© Springer International Publishing Switzerland 2016
Barbara Zavan and Eriberto Bressan (eds.)Dental Stem Cells: Regenerative PotentialStem Cell Biology and Regenerative Medicine10.1007/978-3-319-33299-4_3
Isolation and Cryopreservation of Stem Cells from Dental Tissues
(1)
Wolfson School of Mechanical and Manufacturing Engineering, Loughborough University, Loughborough, Leicestershire, LE11 3TU, UK
(2)
School of Dentistry, College of Medical and Dental Sciences, University of Birmingham, St Chad’s Queensway, Birmingham, B4 6NN, UK
Keywords
Dental pulpCryopreservationGingivaCell banksBiological tissues
Introduction
One of the principal aims of cell-based therapies is to deliver personalised medicine for the repair and regeneration of tissues lost to accidents or disease. To achieve this aim sub-zero temperatures (−196°) are applied that halt biological activity, thus preserving the cells for future clinical applications. The idea of banking stem cells as a means of ‘biological insurance’ has seen a recent rise in popularity that is at least in part due to increased media attention and a greater public awareness of regenerative medicine. Consequently, several companies now exist offering individuals the opportunity to store their own multipotent cells, with the aim of future therapeutic application to restore or regenerate a multitude of tissues throughout the body.
In order for cellular therapies to be effective and widely available a number of biological and pragmatic considerations need to be taken into account. Firstly, the cryopreservation procedure employed must assure the quality and safety of the final biological product. This is of particular importance when the banked cells are intended for clinical use. In order to ensure that cryopreserved stem cells are suitable for clinical application standardised protocols and procedures are required that adhere to current good manufacturing practice (cGMP) [1, 2]. This means that cryo-profiles including type, concentration, equilibration, and dilution of cryoprotective agent (CPA) as well as freezing rate and thawing conditions must be optimised and validated for particular cells/tissues [3]. Additional factors to be considered when banking stem cells for clinical applications are: potential cytotoxicity of the CPA and the immunogenicity of animal or human serum components often incorporated within the cryopreservation medium (CPM). Implementation of these standards will help ensure that cell viability, proliferation, genetic and epigenetic stability, and differentiation are not compromised, allowing banked stem cells to be as clinically effective as freshly isolated cells. One final point concerns the more pragmatic aspects of space, cost-effectiveness, and the establishment of GMP standards governing freezing protocols and the use of CPAs [4]. The scaling of stem cell therapies to meet clinical demand is by no means a simple task and the future success of cellular therapies is equally likely to depend on advances in the field of healthcare engineering as well as in basic science and medicine [5]. This concise review will focus on issues surrounding the cryopreservation of dental tissues, in particular stem cells isolated from dental pulp, which are an emerging and promising source for a wide range of regenerative therapies.
Principles of Cryopreservation
Cryostorage causes a physical insult to cells which could result in structural and molecular changes. The principal aim of any cryopreservation procedure is to provide adequate protection from damage caused by the formation of intracellular and extracellular ice crystals during both freezing and thawing stages [6]. In order to achieve this, CPAs are incorporated in the freezing medium. An ideal CPM is one in which cells maintain their stem cell characteristics over long periods of time (durability), with no loss in biological viability or vitality. CPAs work by penetrating the cell membrane where they bind to water molecules in solution. This acts to prevent an efflux of water from the cytoplasm during freezing, avoiding cellular dehydration and maintaining stable intracellular salt concentrations and pH [7]. To date, a number of different CPMs have been tested for the storage of mesenchymal stem cells (MSCs) derived from a variety of tissues, with the most common solution incorporating dimethyl sulfoxide (DMSO) as a CPA [8]. In addition to the choice and concentration of CPA used, the rate of freezing is also important for maintaining stem cell viability. Cells may incur damage caused by the process of rapid cooling (cold shock) or by the low temperatures used to enable long term preservation (chilling injury). The optimal cooling rate is likely to be specific for each tissue, and is largely determined by the ratio of cell volume to surface area, and by the permeability of the membrane to water and the CPA. The optimal cooling rate is also likely to depend on the concentration of CPA within the cryopreservation medium. Ideally, a CPA solution should be non-toxic for cells and patients, non-antigenic, chemically inert, ensure high cell viability after thawing and allow quick and simple transplantation without the need for additional washing steps [9]. However, producing a freezing environment that is optimal for both cells and patient will require further research, and it is likely that a compromise will have to be reached.
Dental Stem Cell Banking
This chapter focuses on the long-term storage of dental tissues, in particular stem cells derived from the vital central gelatinous tissue called the dental pulp. Dental pulp stem cells (DPSCs) were first identified at the turn of the twenty-first century and have since been shown to have the capacity to differentiate down multiple lineages [10]. DPSCs originate from the neural crest and are responsible for the formation of dentine producing cells called odontoblasts [11].
Barlett and Reade were the first scientists to cryopreserve dental tissue [12]. However, at that time dental cryopreservation was intended for the storage of whole teeth required for auto-transplantation rather than for stem cell isolation [13]. Recently the focus has shifted away from auto-transplantation towards the use of adult dental stem cells as a means of restoring or replacing lost or decayed dental tissue [10]. Indeed, adult stem cells are becoming increasingly popular as a cell source for regenerative medicine since they are not subject to the same ethical concerns as embryonic stem cells. Teeth in particular contain a significant proportion of adult mesenchymal stem cells (MSCs) in relation to their tissue mass [14]. Dental stem cells from extracted teeth have further appeal due to the fact that the process of isolating these multipotent cells is relatively simple and avoids invasive procedures associated with other popular sources of MSCs , such as bone marrow or fat. One of the most promising sources of dental stem cells resides within the primary dentition, which becomes lost during maturation. When human deciduous teeth are lost and replaced by permanent teeth, stem cells located within the pulp chamber can be harvested and stored as a convenient and pain-free source for regenerative medicine. The stem cells from human exfoliated deciduous teeth, or SHED cells, are a rapidly proliferating and multipotent source that are often favoured over other bankable dental stem cells located within the pulp of wisdom teeth or orthodontically extracted teeth [15]. The process of isolating and storing cells from teeth is relatively cost-effective when compared with MSCs derived from cord blood—a popular source for banking [16]. Additionally, the efficacy of these cryopreserved dental MSCs has been validated by a number of studies showing that post-thaw viability and differentiation following short and long term storage remains unaffected [17, 18]. These data highlight the promise of dental MSCs as a source of regenerative cells for long-term banking and clinical application. However, for the creation of cell banks the question of whether to bank intact whole teeth or the isolated stem cell population needs to be further considered.
Banking Intact Whole Teeth
Frequently stem cells are isolated, purified and expanded (to approximately 80–90 % confluence) prior to cryopreservation and the majority of this chapter will primarily focus on this method. However, one should be aware that this method requires resources for stem cell culture and scaling to be available upfront, thereby increasing workload and subsequent cost, with the further caveat of introducing possible phenotypic changes in the cells during expansion. It has been argued that a more rational and cost effective approach would instead be to bank whole teeth, allowing for the isolation and expansion of stem cells only when required. At present research into whole tooth banking for stem cell isolation is limited, and several groups attempting to cryopreserve intact teeth have shown a 25 % incidence of longitudinal fractures after freezing, which may compromise cell viability [19]. Methods for the banking of whole teeth have also encountered difficulties in enabling the CPA to reach the central pulpal region where the MSCs are localised. This consequently leads to problems with pulp necrosis, and can significantly reduce the viability, and hence the availability and clinical application of the cells [20, 21]. Importantly, current evidence demonstrates that MSC immunophenotype and differentiation is unaffected following whole tooth banking [22]. However, there are mixed reports concerning the effects of whole tooth banking on cell proliferation rates and morphologies [19, 22–24]. In order to improve the efficacy of whole tooth banking modern methods such as laser-piercing have been used to introduce microchannels, allowing CPA to reach the central pulp [21]. However, the procedure of whole tooth banking for MSC extraction is still in its infancy, and further advancements will be required if such a method is to be applied on a global scale.
International Licensed Dental Stem Cell Banks
To deliver effective autologous regenerative therapies dental stem cells need to be stored in a state of stasis. To achieve this purpose tooth banks are being developed that provide members of the public the opportunity to bank these clinically useful cells for an annual fee. Currently, the number of international licensed dental stem cell and tooth banks is low. However, due to increased media and public awareness of regenerative medicine and the potentially revolutionary impact of these technologies on the healthcare sector the number is likely to increase. Currently, a number of international laboratories offer collection and storage of dental stem cells, and the ease of the process typically makes it simpler and cheaper than banking cord blood stem cells. Historically, the world’s first tooth bank was developed in Japan at Hiroshima University in 2005 [25]. The country’s national tooth bank was opened soon after in 2008—a collaboration between Hiroshima University and Taipei Medical University. A similar development has since taken place in Norway as collaboration between the Norwegian Institute of Public Health and the University of Bergen [26]. However, tooth banking is not restricted to academic institutions, with a number of commercially licensed tooth banks existing in developed countries such as the UK and USA, as well as in developing countries such as India. At present the USA dominates the commercial tooth banking market with several companies such as BioEden, Pharmacells, Store-A-Tooth and StemSave expanding throughout North America, Europe and the rest of the world.
Cryopreservation Procedure
If banked stem cells are intended for therapeutic use it is important that current standards of good manufacturing practice are implemented throughout the entire process, from cell collection to freezing, storage and post-thaw assessment. The development of a standardised cryopreservation protocol for dental stem cells will require specification of the following:
Tooth Collection
One of the most significant problems related to the cryopreservation of dental tissue is the time elapsed between tissue harvesting and banking [27]. As soon as the tooth is lost or removed the vital pulp will begin to degrade, causing irreparable damage to the cellular component. A previous study has shown that in order to maintain DPSC viability, cell isolation and cryopreservation must occur within 120 h of tooth extraction [23]. Currently, to minimise tissue degradation, teeth are placed in sterile hypotonic phosphate buffered saline solution, which prevents dehydration. This can be done by the individual, the individual’s parents/guardians (in the case of SHED cells) or a dental health professional. The tooth is then transferred into a vial, which is carefully sealed and placed into a thermette (a temperature phase change carrier) and transported to the banking facility in an insulated metal transport vessel—these materials are often provided by the tooth bank [16]. The procedure is designed to maintain the sample in a hypothermic state, and the process is referred to as sustentation. It is of critical importance that at the time of extraction, the central pulp region appears red in colour, indicating sufficient blood flow at the time of isolation. Teeth should be excluded if the central pulp appears grey, as this indicates pulp necrosis. The most appropriate source of deciduous teeth for isolation and banking are the canines and incisors, since these teeth often contain a healthy source of pulp that is starting to loosen. Primary incisors and canines with no pathology and at least one third of the root in-tact can also be banked [28]. Primary molars are not recommended for SHED banking as they take a longer time to resorb, which can have a negative impact on the pulp chamber and consequently on the viability of the stem cells [29].
Cell Isolation and Expansion
The majority of tooth banks isolate and expand the cells prior to freezing. However, before the dental pulp can be isolated the tooth must undergo a rigorous disinfection procedure. Sterilization can be achieved by washing the cells with PBS and povidone iodine [16]. Washes with commercially available bactericidal solutions (e.g. chlorhexidine) may also be used to reduce the number of microorganisms and help further decrease the risk of cross-contamination [23]. After the tooth has been washed and disinfected DPSCs are isolated using protocols originally developed by Gronthos et al. in the following way [30]:
- 1.
Pulp tissue is removed from the tooth manually using sterile forceps or a dental excavator. The pulp may also be removed by flushing the pulp chamber with sterile salt water or saline solution.
- 2.
The excavated pulp is placed in a sterile petri dish and washed several times with PBS to further reduce the risk of bacterial contamination.
- 3.
Pulp tissue is digested with a Collagenase type-I (3 mg/mL)/Dispase II (4 mg/mL) solution or 0.5 % Trypsin/EDTA solution for a period of 1 h at 37 °C.
- 4.
Digested pulp tissue is passed through a 70 μm cell strainer to obtain single cell suspensions.
- 5.
Cell suspensions are cultured at 37 °C/5 % CO2 in growth medium; Alpha-modified Eagle’s medium (α-MEM) supplemented with 20 % foetal bovine serum (FBS), 2 mM L-glutamine, 100 μM L-ascorbate-2-phosphate, 50 U/mL penicillin, and 50 μg/mL streptomycin.
- 6.
In order to characterise or improve the homogeneity of MSC cultures fluorescence activated- (FACS) or magnetic activated- cell sorting (MACS) can be applied to select for Stro-1+ or CD146+ cells. Previous studies have demonstrated that DPSCs can be accurately identified through the presence of these cell surface antigens [34]. However, it should be noted that purification of MSC cell populations may not always be beneficial as it can adversely affect their differentiation and therapeutic potential [32].
Introduction of a Cryopreservation Solution
Incorporation of a CPA within the freezing medium prevents the formation of intracellular ice crystals that damage the cytoplasmic membrane during freezing. Protocols utilising DMSO as a CPA are widely used both in research and the clinic, since this method consistently allows high post-thaw recovery and differentiation [35]. However, there are a number of documented issues concerning the use of DMSO. Firstly, DMSO is recognised as a cytotoxic chemical that can cause adverse side effects such as sickness if introduced to a patient during cell therapy [36]. Secondly, DMSO has been reported to cause epigenetic changes and cytotoxicity at levels routinely used for MSC cryopreservation [37, 38]. Surprisingly, given the known adverse effects of DMSO there are currently no regulations or guidelines governing the use of this chemical for cell banking. In fact, a review of the current literature has identified that 10 % DMSO is most commonly incorporated within the freezing medium—currently used by approximately 80 % of cell therapy centres [39]. However, due to the documented negative effects of this CPA on both cells and patient there is a need to find effective alternative methods. Reducing the concentration of DMSO has been postulated as one way of reducing associated cellular toxicity [8]. However, reports have shown that the survival and number of colonies formed by MSCs was significantly decreased as a consequence, and that the magnitude of this decrease was inversely proportional to DMSO concentration [23]. Furthermore, concentrations below 7 % have been shown to cause a significant decline in DPSC viability [2, 23]. Therefore, it was proposed that an alternative approach that employs a simple post-thaw washing step may represent an effective way of reducing the patient-associated effects of this CPA without affecting cell recovery [40]. However, this approach fails to take into account any epigenetic changes associated with the incorporation of 10 % DMSO. Furthermore, if applying such an approach, one has to be careful not to trigger a damaging osmotic shock [23]. To avoid triggering an osmotic shock a similar result might achieved by avoiding post-thaw washes and instead simply fractionating the delivery of stem cells [41].
Alternatively, one could reduce the concentration of DMSO in the CPM by incorporating biocompatible CPAs that reduce the risk of cellular toxicity and accompanying side effects upon cell transplantation. It is important that these non-toxic alternatives are able to provide a level of efficacy similar to DMSO without the documented side-effects. Lowering the concentration of DMSO by incorporating sugar derivatives such as hydroxyethyl starch or trehalose has been shown to provide comparable levels of protection from freezing-induced cell damage to traditional 10 % DMSO-based solutions [9, 42]. The incorporation of CPAs such as methylcellulose or polyethylene glycol has also shown promise, reducing the concentration of DMSO required in the CPM. Importantly, these freezing mediums provide a level of protection that virtually eliminates the need to incorporate serum, thereby reducing potential immunological complications resulting from cell transplantation. Indeed, the formulation of an effective serum-free CPM is likely to represent an important step required for the clinical translation of cellular therapies [43–46].
Complete replacement of DMSO with non-toxic alternatives has proven a more difficult task. For instance CPAs such as glycerol and proline are effective when used in combination with DMSO but provide inadequate protection when used independently [47]. In fact only a small number of CPAs have been identified that provide adequate cryoprotection without the need to incorporate DMSO. Currently, only CPMs containing polyvinylpyrollidone (PVP) or ectoin have been shown to achieve levels of MSC post-thaw viability and differentiation comparable to DMSO-based solutions [45, 47]. However, at present these studies are not comprehensive enough to draw any significant conclusions, and the efficacy of these CPAs will need to be evaluated in long-term studies before their clinical applicability can be comprehensively assessed. In conclusion, significant progress is being made in the identification of serum-free freezing mediums that contain non-toxic CPAs for the cryoprotection of MSCs. However, at present no studies have analysed the effects of novel biocompatible CPAs on multipotent dental stem cells. Therefore, considerable further investment is required into research that comprehensively examines the efficacy of non-toxic CPAs for dental stem cell banking.
Freezing Protocol
Controlled-Rate Freezing
Damage caused by cell dehydration and ice crystal formation can be minimised by controlling the freezing rate. A slow and controlled freezing rate of 1–2 °C per minute is generally considered optimal for maintaining MSC viability during cryopreservation [48, 49]. Controlled rate freezers (CRFs) are frequently used to accurately control the freezing rate, and can be either manual or programmable [50, 51]. Although controlled-rate freezing has the advantage of reducing the likelihood of cellular damage occurring through ice crystal formation, one of the fundamental issues with CRFs is that they cannot be scaled to provide uniformity of temperature to all vials during large scale banking [52]. This represents a significant problem, and if cell therapies are to be applied on a national or international scale advances in CRF technology will be required. Furthermore, CRF development, along with vapour phase storage in liquid nitrogen is costly, and could impact the clinical translation of regenerative medicine. Ultra-slow programmable freezing systems (USPF) represent an alternative method that apply a highly controlled freezing rate of −0.3 to −0.6 °C per minute, allowing comparatively high recovery rates and increased levels of efficiency that are in compliance with cGMP requirements [4, 53]. However, a significant caveat is that USPFs are more complex and costly than traditional slow rate freezing (SRF) [54]. Much like CRFs, scaling is also a significant pragmatic consideration when applying USPFs for the high-volume banking of dental tissue. Ultimately, the choice between programmable or manual freezing will be dependent on the resources available and the experience of the scientists or technicians on site.
Uncontrolled-Rate Freezing
An alternative approach where samples are first cooled at −4 °C and then directly deposited into a freezer at −80 °C or liquid phase nitrogen can also be applied [55]. Uncontrolled-rate freezing represents a comparatively cheap and simple way of cryopreserving cells that requires no specialist training. This method has been most frequently applied for the preservation of peripheral blood stem cells with consistent results [56, 57]. Initial studies have also shown that uncontrolled freezing can be successfully used for the long-term cryopreservation of dental pulp stem cells [58]. Based on the parameters of the study the authors found that this method was as effective at maintaining cell viability, proliferation, and differentiation as CRFs with subsequent liquid nitrogen storage. However, to date uncontrolled-rate freezing has only been tested in the presence of 10 % DMSO, and studies will be required to test the efficacy of this method for dental stem cell preservation in combination with low DMSO concentrations or non-toxic DMSO replacements.
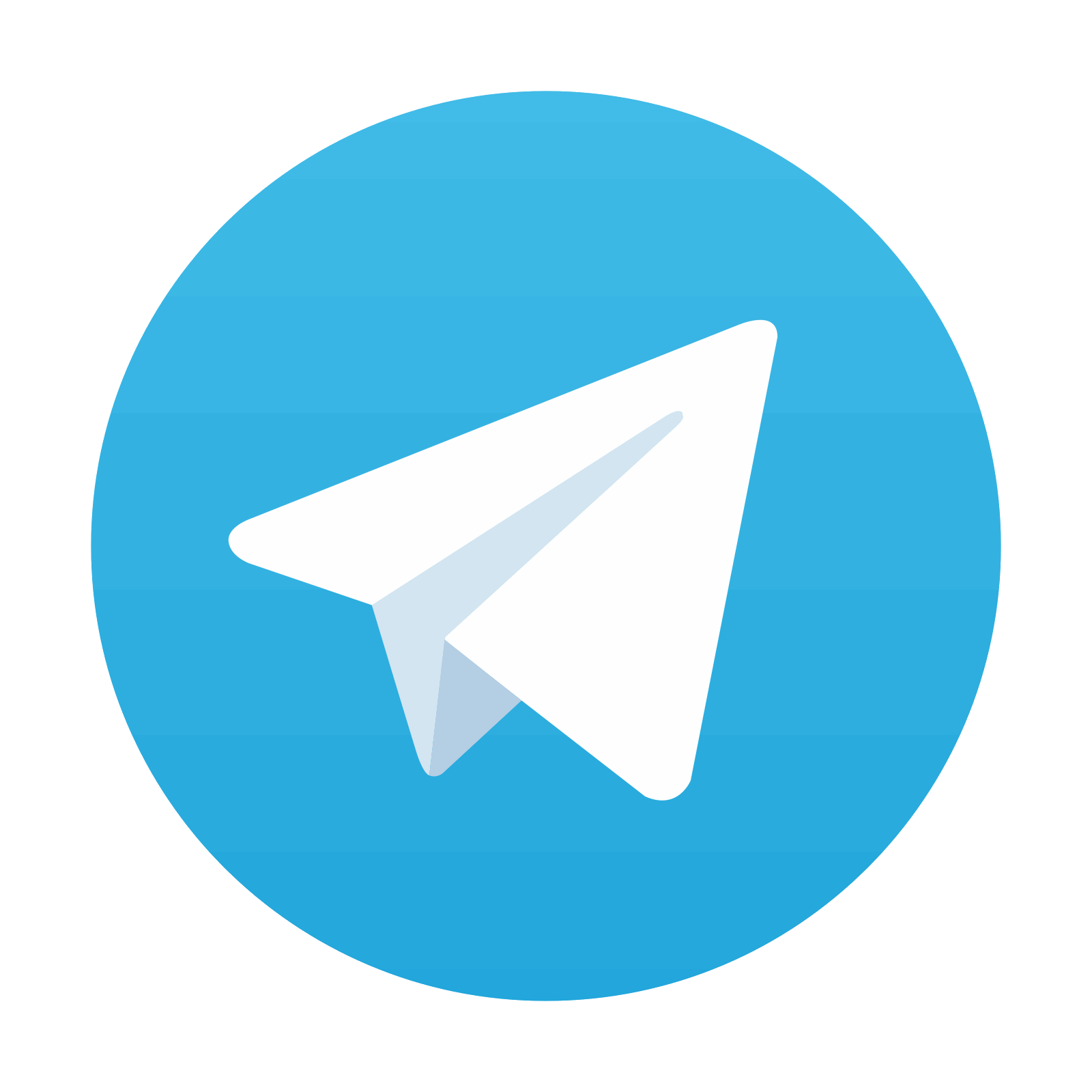
Stay updated, free dental videos. Join our Telegram channel

VIDEdental - Online dental courses
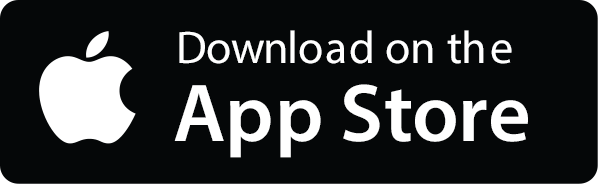
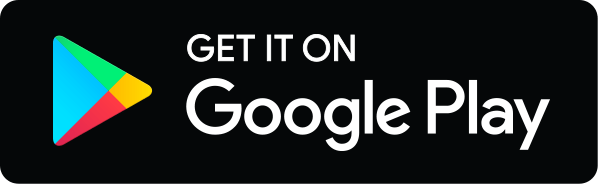
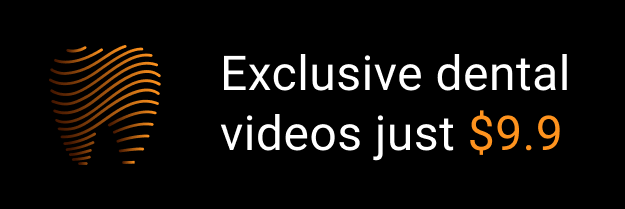