7
Root‐End Filling and Perforation Repair Materials and Techniques
Josette Camilleri1 and Christof Pertl2,3
1School of Dentistry, Institute of Clinical Sciences, College of Medical and Dental Sciences, University of Birmingham, Birmingham, UK
2Department of Dental Medicine and Oral Health, Medical University of Graz, Austria
3Harvard School of Dental Medicine, Boston, MA, USA
7.1 Introduction
Corrective or apical surgery is undertaken when management of primary disease fails or problems are encountered during root canal therapy. The prognosis of apical surgery has changed with the adoption of microsurgical approaches (i.e. using a microscope, ultrasonic tips, and new materials). However, a review of the effects of surgical and nonsurgical therapy in the retreatment of teeth with apical periodontitis and of surgical root‐end resection employing various conditions, materials, devices, and techniques found no clear evidence of superiority for either with regard to healing at 1‐, 4‐ or 10‐year follow‐up, although the results were of very low quality [1]. Only two random controlled clinical trials have found that healing rates can be higher in cases treated surgically as compared to nonsurgically, at least in the short term. A single trial reported that, in the medium to long term, healing rates for the two procedures were very similar. There is presently little evidence for a sound decision‐making process amongst alternatives for the retreatment of cases presenting with periradicular pathosis. More well‐designed random controlled trials should be performed, with a follow‐up of at least four years and a consistent sample size, in order to detect the true difference in the long term between the outcomes of the two approaches, if any exists [2].
Apical surgery is necessary when there is persistent active periodontitis at the root end of the tooth. Corrective or reparative surgery is required to seal off perforations connecting the root canal system to the periodontal ligament space along the tooth root and surrounding bone. Perforation repair can be performed through the root canal or surgically. The challenges with nonsurgical repair of perforations include the inability to properly assess the size of the defect, difficulty in gaining access, difficulty in controlling the amount of material extruded to the periodontium and subsequently in removing this excess, and difficulty in controlling bleeding, which might affect the material used to seal the perforation.
For both corrective and apical surgery, a material is required to block the pathway from the canal to the periapical area or the exit created along the root during perforation. Several materials have been used for this purpose. The major advances in this field have been the introduction of the operative microscope and microsurgery, the use of piezo‐sonics for root‐end canal preparation, and the development of the hydraulic silicate cements.
7.2 The Surgical Environment
Access to the apical area, or the root face which needs repair, is gained by raising a flap and possibly removing overlying bone. Flap design is dependent on the anatomy of the area, the number and location of roots, and whether the flap is in an aesthetically sensitive region. Usually, sulcular incisions with either mesial or distal vertical releasing incisions (Figure 7.1a), or both, are performed. The vertical part of the incision should extend from the alveolar mucosa to the attached gingiva and end in a line perpendicular to the gingival margin (Figure 7.1b). Vertical releasing incisions allow easier mobilization of the flap for better visibility (Figure 7.1c). The releasing incisions of a palatal flap must be rather short (4–6 mm) to avoid trauma to the palatal artery (Figure 7.1d) A submarginal flap design (Figure 7.2) avoids touching the critical gingival margin but creates a risk of unpleasant postoperative scarring along the incision line.
Once incisions are made and the flap is raised, the root end or area of perforation often needs to be accessed by removal of the overlying bone (Figure 7.2a), although in some cases there is none as it has been resorbed during the disease process (Figure 7.3a). For perforation repair, the intervention is similar.
With apical surgery, once the flap is raised, the root apex is exposed, and root‐end resection follows (Figure 7.2b); the clinical implications and management of this have been thoroughly discussed [3]. Root‐end resection is necessary to remove any pathology, such as root resorption, fractured root tips, cysts, granulomata, tissues, and infected cementum and dentine at the root apex. It further allows removal of anatomical variations such as root curvatures, lateral and accessory canals, apical deltas, and calcifications. Other indications include the avoidance of operator errors in canal preparation, likely improvement in the removal of a soft tissue lesion, and the need for access to the canal system. The root end should not be significantly bevelled buccally; a rather perpendicular resection angle reduces the number of exposed dentinal tubules and facilitates an anatomically correct class I retrograde ultrasonic preparation. Still, the angles of resection used will be affected by the root inclination and curvature, number of roots, thickness of bone, and position of the root in the bone and arch. Root‐end resection is best performed with a fissure bur under water spray.

Figure 7.1 Flap outline showing (a) sulcular triangular flap with a mesial vertical incision; (b) guiding of the releasing incision from the alveolar mucosa, proceeding to the attached gingiva; (c) flap retraction to expose the surgical site of a full‐thickness flap with a distal vertical incision; (d) sulcular palatal flap with two short releasing incisions.
Source: Christof Pertl.

Figure 7.2 (a) Raised submarginal flap and buccal bone perforation around the root apices of affected teeth, caused by pathological inflammatory processes. (b) After apical resection, the black discolouration of the root canal indicates bacterial contamination. (c) Retrograde preparation of the root canal. (d) Root‐end filling. (e) Suture placement.
Source: Christof Pertl.

Figure 7.3 Details of an apical surgical procedure following incision and raising of a flap. (a) Surgical site, showing the cystic lesion having eroded the buccal plate. (b) Root‐end cavity preparation with ultrasonic tip. (c) Placement of root‐end filling material.
Source: Christof Pertl.

Figure 7.4 Root‐end resection, preparation, and filling. (a) Resected root end of a lower first molar mesial root, showing two canals filled with gutta‐percha, joined by an isthmus which has been left unobturated. (b) Use of methylene blue to differentiate the isthmus region and the dentine from the surrounding bone. (c) Root‐end cavity preparation. (d) Filled root end.
Source: Christof Pertl.
The appearance of the root face following root‐end resection will vary depending upon the type of bur used, external root anatomy, anatomy of the canal system exposed at the particular angle of resection chosen, and nature and density of the root canal filling material. Once the root tip is removed, it is necessary to check for isthmuses (Figure 7.4a), as these will be a continuous source of infection. Methylene blue is used to delimit the dentine from the surrounding bone and to expose any untreated canal anatomy present (Figure 7.4b).
Microscopically, dentinal tubules will now be exposed to the periradicular tissues. Cementum can reform over such resected tubules and a periodontal encapsulation, with a variable degree of fibre attachment, can occur [4]. Another problem with exposed dentinal tubules is the risk of reinfection of the periradicular tissues by bacteria entrapped inside them. However, no correlation has been found between the presence of microrganisms in the dentinal tubules and the degree of periradicular inflammation [4]. The prepared dentine surface also has a potentially infected smear layer, which may serve as a source of irritation to the periradicular tissues, primarily preventing the intimate layering of cementum against the resected tubules. The smear layer may block these tubules and serve as a source of obturation of the potential avenues of communication, especially with tubules contaminated with bacteria or exposed to oral fluids over long periods of time. Experiments designed to evaluate these aspects are essential, as there are no unequivocal data to guide the proper management of such a smear layer.
A root‐end cavity is prepared in order to enable an adequate seal against potential avenues of communication from the resected root end to the canal system (Figures 7.2c and 7.4c). This preparation should be performed with ultrasonic tips (Figure 7.3b), and its design made to suit the material to be used to make the seal. The use of ultrasonic tips allows apical preparations along the long axis of the root after minimal root‐end resection. Dentine demineralization, and thus exposure of collagen by smear‐layer removal, is debatable. Ideally, the area should not be irritated. The prepared end is filled (Figures 7.2d, 7.3c, and 7.4d), the site irrigated, and the flap repositioned and sutured (Figures 7.2e). A similar procedure is used for perforation repair (Figure 7.5).
The cavity design for adhesive materials is essentially a simple concave shape, as the materials can be bonded. Other material types, such as hydraulic calcium silicate cements (HCSCs) and zinc oxide‐eugenol (ZOE), require a deeper retentive cavity. The use of high magnification in the preparation of shallow and concave root‐end cavities for bonded resin‐based root‐end filling materials, as compared with endodontic microsurgery with the use of high‐magnification, ultrasonic root‐end preparation, and root‐end filling with Super EBA, intermediate restorative material (IRM), and HCSC, has been reviewed [5]. The root‐end cavities prepared for ZOE and HCSC had a higher probability of success compared with shallow cavity preparation and placement of a resin‐based material.

Figure 7.5 Clinical case showing the procedure for root perforation repair. (a) Preoperative periapical view, showing a mesial mid‐root perforation by a metal post, part of a crown used as a bridge abutment on tooth 25, and a pier abutment for a four‐unit bridge replacing tooth 26. (b) Postoperative radiograph. (c) Recall radiograph taken two years later, showing complete healing and bone formation in the defect. (d) Clinical photograph of the site. (e) Flap design showing a sulcular flap with a mesial relieving incision. (f) Flap raised and surgical site exposed. (g) Exposed root surface showing perforation. (h) After repair. (i) Flap repositioned and sutured.
Source: Christof Pertl.
In terms of surgical protocol, there is some evidence that using a papilla base incision may be beneficial for the preservation of the interdental papilla compared with complete papilla mobilization, with no evidence of less pain in the papilla base‐incision group at day 1 post‐surgery [1]. However, suturing of the flap to a small papilla fragment may lead to poor healing in practice. Using more modern techniques, such as by modifying the method of osteotomy (i.e. the type of instrument used) or the type of preparation of the retrograde cavity (apicoectomy angle, instruments used for root‐end preparation), resulted in better healing [6]. Although there is very little evidence that ultrasonic devices for root‐end preparation improve healing at one year after retreatment as compared with the use of a traditional bur, in practice such burs are not much used nowadays, as the dedicated ultrasonic tips allow better access. One study showed evidence of better healing at one year when root ends were filled with an HCSC rather than being treated by smoothing the end of an orthograde gutta‐percha root filling [1].
The use of magnification helps the clinical procedure, and is today accepted as best practice. The type of optics used seems to be important: an operating microscope provided 94.9% successful healing, whereas loupes gave only 90.6%. Endoscopic devices have been advocated in order to improve visibility [7]. No difference in outcome is shown with the treatment of different arches [8].
The correlation between clinical outcome and material type is not very clear. However, a longitudinal assessment of the prognosis of apical microsurgery at five years was 8% poorer compared to assessment at one year, and material choice was shown to affect this rate [7]. ProRoot MTA had a better outcome than either Super EBA [7] or amalgam [6] at five years.
7.3 Materials for Endodontic Surgery
The environment in which endodontic surgery is performed – specifically, where root‐end filling and perforation repair materials are placed – is shown in Figures 7.1–7.5. For convenience, nonsurgical perforation repair is included here too, as the intent, context, and materials are all the same. However, when the materials are used in the coronal aspect, which is the only location where nonsurgical repair is possible, they may be subject to a different environment. This will be noted in the appropriate sections.
A root‐end filling material must be adapted to preexisting gutta‐percha and sealer as well as to the exposed, cut dentine surface, which potentially has exposed dentinal tubules and a smear layer (Figures 7.3 and 7.4). The area is inherently wet and will get blood over it within a few minutes. These conditions are very particular and very adverse. The materials used for root perforation offer other challenges, too (e.g. for the irrigation protocol and the material used to restore the tooth after repair).
A number of materials have been used for root‐end filling, usually adopted from restorative dentistry and not specifically designed for this purpose. Such materials have included dental silver amalgam and ZOE cements, as well as filled (composite) resins (FRs) and glass ionomer cement (GICs). The properties of these materials will be discussed in Section 7.3.1. In the mid‐1990s, HCSCs were specifically developed for use in endodontic surgery. Their properties will be discussed separately in Section 7.3.2.
To date, there are no standards for testing endodontic surgery materials. The only ISO standard for endodontics‐related procedures is that for root canal sealer [9]. Otherwise, the conventional materials used in this context are tested according to their own respective standards [10–14]. As yet, there is no standard for HCSCs.
7.3.1 Conventional Materials
A wide range of types of materials have been used clinically for root‐end filling. These include gold foil, polycarboxylate cement, gutta‐percha (both cold and injectable), silver amalgam, GIC, and various ZOE‐based cements. In this chapter, only the most common and most researched materials will be discussed. Although historically dental silver amalgam was used as a root‐end filling material, it will not be included here due to the European directive to limit its use [15]. This directive was based on environmental pollution concerns rather than for any toxicity issues.
The chemistry, mixing, and physical and biological properties of each material type will be discussed, with special attention given to clinical applications and particular clinical interactions. There is no evidence of any leakage technique which shows the superiority of any one material compared to another, never mind of the clinical relevance of leakage [16]. Any reference to sealing ability in this chapter is related to the interaction of the material with dentine.
7.3.1.1 Zinc Oxide‐Eugenol Cements
ZOE‐based materials have a variety of uses in dentistry. Commercial formulations also contain zinc acetate dihydrate as an accelerator, which furnishes the water required to initiate the setting reaction. Reinforced versions have been used for root‐end cavity restoration. These include Super EBA (Bosworth Co., Skokie, IL, USA) and IRM (Dentsply Caulk, Milford, DE, USA). Although these are often grouped together, they exhibit slightly different characteristics and properties.
7.3.1.1.1 Chemistry and Physical Properties
Super EBA is a cement whose liquid is a mixture of 32% eugenol and 68% ortho‐ethoxybenzoic acid (EBA). This kind of material was first suggested for root‐end filling in 1978 [17]. The chelating agent is EBA, which leads to a material with high water solubility, although this is reduced by the addition of hydrogenated rosin [18]. The mechanical properties can be improved by the addition of substances such as monocalcium phosphate, fused quartz, or aluminium oxide. In fact, the commercial product also has an ‘alumina‐reinforced’ version.
Table 7.1 Properties of ZOE‐based root‐end filling materials.
Property | Super EBA | IRM | Reference |
---|---|---|---|
Setting time (min) | 9 | 6 | [21] |
Compressive strength after setting (MPa) | 60 | 52.2 | [21] |
Compressive strength after three weeks (MPa) | 78.1 | 57.4 | [21] |
Tensile strength (MPa) | – | 4.1 | [22] |
IRM includes polymer particles in the powder, an idea first suggested by Curtis [19]. For root‐end fillings, a high powder‐to‐liquid ratio (P : L) is recommended. High P : L has other advantages, such as ease of placement and decreased setting time, toxicity, and solubility [20].
ZOE materials set through the formation of the salt zinc eugenolate, a weak five‐membered ring chelate, as a matrix embedding unreacted zinc oxide, whilst Super EBA forms zinc ortho‐ethoxybenzoate, a stronger six‐membered ring chelate.
The properties of Super EBA and IRM are listed in Table 7.1 [21, 22]. Super EBA was stable with no weight changes over a period of storage in water, whilst IRM showed a reduction in weight over three weeks [23]. Reinforced ZOE cements have a radiopacity of between 5 and 8 mm Al/mm, which is similar to that of a filled gutta‐percha, at about 6.1 mm Al/mm [24]. It is thus difficult to distinguish such a root‐end filling material from the obturating material.
Although leakage studies are not being taken into consideration here, it is worth mentioning the marginal adaptation of these materials. IRM exhibits the largest gaps and poorest adaptation compared with both Super EBA and amalgam [23]. Using a high‐speed bur to finish and contour hardened Super EBA preserves the marginal adaptation [25].
7.3.1.1.2 Biological Properties
The biological activity of root‐end filling materials is important, particularly their interaction with the periodontium and bone. This interaction has been studied at the cellular level, assessing toxicity in contact with human periodontal ligament cells and osteoblast‐like cells. In one study, both IRM and Super EBA cement were found to be less toxic than amalgam [26]. In another, later study, however, both freshly mixed and set amalgam were found to be significantly less toxic than Super EBA or IRM [27]. Compared with the HCSCs developed later, IRM has been shown to be more cytotoxic [28]. In other work, modified ZOE cements induced cell death in both periodontal ligament cells [29, 30] and gingival fibroblasts, even after their surfaces had been washed to remove eluate [30]. Both material composition and surface texture affect cellular adhesion and morphology. The contradictory data obtained could be due to variations in the test method and the cell culture system used. It has been reported that the choice of end point and the cell culture system affect the results of cytotoxicity experiments on dental materials [31]. Neither IRM nor Super EBA is mutagenic, as determined by the Ames Test [32].
Reactions to implanted IRM and Super EBA include the formation of granulation tissue [33] and the production of a fibrous mass. When set materials were used as implants, the EBA‐containing cement was always less irritating than ZOE ones [34]. An initial inflammatory reaction was observed, which subsided over time. Furthermore, when there was no inflammation, healing progressed by formation of connective tissue [35]. There was no evidence of inhibition of dentoalveolar or osseous wound healing associated with these materials [36]. When used as a root‐end filling material in dogs, IRM showed a better interaction than Super EBA. Both materials were associated with the formation of new root cementum on the resected dentine surface [37].
It has recently been shown that application of enamel matrix derivatives on denuded root dentine promotes periodontal regeneration. Enamel matrix derivatives were shown to adhere to the etched dentin, but their adherence to IRM was significantly less than that to dentine or FR [38].
7.3.1.1.3 Antimicrobial Properties
The antimicrobial properties of these materials have not been extensively studied for this specific application. There is no literature on these properties in perforation repair. Using Enterococcus faecalis bacterial cultures, IRM was found to be adequately antimicrobial initially, but to be significantly less so after seven days [39]. It also showed a three‐hour delay before any antimicrobial activity manifested. The set material completely inhibited Pseudomonas aeruginosa and delayed or limited the growth of Staphylococcus aureus and E. faecalis [40]. In contact with S. aureus, E. faecalis, and Streptococcus mutans, the minimum bactericidal concentration of IRM was higher than that of HCSC. Both materials were reported to show adequate antimicrobial properties [41].
7.3.1.1.4 Clinical Technique
For root‐end surgery, after raising the flap and exposing the surgical site, the materials must be prepared and placed in situ as described in Section 7.1. For perforation repair, depending on the level of the perforation, either a flap can be raised or the repair can be effected through the access cavity. In either case, the dentine will be prepared and a smear layer, potentially packed with bacteria, will be formed. There are no reports of the effect of smear‐layer removal on the performance of reinforced ZOE cements.
Super EBA and IRM are both presented in a powder and liquid form (Figure 7.6). Super EBA cement provides good handling characteristics when properly mixed. However, mixing is difficult, requiring more effort and practice than most other root‐end filling materials. The cement is well suited for conservative ultrasonic root‐end preparations and may be placed through the use of the convex surface of a small spoon excavator and packed with appropriately‐sized instruments [42]. IRM behaves best when handled correctly. A thick mixture improves the ease of root‐end placement. Because it does not adhere well to itself, it should be inserted as a single mass and packed in place rather than being placed incrementally [42].

Figure 7.6 Presentation of (a) IRM
(Source: Courtesy of Dentsply Sirona) and (b) Super EBA
(Source: Keystone Industries) as both a powder and a liquid.
Both materials are mixed on a glass slab by adding increments of powder to the liquid until the mixture is not sticky and can be rolled into a ball. The postoperative healing of root‐end surgery performed using IRM has been reported to be similar to that of HCSCs at around three to five hours, and still to be so even 48 hours after the procedure [43].
7.3.1.1.5 Environmental Interactions
For all endodontic surgery materials, the environmental interactions include that with dentine and that with blood and tissue fluids. For perforation repair materials, the interactions with the irrigating solutions and subsequently the restorative materials must additionally be considered.
There has not been much research on the interaction of ZOE cements with dentine. Any ‘bond’ is purely a result of mechanical key, although its failure has been said to be ‘mixed adhesive and cohesive’ [44]. However, the bond ‘strength’ when the material is used as a root‐end filling material is lower than that of HCSCs [44]. Super EBA was shown to have a very good sealing ability, although this deteriorated with time [45]. The use of irrigating solutions affected the bond strength and sealing ability of these materials when they were used for perforation repair. Either sodium hypochlorite irrigation or no irrigation was better than irrigation with chelating agents such as ethylene diamine tetra‐acetic acid (EDTA), which led to reduced sealing ability [46]. Hypochlorite irrigation did not affect the bond strength of IRM [47, 48], whilst Super EBA lost strength when exposed to sodium hypochlorite and other oxidizing agents used in tooth bleaching [47].
For both procedures, washout behaviour is important, both during the irrigation of the site prior to flap repositioning and in the long term via natural interaction with blood in the area. IRM exhibited low washout, similar to amalgam and lower than for HCSCs [49, 50]. The importance to the long‐term success of the procedure arises because if washout is fast, the area may be recolonized by bacteria, leading to treatment failure.
7.3.1.1.6 Clinical Evaluation
Although a number of studies evaluating material properties have included reinforced ZOE cements as perforation repair materials, there is no clinical evidence of their suitability for this specific purpose. No clinical study has ever reported success in such an application, notwithstanding long clinical use.
Both IRM and Super EBA had a successful outcome when used as root‐end filling materials. Radiologic and clinical examination indicated a success rate of 91% for IRM and of 82% for Super‐EBA after 12 months [51]. Similarly, an 85% success rate has been found for IRM, although this is lower than the 90% recorded for gutta‐percha [52]. IRM used in cavities prepared ultrasonically exhibited an 80% success rate overall; this was highest for incisors (100%) and lowest for molars and premolars (78 and 69%, respectively) [53]. When IRM was used as a retrograde filling material, the clinical success rate was similar to that of an HCSC [54, 55]. Super EBA gave a similar outcome [56], even at four years [57].
7.3.1.2 Glass Ionomer Cements
Both conventional and resin‐based GICs will be discussed in this section, although the latter are less popular for use in perforation repair and root‐end filling. The main material characteristic of interest is dentine bonding through chemical interaction (chelation), but attention has also been given to ion leaching, particularly of fluoride.
7.3.1.2.1 Chemistry and Physical Properties
GICs are composed of an acid‐reactive calcium fluoroaluminosilicate glass powder mixed with a polyacrylic acid solution. The material properties are dependent on the glass composition. Various compositions have been used, and aluminium‐free formulations containing strontium [58–60], zinc [59, 61, 62], or both have been developed for use as bone cements. The partial substitution of calcium oxide by strontium, magnesium, or zinc enhances the cement’s mechanical properties [63], improves its antimicrobial characteristics [64], and reduces the available aluminium.
The chemical composition and properties of GICs are well documented in a series of articles by Crisp and Wilson published between 1974 and 1977 [65–73]. The polyacrylic acid solution is modified by the addition of tartaric and maleic acids, which improve the material’s handling and setting.
GICs undergo various stages of setting and maturation, which may be described as dissolution, gelation, and hardening. The acidic liquid attacks and dissolves the glass surface, leaching predominantly calcium early in the reaction, followed by the slower release of aluminium (and further calcium); these ions form the salt matrix. At the same time, a hydrated silica gel is formed. Fluoride and sodium are also released in the long term. Initial set (gelation) is due to the cross‐linking of the polymeric acid by calcium ions. Hardening occurs when the calcium is subsequently displaced by aluminium ions. The gelation stage is the most susceptible to contamination and drying out. Hardening can take up to seven days.
In part, the tartaric acid binds the calcium ions released early in the reaction and so controls the working and setting times. The rate of formation of aluminium cross‐links is also increased, with a resultant reduction in setting time.
GICs have been modified by sintering with silver or by the addition of a resin (i.e. a light‐curing polymer component), for so‐called ‘cermets’ and resin‐modified GICs (rmGICs), respectively. Cermets are not very popular in endodontic practice. The ability to command‐cure by light makes rmGICs useful for various clinical applications, although they release less fluoride than the non‐resin‐modified cements. The resin improves working and setting times, strength, toughness, and resistance to acid attack and desiccation. These materials are dual‐cure: light activation leads to polymerization of the monomer, whilst the conventional GIC acid–base reaction proceeds at a slightly slower pace than in unmodified cements.
7.3.1.2.2 Biological Properties
The biocompatibility of GICs used as root‐end filling materials and for the repair of root perforations has been assessed both at the cellular level and following implantation in test animals. The reported cellular interaction is not clear, with studies showing dense and confluent cells [74], poor cellular attachment [75], or periodontal ligament cell apoptosis [76, 77], indicating that the material does not preserve the integrity of the periodontal tissues [78]. The leachate has been found to be worse than that from Super EBA, amalgam, and mineral trioxide aggregate (MTA) in inhibiting cell proliferation [31]. The resin‐based cements were cytotoxic to primary human gingival fibroblast cultures, inhibiting cell growth and proliferation [79]. Their eluates were also toxic. In contrast, Geristore, an rmGIC, exhibited enhanced biological behaviour for human periodontal ligament cells and superior biocompatibility in comparison with MTA and conventional GIC [80]. On this basis, it can be suggested as a material of choice for root resorption, perforations, and root‐end filling. Both material and time affected cell viability with rmGICs, showing less cell‐inhibitory effect initially when compared with dental silver amalgam; at 48 and 72 hours, however, all materials exhibited a similar, slightly inhibitory effect [81].
Intraosseous implantation of GIC resulted in connective tissue infiltration with plasma cells and a reaction similar to that induced by ZOE‐based materials [82, 83]. The tissue response to rmGIC was shown to be similar to that with ZOE: a mild inflammation initially, which subsided with time – much better than the response to amalgam [84].
7.3.1.2.3 Antimicrobial Properties
The antibacterial effect of GICs is related to their acidity [85], since adjustment of the liquid to pH 5 resulted in a decrease in activity [86]. Furthermore, fluoride concentration has been linked with antimicrobial activity, with no such activity reported when the fluoride release was lowered [87]. The effect of fluoride will not have a great impact in GICs used in endodontic surgery, however, since fluoride recharge is necessary for continuous fluoride release. The antibacterial activity of GICs increases with topical applications of fluoride toothpaste and gels [87].
7.3.1.2.4 Clinical Technique
GICs are marketed according to their particular use in dentistry. For endodontic surgery, the ordinary restorative materials are employed. For these purposes, several brands are available, and further material developments have been undertaken to improve their properties. The restorative GICs require a specific clinical technique which involves the removal of the smear layer from the dentine by treatment with polyacrylic acid solution. There is no literature on the effectiveness of this cavity ‘conditioning’ on the bonding of GICs to root dentine in the present context.
Conventional GICs are powder–liquid systems. The polyacrylic acid may be present in solution, as is the case with Fuji IX (GC Europe, Leuven, Belgium; Figure 7.7a), or incorporated in the powder (when the cement is to be mixed with water), as in Chemfil (Dentsply, DeTrey, Konstanz, Germany; Figure 7.7b). The mixing ratio is critical to the outcome. The scoop provided to dose the powder must be used, and the liquid must be properly measured by a dropper. The conventional GICs are also available in pre‐dosed capsules, to be mixed by mechanical shaking, which therefore require a nozzle and a special applicator in order to facilitate clinical application (Figure 7.7c), even though the P : L ratio is adjusted lower to enable this approach. The rmGICs have various presentations: both powder–liquid systems and syringeable pastes which can be placed directly at the site (Figure 7.7d). The mixing of powder–liquid systems is done on a paper pad supplied for the purpose, in proportions specified by the manufacturer. The root‐end cavity may be a simple saucer shape prepared by ultrasonic instrumentation. GIC can also be placed as a retrograde filling material without a root‐end cavity preparation. A resin‐modified version in particular exhibited good adaptation to the root canal walls [88]. The placement of GIC at the surgical site is carried out by the use of a ‘flat plastic’ instrument, and it is packed with a plugger or burnisher.
7.3.1.2.5 Environmental Interactions
The ordinary restorative clinical use of GICs includes dentine ‘conditioning’ to enable adhesion, and a varnish over the material to minimize water sorption and early ion leaching, both of which result in poorer cross‐linking and deterioration of strength. This behaviour has not been investigated in the context of surgery and perforation repair. GICs show very good interaction with coronal but not with radicular dentine. The quality of the seal is debatable. It has been shown to be comparable to that of amalgam in one study [89] but not in others [90, 91]. Debatable findings are also reported for the rmGICs compared with the plain acid–base version. Whilst larger gaps were found on a scanning electron microscope (SEM) for resin‐modified cements at the root end [92], dye leakage with methylene blue was less than with conventional GICs [93]. In more recent studies, GIC root‐end filling materials exhibited large marginal gaps compared with HCSCs [94, 95], but again this result is debatable as good marginal adaptation was observed for GICs when measured both directly and on resin replicas [96] and when using capillary‐flow porometry [97].
The bond strength of GICs to root dentine has only been reported for perforation repair materials; blood contamination results in a drop in value [98]. Neither the effect of irrigating solutions nor washout appears to have been investigated for GICs in the present context.
7.3.1.2.6 Clinical Evaluation
Although GICs have been used for endodontic applications for a number of years, clinical studies assessing their performance are scarce. A review of the literature showed that GIC appeared to be equivalent to amalgam [99], with amalgam being the worst root‐end filling material studied.

Figure 7.7 Various presentations of glass ionomer cement. (a) Fuji IX.
Source: Courtesy of GC Europe . (b) Chemfil.
Source: Courtesy of Dentsply Sirona. Both (a) and (b) are examples of conventional hand‐spatulated cements. (c) Fuji IX capsules (a dentine conditioner is also supplied).
Source: Courtesy of GC Europe. (d) Geristore, a resin‐modified glass ionomer.
Source: Denmat.
7.3.1.3 Filled Resin and Dentine Bonding Systems
The use of a dentine bonding system (DBS), with or without the use of FR, is another example of a material (and technique) borrowed from restorative dentistry and adapted to endodontic surgery. Creation of a leak‐resistant apical seal is possible with this approach, although such use is technique‐sensitive. A dry field is necessary for the dentine bonding agent and FR root‐end filling, although some such fills may be less sensitive to moisture. The popularity of the approach lies in the ability to bond to dentine and thus (presumably) seal off the freshly exposed dentinal tubules following root resection. FRs and DBSs as used in restorative dentistry for coronal restoration can be employed, although a number of products have been developed especially for root‐end filling; one such is Retroplast (Retroplast Trading, Roervig, Denmark). For the repair of root perforations, the use of a restorative material does not pose much difficulty except in the need to maintain a dry field during application. In nonsurgical repair of root perforations, the tooth can be restored by bulk filling with FR.
7.3.1.3.1 Chemistry and Mechanical Properties
FRs used for restorative procedures are classified according to the filler particle size. They consist of an organic polymeric matrix with an inorganic filler bonded to it by a silane coupling agent. The initially fluid but viscous monomer is converted into a rigid polymer by free radical‐addition polymerization, now commonly light‐activated. Conceptually, the base monomer is the very high‐viscosity bisphenol A‐glycidyl methacrylate (bis‐GMA). Its high molecular weight helps minimize polymerization shrinkage. However, low‐viscosity monomers must be incorporated to make the filled system workable (e.g. methyl methacrylate (MMA), ethylene glycol dimethacrylate (EDMA), or triethylene glycol dimethacrylate (TEGDMA)). An activator–initiator system is present to allow command set, but polymerization inhibitors such as hydroquinone must be included to provide a useful storage life. The filler is present for many reasons: increased strength and stiffness, reduced polymerization shrinkage, water sorption and thermal expansion, control of optical appearance, and provision of radiopacity.
Retroplast was developed specifically for root‐end filling and uses ytterbium trifluoride as its principal filler. The original formulation included silver to increase its radiopacity, but this led to discolouration [100].
7.3.1.3.2 Biological Properties
The release of unpolymerized monomer in contact with periapical tissues is of particular concern. Various possible resin components have been found to suppress macrophage mitochondrial activity, with TC50 values at 24 hours reported as follows: HEMA, 10 000 μmol/l; 4‐META, 3800 μmol/l; Bis‐GMA, 130 μmol/l; UDMA, 110 μmol/l [101]. The effect is time‐dependent, and residual effects were observed for all resins [101]. Macrophage cytokine secretion has also been investigated [102]. All dentine bonding components completely suppressed lipopolysaccharide (LPS)‐induced interleukin‐1β (IL‐1β) and tumour necrosis factor alpha (TNF‐α) secretion at concentrations that suppressed mitochondrial activity by 50%. In addition, 4‐methacryloxyethyl trimellitic anhydride induced secretion of IL‐1β, but not TNF‐α, without the LPS challenge. These results indicate that DBS components may alter normal macrophage‐directed inflammatory responses if the macrophages are exposed to sufficiently high concentrations [102]. Polymerized DBSs can also alter the viability of monocytes, decreasing it with time [103].
The biological activity of root‐end filling materials has been assessed at the relevant local cellular level using gingival fibroblasts, periodontal ligament, and bone cells. Retroplast in contact with fibroblasts and macrophages resulted in cell death and no cytokine production, particularly early in incubation [104]. Conversely, leachates from Retroplast were less cytotoxic than those from Super EBA cement [105]. Reactions at the tissue level were assessed by connective tissue interactions in test animals. FRs caused moderate to severe inflammatory reactions in the first seven days, but these decreased by 60 and 90 days, performing as well as amalgam [106]. Although most materials were considered to be biocompatible when first tested, compared with more recent developments they actually performed poorly. In fact, cellular attachment to Retroplast was shown to be very poor compared with MTA [75].
7.3.1.3.3 Antimicrobial Properties
The antimicrobial properties of FRs and DBSs used for root‐end filling have been assessed using the direct‐contact test, which is independent of the solubility of the materials and so gives more predictive and quantitative data. These materials are not as antimicrobial as MTA or IRM [40].
7.3.1.3.4 Clinical Technique
Use of a DBS and FR permits conservative root‐end preparation. A slightly concave preparation (rather than a conventional deep cavity) followed by resin bonding to the entire resected root end is suggested. This has the advantage of sealing exposed dentinal tubules as well as the main canal(s). All polymerizing resins leave an uncured oxygen‐inhibited surface layer that may interfere with initial healing and should therefore be removed with a cotton swab before wound closure [107], although this is likely to be an incomplete process.
7.3.1.3.5 Environmental Interactions
The effect of blood contamination has been investigated for DBSs by evaluating their leakage. Although leakage has been shown to be a very limited and essentially useless test, it is used very frequently. A number of systems tested did not leak, and blood contamination did not adversely affect their performance [108]. The use of a DBS enhances the retention of composites and reduces leakage [109].
7.3.1.3.6 Clinical Evaluation
An excellent long‐term clinical success rate (90%) with the use of Retroplast FR and Gluma (Bayer AG) DBS has been reported [100]. A clinical study comparing modified Retroplast with the original formulation containing silver at one year showed 80% complete healing, 2% scar tissue, 12% uncertain healing, and 6% failure. No significant difference in this healing pattern was found between the two formulations. When the outcome was uncertain at one year, a roughly two‐ to four‐year follow‐up showed improved outcomes [100]. Retroplast also gave a very good outcome in another clinical study [110], where based on radiographic evaluation the healing of treated roots was characterized as complete (77%), incomplete (5%), uncertain (7%), or unsatisfactory (11%). Some 95% of roots classified as completely healed at the one‐year control were also completely healed at the final examination at four years. Long‐term study therefore suggests that Retroplast can be used for root‐end filling with a successful treatment outcome [110]. However, Retroplast also gave less predictable healing compared with MTA, with lower success rates, and did not perform as well in all tooth types, especially mandibular premolars and molars [111]. Five‐year follow‐up showed that MTA had a higher success rate in the long term [112].
7.3.1.4 Other Materials and Techniques
Besides the conventional materials used in restorative dentistry, other materials and agents have also been employed with a view to enhancing healing potential and thus clinical success. Enamel matrix derivatives adhere to dentine, but also very well to FRs used as root‐end filling materials [38]. Bone cement has been tested as an alternative to restorative materials, but with no apparent benefit – although the testing was limited to that for leakage [113].
7.3.2 Hydraulic Cements
HCSCs were first introduced in 1993, specifically for root‐end surgery and repair of root perforations. The first two papers published on hydraulic cements described the sealing ability of so‐called ‘mineral trioxide aggregate’ (MTA), used to repair lateral root perforations [114] and as a root‐end filling material [115]. MTA is essentially composed of Portland cement and bismuth oxide, and this formulation was patented – the grey version in 1993 and 1995 [116, 117], the white in 2002 [118, 119]; all patents are now expired.
The invention was based on the hydraulic nature of Portland cement, used in the construction industry as a binder for concrete. Bismuth oxide (Bi2O3) was added as a radiopacifier [24]. The first product was Grey MTA, marketed by Dentsply Tulsa in 1998, followed shortly by White MTA. These were the only such products available until MTA Angelus was launched in 2001 by Brazilian company Angelus. Being a trade name, ‘MTA’ should only be used as such for relevant branded products: mixtures of Portland cement‐like materials and bismuth oxide. The term ‘MTA‐like’ is sometimes used to describe other formulations, but it is improper to use ‘MTA’ generically. The term that describes this type of material precisely is ‘hydraulic silicate cement’ [120]; in this book, the abbreviation ‘HCSC’ is used, as it stresses the calcium content. Such materials are distinct from all other types of dental material in that they interact chemically with the environment they are placed in as a result of their hydraulic nature; that is, the setting reaction can in part (at least) involve water from the site. A classification of hydraulic cements was proposed in Chapter 1, and the different subtypes based on the material composition are summarized in Table 7.2. In this chapter, only the subtypes used in reparative and corrective procedures will be discussed. A complete classification of the hydraulic cements has been published in the literature [121].
Table 7.2 Classification of hydraulic cements based on their composition.
Type | Cement | Radiopacifier | Additives | Water |
---|---|---|---|---|
1 | Portland cement | ✓/ × | × | ✓ |
2 | Portland cement | ✓ | ✓ | ✓ |
3 | Portland cement | ✓ | ✓ | × |
4 | Tricalcium/dicalcium silicate | ✓ | ✓ | ✓ |
5 | Tricalcium/dicalcium silicate | ✓ | ✓ | × |
7.3.2.1 Portland Cement‐Based Hydraulic Cements: Types 1–3
7.3.2.1.1 g539Chemistry and Mechanical Properties
The Type 1–3 materials include Portland cement as the main cementitious and active system. The most well characterized of these is the first product to have been sold: ProRoot MTA (Dentsply). Type 2 materials include additives to enhance their performance. The Type 3 materials are provided in ready to use syringes and are not mixed with water. They hydrate by interaction with the environmental fluids. One type of commercial material is Bio C‐Repair by Angelus.
MTA Chemistry
The essential compounds of the original products called ‘MTA’ are di‐ and tricalcium silicates, plus small amounts of tricalcium aluminate, which make up the Portland cement portion, along with a little calcium sulphate added to control setting and 20% bismuth oxide for radiopacity [122, 123]. Grey MTA also contains tetracalcium aluminoferrite [21]. The initial reports were somewhat confused, indicating the presence of oxides and phosphates [21, 114, 115], which is apparently what prompted the label ‘mineral trioxide aggregate’.
An HCSC such as MTA is mixed and reacts with water to form hydrated calcium silicate and calcium hydroxide. Tricalcium aluminate reacts with sulphate (from the added calcium sulphate) and rapidly forms a so‐called ‘high’ sulphate or sulphoaluminate known as ettringite, which decomposes to a ‘low’ sulphate or sulphoaluminate (monosulphate) when the sulphate ions are depleted in solution by the first reaction [124, 125]. This controls the rate of the setting process. The overall progress of the reaction with water is quite complex, having four stages [126–129]. In the pre‐induction stage (first few minutes), rapid ion dissolution occurs and the tricalcium silicate reacts to form calcium hydroxide and calcium silicate hydrate gel, deposited at the cement particle surface. Very little dicalcium silicate reacts in the initial stages. The tricalcium aluminate also hydrolyses rapidly, forming ettringite, again on the cement particle surface. A halo of reaction products can be seen to form around the cement particles (Figure 7.8). Pre‐induction is followed by the induction or dormant stage (1–2 hours), in which the hydration reaction progresses very slowly because the first products form a diffusive barrier. In this stage, the cement is plastic and workable. Setting proper commences when the barrier coating ruptures and solid reaction products gradually replace the liquid phase. The acceleration stage follows (some 3~12 hours after mixing), when the rate of hydration increases again, controlled by the nucleation and growth of the reaction products. At this stage, the dicalcium silicate reaction commences, forming more calcium silicate hydrate gel and calcium hydroxide. Finally, in the post‐acceleration stage, the hydration rate slows gradually as the amount of unreacted material declines and diffusion becomes more difficult.
The hydration reactions are outlined as follows:


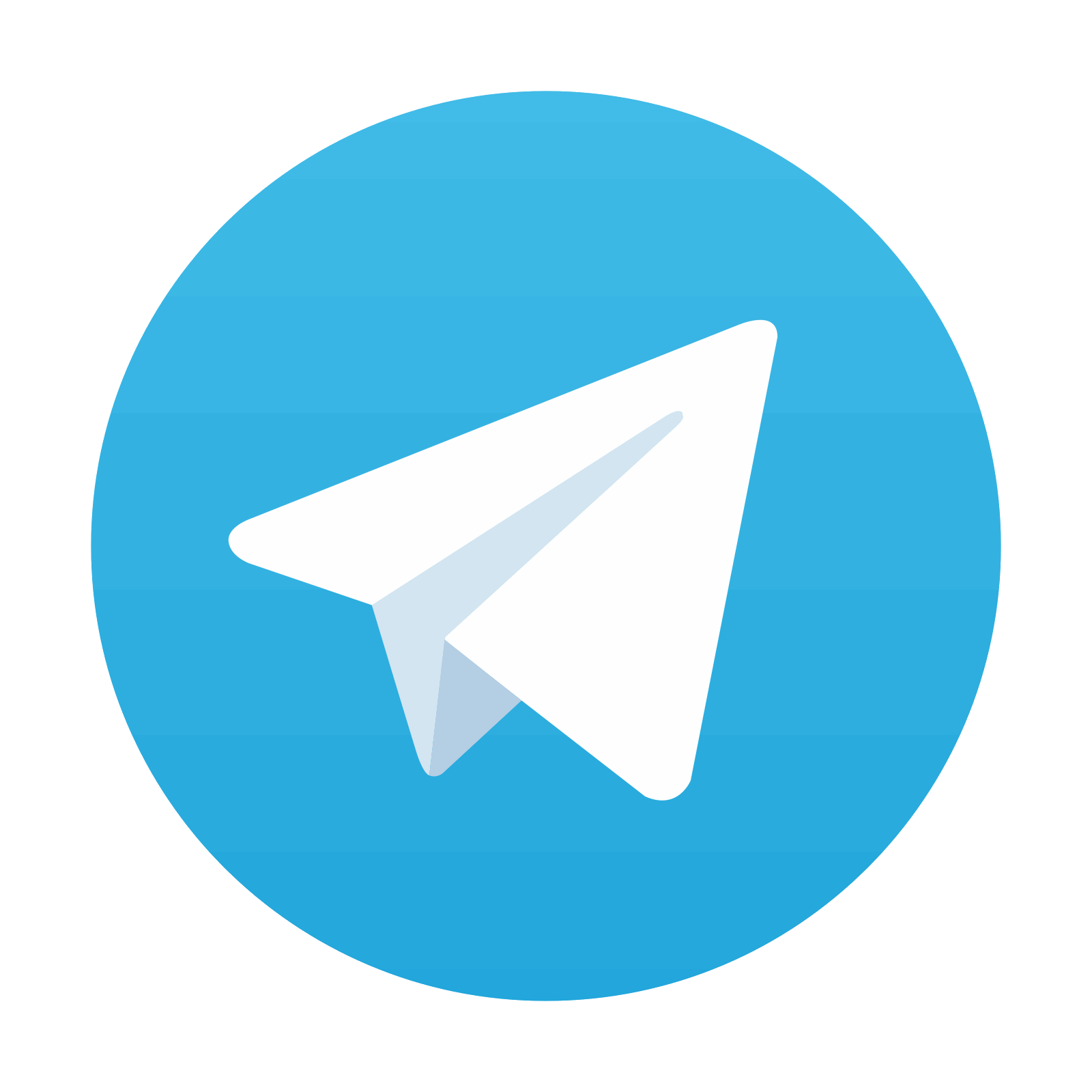
Stay updated, free dental videos. Join our Telegram channel

VIDEdental - Online dental courses
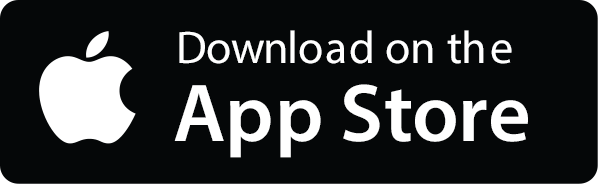
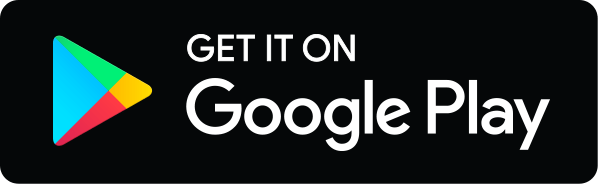