun et al. demonstrated expression of cementum attachment protein and cementum protein-23 (CP-23), two putative cementoblast markers, in EMD-stimulated whole dental follicle and in cultured DFPCs stimulated with EMD or BMP-2 and BMP-7 [117].
Even within a single cell source differences may be greater than one would think. PDLSCs have been isolated from the alveolar socket following extraction, from the root surface after extraction [95, 96], from deciduous [118–120] or permanent teeth [118, 119], from supernumerary teeth [120] and from periodontal granulation tissue [121, 122] each source showed different characteristics and regenerative potential [123], therefore strict identification criteria should be used when reporting and comparing results from each particular cell type.
Other important consideration are related to the culture medium, the oxygen concentration during cell culturing or the combined use of growth factors, which may enhance or reduce their regenerative potential and modify their characteristics [123].
The use of dental derived stem cells in periodontal therapy has important advantages. First of all that, because of their origin, they may be more adequate in the regeneration of periodontal tissues as opposed to other MSCs as dental stem cells appear to be more committed to odontogenic rather than osteogenic development [90]. Secondly, when autologous therapy is considered, the same dentist providing treatment to the patient could also be harvesting the cells beforehand. The problem in periodontal therapy is that not always the patient requiring periodontal regeneration will also need tooth extraction so not all cell types may be available. Periodontitis defects need a large number of cells (about 107 cells for one defect), which we may not be able to obtain from a single subject when using autologous stem cells from oral tissues [124]. Also at the time of treatment most periodontal patients are not young and the potential of their stem cells may be reduced [125]. Luckily because of immunoregulatory potential of DPSCs [126] SCAP and PDLSCs [90] allogenic dental stem cell therapy may be feasible as demonstrated in animal models [127, 128] therefore stem cells collected from deciduous teeth or teeth extracted for orthodontic reasons in younger individuals may be used in older patients. This immunoregulatory capacity is interesting as it has been suggested that it may play a role in reducing the inflammatory reaction to biomaterials used in tissue regeneration thus stimulating faster bone formation [129]. Other solutions to improve stem cell availability could be the cryopreservation of one’s stem cells for future autologous use. Cryopreservation of extracted third molars was found to be an adequate and cost-effective method of preservation of MSC cells. After recovery, these cells maintained the characteristics of mesenchymal stem cells (ability to differentiate into osteogenic, adipogenic, and chondrogenic pathways) showing the possibility of banking MSC with minimal processing [130]. Finally, the immortalization of adult cells via gene therapy has been suggested [131] although raising questions on the costs and safety of these therapies.
On this path, adult adipocytes are known to be able to de-differentiated back to levels with higher generative capabilities and because of the large amounts of human lipoaspirates readily available, and the fact that their procurement induces only low morbidity, ASCs may be useful in future clinical cell-based therapy for periodontal disease. Adipose tissue is the richest source of MSCs, 100 times more than bone marrow [132]. The use of adipose-derived stem cells (ASCs) for periodontal tissue regeneration in vivo has been tested in animal models. These studies demonstrated the ability to promote tissue regeneration with this cell construct [133–136].
iPSCs may also represent an alternative source of stem cells. These are induced pluripotent stem (iPS) cells were first created reprogramming mice somatic cells through viral introduction of four transcription factors (Oct3/4, Sox2, Klf4, and c-Myc) from a group from Kyoto University [137]. iPS cells demonstrated to be completely similar to the most widely used Stem Cells and can be created reprogramming adult human dermal fibroblasts into a pluripotent state or practically any human somatic cell source [138]. IPSC lines were derived from human somatic cells reprogram somatic cell nuclei to an undifferentiated state [139, 140]. This technology may allow the implantation of Stem Cells without the drawbacks of the surgery required for their harvesting from the patient. Moreover, in alternative to reprogramming of patients’ somatic cells, encouraging results have been conducted towards the establishment of an iPS cell bank consisting of various human leukocyte antigen (HLA) types. Dental Pulp Cells (DPCs) could represent an excellent source for iPS cell banks using retroviral vector for delivery of the exogenous genes [141].
Once proved as safe and effective, the possibility to generate an HLA-type banking of human pluripotent stem cell (hPSC) lines would greatly increase cost-effectiveness and practicality with the medical and oral health care cell therapy delivery systems, as well as safety and quality control measures of these therapies. Studies should determine whether IPSCs are better than DSCs in terms of safety and efficacy in periodontal regeneration.
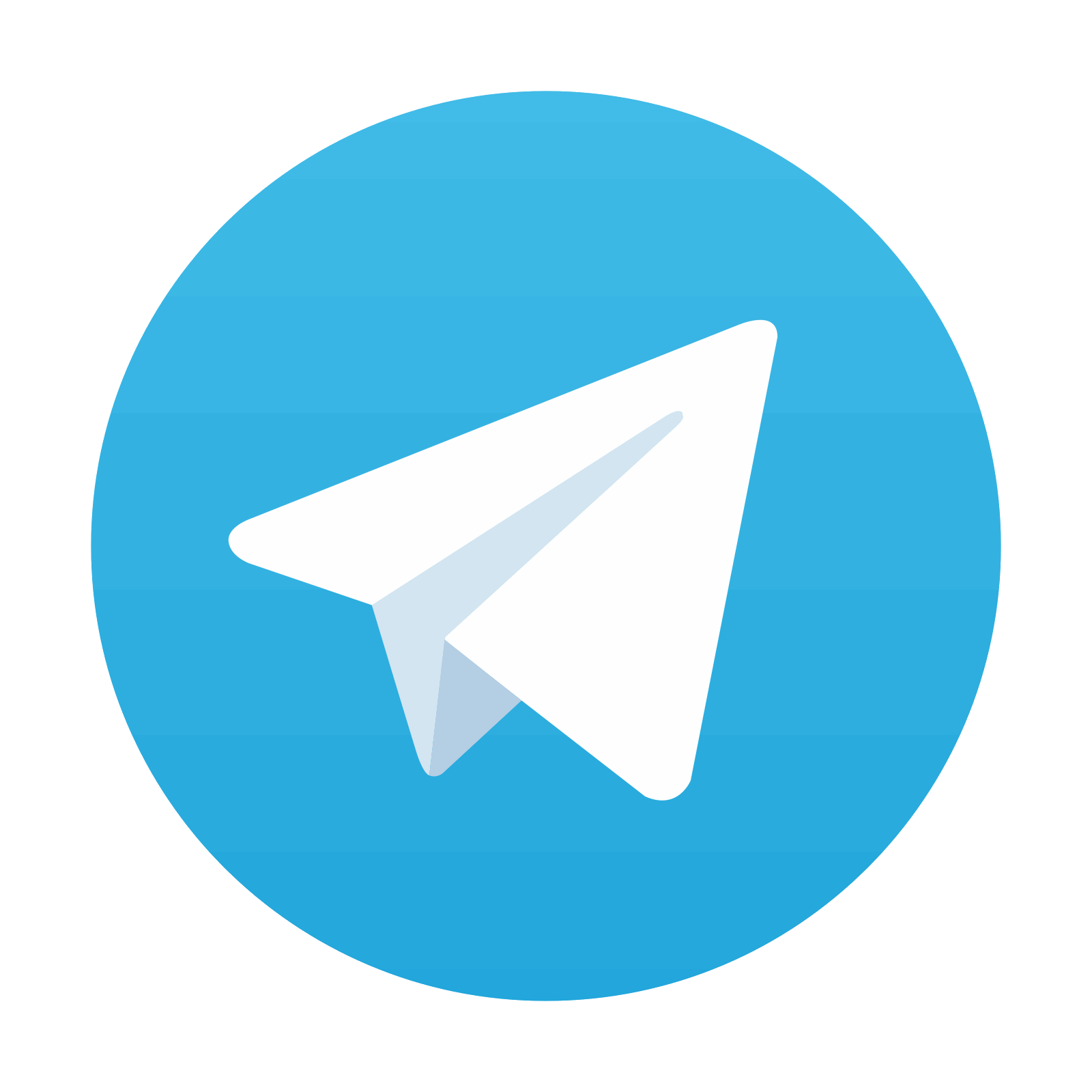
Stay updated, free dental videos. Join our Telegram channel

VIDEdental - Online dental courses
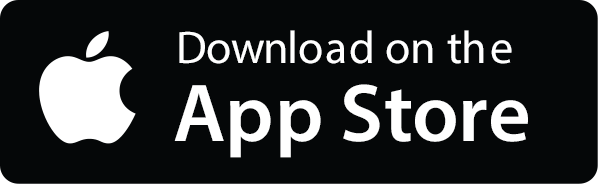
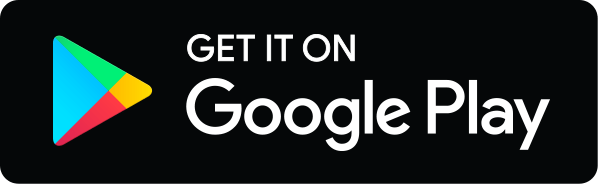
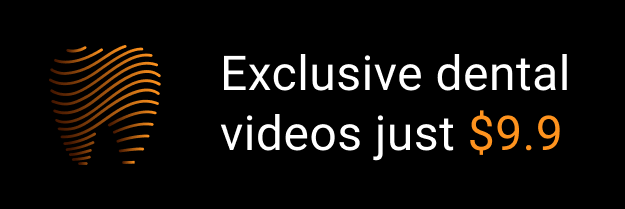