5
Irrigating Solutions, Devices, and Techniques
Christos Boutsioukis1 and Maria Teresa Arias‐Moliz2
1 Department of Endodontology, Academic Centre for Dentistry Amsterdam (ACTA), University of Amsterdam and Vrije Universiteit Amsterdam, Amsterdam, The Netherlands
2 Department of Microbiology, Faculty of Dentistry, University of Granada, Granada, Spain
5.1 Introduction
Irrigation plays a pivotal role in root canal treatment [1, 2]. A considerable part of the root canal system is beyond the reach of instruments [3], so their main role is to provide radicular access in order to facilitate irrigant delivery [1]. Irrigating solutions are therefore expected to kill microorganisms and disrupt the biofilm in most of the root canal system (Figure 5.1), inactivate virulence factors such as endotoxin, dissolve pulp‐tissue remnants, remove hard‐tissue debris and the smear layer created during instrumentation or prevent their formation, provide lubrication for instruments, and be biocompatible and easily available at low cost [1, 2, 4]. Amongst all these requirements, the strong antimicrobial effect is arguably the most important. However, even the best irrigating solution will not fulfil these goals if it cannot reach bacteria and tissue remnants inside the root canal system in sufficient amounts. Therefore, effective irrigant delivery and activation/agitation techniques are equally important, in order to ensure that the solutions are delivered throughout the root canal system and adequately refreshed so that a high concentration of their active components is maintained. Irrigation techniques must also create a flow that carries bacteria, biofilm fragments, and tissue remnants out of the root canal; this can contribute to the mechanical cleaning. Finally, the action of irrigating solutions should be limited within the constraints of the root canal system [5].

Figure 5.1 Scanning electron microscope photograph of a 24‐hour bacterial biofilm grown on dentine. A characteristic network of polysaccharides covering the bacteria is starting to be formed.
Source: Maria Teresa Arias‐Moliz.
In order to reach all these goals, a wide variety of solutions and techniques have been proposed for root canal irrigation, and new ones are constantly being introduced. Not all solutions and techniques are equally effective or popular, however, so this chapter will focus only on the most commonly used or known ones. Furthermore, where possible, these will be compared to the current clinical standards, with which most readers are expected to have some experience; that is, sodium hypochlorite (NaOCl), ethylenediamine tetraacetic acid (EDTA), syringe irrigation, and ultrasonic activation.
5.2 Irrigating Solutions
5.2.1 Sodium Hypochlorite
NaOCl is the most popular irrigating solution in use today, and is considered the primary irrigant of choice during chemomechanical preparation [6]. Its active chemical compound is the free available chlorine, consisting of the hypochlorite ion (OCl−) and hypochlorous acid (HOCl) [7]. The hypochlorite ion prevails in alkaline solutions (pH > 7.6) and has a powerful oxidative effect which is responsible for a tissue‐dissolving activity, whereas the hypochlorous acid predominates in more acidic solutions (pH < 7.6) and has a stronger bactericidal effect, possibly because it is a smaller uncharged molecule that easily penetrates the bacterial membrane and disrupts proteins [8]. Lowering the pH renders the solution more unstable without a clear benefit in terms of antimicrobial activity, whilst maintaining a stable high pH can increase the proteolytic action of the solution and improve the chemical debridement of the root canal [9, 10].
NaOCl possesses several of the properties of an ideal root canal irrigant [2]. Due to the free available chlorine, it exerts a strong antimicrobial effect against both planktonic and biofilm bacteria (Figure 5.2) [11–16], and it can also dissolve pulp‐tissue remnants [17–19]. In addition, it reduces bacterial virulence factors related to periapical inflammation and clinical symptoms, such as endotoxins and lipoteichoic acids [20]. The main disadvantages of NaOCl are that it cannot dissolve hard‐tissue debris and the inorganic components of the smear layer, and it is caustic to soft tissues, leading to rapid haemolysis, ulceration, inhibition of neutrophil migration, and destruction of endothelial and fibroblast cells [21]. Thus, inadvertent extrusion towards the periapical tissues through the apical foramen or a perforation may result in a NaOCl accident [22, 23]. Accidental ingestion of small amounts of NaOCl during root canal treatment due to insufficient isolation is unlikely to have any clinically significant effect, but it should nevertheless be prevented [24]. Prolonged skin contact may cause irritation or dermal hypersensitivity [24], whilst contact with the eye may cause a chemical burn [25, 26]. Moreover, NaOCl is a very reactive solution; in addition to bacteria and pulp‐tissue remnants [27–29], it reacts strongly with the proteins in the dentine matrix, possibly altering the mechanical properties of the latter [30], and also with most other irrigants, so the available chlorine is rapidly consumed in the root canal [31–33]. Therefore, it has a strong but short‐term effect and is not suitable for use as an inter‐appointment medicament.

Figure 5.2 Dentinal tubules contaminated with Enterococcus faecalis after treatment with 2.5% NaOCl for three minutes. Red‐coloured bacteria are cells with damaged membranes, whilst green‐coloured bacteria are cells with intact membranes viewed under a confocal laser scanning microscope following LIVE/DEAD staining (BacLight; Invitrogen, Eugene, OR, USA). The pulpal side is at the upper left side of the specimen.
Source: Maria Teresa Arias‐Moliz.
Concentrations ranging from 0.5 to 6% have been proposed for use during root canal treatment. The desirable effects of NaOCl are concentration‐dependent, becoming stronger with increasing concentration [11, 34] – but so too are its undesirable effects [30, 35, 36]. In order to compensate for the rapid consumption of the free available chlorine in the root canal, frequent exchange with large volumes of fresh irrigant is advocated [27‐29, 37]. To date, however, there are no specific guidelines concerning the optimum concentration, volume, and contact time, arguably because of the highly variable clinical situation with regard to the microbial load, anatomy, amount of pulp‐tissue remnants, and so on.
In order to improve the effectiveness of NaOCl without increasing its concentration, preheating of the solution to 50–60 °C prior to irrigation has been suggested [38]. The temperature increase seems to amplify its antimicrobial activity [18, 39], as well as its ability to dissolve pulp tissue [18, 34, 40] and the organic component of the smear layer [41]. However, the clinical relevance of this additional step is questionable, since the temperature of the solution drops to 37 °C very soon after intracanal delivery in vivo [42]. Heating of NaOCl in situ (inside the root canal) has been proposed as an alternative to extraoral preheating [43, 44], but the efficacy and the potential side effects of this method have not yet been fully evaluated.
Finally, NaOCl is inexpensive and widely available. The amount of free available chlorine in solutions obtained from domestic sources is unpredictable, so professional sources should be preferred [45]. Even though it has demonstrated an acceptable shelf‐life [46], stored NaOCl solutions become unstable when in contact with organic matter, light, and other chemicals, which accelerates the loss of free available chlorine [31, 47]. Storage in opaque nonreactive bottles with air‐tight caps placed in a cool, dark place is therefore crucial [48].
5.2.2 Chlorhexidine
Chlorhexidine gluconate (CHX) is a cationic bisbiguanide with an antimicrobial activity against bacteria, yeast, and fungi, particularly Candida albicans [15, 49, 50]. The CHX molecule has the ability to bind to the negatively charged microbial cell walls, thereby altering their osmotic equilibrium [51]. At low concentrations, it exerts a bacteriostatic effect, leaking low‐molecular‐weight substances out of the microorganisms, whereas at higher concentrations it has a bactericidal one, due to precipitation or coagulation of the bacterial cytoplasm components [52, 53].
CHX has been proposed for root canal irrigation at a concentration of 2% [2, 54, 55]. Its antimicrobial activity is stronger against planktonic bacteria than biofilms, probably because of its interaction with the biofilm exopolymeric matrix, which hinders the molecules’ diffusion towards the microbial cells [56, 57]. It is generally considered less effective than NaOCl [15, 58, 59], although a systematic review of clinical studies found some controversy on this matter [60]. It can reduce the endotoxin levels in infected root canals after chemomechanical preparation, although this effect is also lower than with NaOCl [61]. In addition, it exerts no dissolution effect against either soft or hard tissues [17, 62]. These disadvantages are the main reasons why NaOCl is preferred as the primary irrigant during root canal treatment [2], except in cases of hypersensitivity to NaOCl [63].
Nevertheless, CHX has some important advantages. For instance, it can be adsorbed to negatively charged surfaces such as the dentine matrix and slowly released back into the root canal, thereby maintaining antimicrobial activity for several hours up to a few weeks [64–69]. This property is known as substantivity and is claimed to be CHX’s main advantage over NaOCl [66, 67, 70]. It appears to last longest when the application time of CHX is prolonged [64, 69, 71] or when dentine collagen is exposed in the root canal during application [68]. However, it should be noted that many of the studies on this topic have not examined substantivity under clinically realistic conditions.
Another advantage is that CHX does not react with the collagen in the dentine matrix and consequently does not affect its structure, in contrast to NaOCl [72]. Moreover, it inhibits the bacteria‐related activation of dentine matrix metalloproteinases (MMPs), enzymes involved in collagen‐network degradation within the hybrid layer [73, 74], so it can prolong the integrity and durability of the resin–dentine bond [67]. Finally, CHX has not been associated with adverse side effects when used as a root canal irrigant [53], although it is cytotoxic to human fibroblasts and stem cells of the apical papilla [75, 76] – perhaps even to a greater degree than NaOCl [75, 77]. In addition, it reacts with residual NaOCl in the root canal and forms an orange‐brown precipitate [32, 78].
5.2.3 Ethylenediamine Tetraacetic Acid
EDTA is a chelating agent that binds metal ions, such as Ca++, and forms soluble complexes [79]. Chelation is a self‐limited process, so that when all the available ions have been bound, equilibrium is reached and no more chelation occurs [80]. EDTA is available in the form of various salts, such as disodium and tetrasodium EDTA [79]. The disodium salt at a concentration 15–17% has a neutral or slightly alkaline pH (~7–8), is a strong chelator, and is the most widely used form for root canal irrigation [6, 81]. It can dissolve hard‐tissue debris formed during root canal instrumentation and the inorganic components of the smear layer [82]. The solution is applied for one to five minutes [82–84], within which time it can demineralize dentine to a depth of 20–30 μm [85]. Prolonged contact with dentine can cause excessive demineralization and reduce its microhardness [85, 86].

Figure 5.3 Scanning electron microscope photograph of a contaminated smear layer on dentine (a) before and (b) after treatment with 2.5% NaOCl or (c) after 2.5% NaOCl followed by a final flush with 17% EDTA.
Source: Maria Teresa Arias‐Moliz.
Since EDTA acts only on the inorganic component of debris and the smear layer, it must be combined with NaOCl in order to remove them completely (Figure 5.3) [87, 88]. However, when NaOCl is used after EDTA, it attacks the exposed dentine collagen, causing erosion [89], which appears to be unfavourable for bonding [90, 91]. Moreover, EDTA reacts with NaOCl, causing a rapid loss of the free available chlorine [31, 32, 54, 92]. Consequently, the alternating use of NaOCl and EDTA during instrumentation [93], whether in a liquid or a gel form, is contraindicated. Moreover, gels containing EDTA or other chelators that are used in combination with rotary instruments do not reduce the stress applied to the instruments during root canal preparation, and in some cases may even increase it [94, 95]. Aqueous solutions are far more effective lubricants [94, 95], and this effect is mostly independent of the presence of EDTA [94], so NaOCl is currently considered a sufficient lubricant during rotary instrumentation [96]. Nevertheless, EDTA‐containing gels may still be useful during the initial negotiation of calcified root canals.
EDTA has little or no antimicrobial activity [11, 97, 98], but its chelating action alters the outer membrane of Gram‐negative bacteria [99] and destabilizes the biofilm matrix [57]. It also promotes the detachment of the biofilm [100] and the removal of bacteria present in the smear layer [101]. Some studies have further reported an antifungal action [102, 103]. EDTA exerts a very limited tissue‐dissolving effect [104, 105]. It is less cytotoxic than NaOCl and CHX [75], and it lacks genotoxicity [106], but it affects the function and viability of macrophages [106, 107]. Moreover, it is inexpensive and widely available [108].
In contrast to disodium EDTA, the tetrasodium salt (Na4EDTA) has a higher pH and is a weaker chelator [109]. Thus, when mixed with NaOCl, it does not consume the free available chlorine as rapidly as the disodium salt, and the mixture maintains the properties of both solutions in the short term [105, 110]. However, it should be used immediately, because of the gradual loss of available chlorine over time [105, 111]. Heating of the mixture must be avoided as it accelerates the chlorine loss [112].
5.2.4 Citric Acid
Citric acid (CA) is a weak tricarboxylic acid that can be used instead of EDTA for smear layer removal [113–115]. It sequesters Ca++ ions, forming a soluble chelate. Proposed concentrations range between 1 and 50%, although the most commonly used is 10% (pH 1.1–1.9) [116]. CA is more biocompatible and less cytotoxic than EDTA [117, 118]. It is also widely available and inexpensive. Nevertheless, it leaves precipitated crystals on the root canal wall, which may interfere with the filling [113]; it reduces dentine microhardness, although to a lesser extent than EDTA [119]; it causes more dentine erosion than EDTA and other chelators [89]; and it reacts with NaOCl, thereby rapidly consuming the available chlorine [31]. It has shown some antimicrobial activity against planktonic bacteria [97], which may derive from the alteration of cell membrane permeability due to a decrease in the internal pH of the microbial cells [120]. However, the effect against biofilms is very low [11]. Finally, CA lacks the ability to dissolve pulp tissue [17].
5.2.5 Etidronic Acid
1‐Hydroxyethane 1,1‐diphosphonic acid (HEDP), also known as etidronic acid or etidronate, is a mild chelating agent that has traditionally been used in water treatment [121] and the treatment of patients suffering from osteoporosis or Paget’s disease [122, 123]. It is as effective as EDTA in removing the smear layer, but it requires longer application times (five versus one minute) [84]. It was the first weak chelating agent proposed for use in a mixture with NaOCl during root canal preparation under the concept of continuous chelation, in order to simplify the irrigation protocol [31]. The mixture maintains the desired properties of both compounds in the short term [12, 31, 59, 104, 111,124–126], so it reduces smear‐layer formation and hard‐tissue debris accumulation [124, 125], dissolves pulp‐tissue remnants [104, 126], and exerts a strong antimicrobial effect against biofilms on the dentine surface [59] and inside dentinal tubules [12], even in the presence of hard‐tissue debris or a smear layer [19, 127, 128]. This antimicrobial effect appears to be at least similar to that of a protocol including NaOCl followed by a final rinse with EDTA [16].
HEDP can be obtained in a pure form from various chemical producers, but it is also marketed specifically for root canal irrigation in the form of capsules to be mixed with NaOCl solutions (Dual Rinse HEDP; Medcem GmbH, Vienna, Austria). The mixture remains active for one hour at room temperature [111, 129], but storage at lower temperature maintains the free available chlorine for seven hours; heating has the opposite effect [129]. The presence of HEDP in a mixture does not increase its cytotoxicity [130], and no adverse effects have been observed so far during its clinical use [131]. Nevertheless, other weak chelators that can be used for continuous chelation are also under evaluation and may prove to perform better than it [132, 133].
5.2.6 Maleic Acid
Maleic acid (MA), a mild dicarboxylic acid, has also been proposed as an alternative to EDTA, given its capacity to remove the smear layer [134–137]. It is more biocompatible than EDTA [138, 139] and exerts a greater antimicrobial activity than either EDTA or CA against planktonic bacteria and biofilm [140, 141]. Its antimicrobial effect is also related to the internal pH reduction as a consequence of its low pH (~1.05) [120]. The concentration of choice for root canal irrigation is 7% [138], since higher concentrations can cause intertubular dentine damage [142].
5.2.7 Ozonated Water
Ozone (O3) is a very reactive gas that is normally found in the atmosphere but can also be produced by a generator [143]. In the presence of water, it forms oxygen radicals, which penetrate the cell membranes, disturbing the osmotic balance and promoting the oxidation of aminoacids and nucleic acids [144]. Thus, ozonated water exerts an antimicrobial effect against bacteria, fungi, protozoa, and viruses, which motivates its use in water processing [145], the food industry [146], and the treatment of some diseases [147, 148]. However, its effectiveness against root canal infections is limited. A number of studies have shown that ozonated water is clearly less effective than NaOCl against both planktonic bacteria and biofilms [143, 149‐151]. Moreover, it cannot neutralize endotoxin [152]. A recent systematic review concluded that, despite its low toxicity, ozonated water is not indicated for use as a root canal irrigant, whether as a replacement or an adjunct to NaOCl [153].
5.2.8 Electrochemically Activated Water
Electrochemically activated water (EAW) is produced by electrolysis of tap water or low‐concentration salt solutions, such as saline, in special devices – a process similar to that used for large‐scale production of NaOCl [154]. Two types of solutions are produced: an anolyte and a catholyte. The anolyte seems to contain HOCl and OCl−, and its bactericidal potential is largely attributed to the presence of free available chlorine [155]. However, its concentration is typically much lower than that of NaOCl solutions [156]. The catholyte is alkaline and is claimed to have a cleaning or detergent effect [155, 157]. The antimicrobial effect of EAW against biofilms may be similar to that of NaOCl in the absence of dentine [158], but it is clearly inferior to it inside root canals [154, 159, 160]. In addition, EAW cannot dissolve pulp tissue [161].
5.2.9 Saline
Physiologic saline (0.9% NaCl) is an inert solution with no direct antimicrobial effect and no ability to dissolve pulp‐tissue remnants or hard‐tissue debris [2, 17]. It is often used as a control solution in experiments. Despite its lack of chemical activity, it can still exert a mechanical cleaning effect when flowing inside the root canal system [162, 163], and it can act as a lubricant for rotary instruments [94, 95]. Nevertheless, it should not be used as a primary irrigant, only for intermediate flushing to prevent contact between two irrigants that react with each other (e.g. NaOCl and CHX) [164] or where there is a temporary need for an inert irrigant (e.g. after a NaOCl accident) [165].
5.2.10 Mixtures of Irrigating Solutions
None of the available irrigating solutions can satisfy all the requirements outlined at the beginning of this chapter, so mixtures containing more than one have been developed. Many of these include an antimicrobial or a chelating agent together with one or more surfactants, the latter being added in order to reduce the surface tension. Examples include Chlor‐XTRa (Vista Dental, Racine, WI, USA), which contains 6% NaOCl, CHX‐Plus (Vista Dental, Racine, WI, USA), which contains up to 2% CHX, and SmearClear (SybronEndo, Orange, CA, USA), which contains EDTA. A common misconception amongst dentists is that a reduction of the surface tension can improve the penetration of the mixture in the root canal system [166–169]. However, a decrease in the surface tension of an irrigant does not provide any clinically‐relevant advantage. Surface tension only acts on interfaces between immiscible fluids, and there are no such interfaces limiting irrigant penetration in the root canal in vivo [170]. Nonetheless, several surfactants exert some direct antimicrobial effect independent of the surface tension reduction. Cetrimide (CTR), for example – a cationic surfactant widely used in mixtures with common irrigants – is known to be bactericidal and also decreases a biofilm’s mechanical stability by weakening the cohesive forces within the exopolymeric matrix [171]. This additional effect may not be detectable in mixtures with strong antimicrobial solutions (e.g. NaOCl at high concentration), but it does seem to improve the antimicrobial action of weaker ones (e.g. CHX, EDTA, CA, MA) [15, 68, 141, 172, 173]. Its mixtures with chelators also seem to have a long residual effect [174, 175].
The literature shows that the addition of surfactants does not enhance the antimicrobial effect of NaOCl at high concentrations [172, 176, 177], nor its tissue‐dissolution capacity [178–180]. To the contrary, it may actually accelerate the loss of free available chlorine [181]. The combination of 2% CHX with surfactants (CHX‐Plus; Vista Dental) appears to have a stronger effect against biofilm than CHX alone [172, 182], but this is likely due to the additional antimicrobial effect of the surfactants [172]. Removal of calcium from dentine and removal of the smear layer also seem to be unaffected by the addition of surfactants to EDTA [183–185]. The same rationale applies to other mixtures containing surfactants together with antimicrobials and chelators (see later).
5.2.10.1 BioPure MTAD
BioPure MTAD (Denstply Sirona, Charlotte, NC, USA) is a mixture of 3% doxycycline, 4.25% CA, and 0.5% surfactant (Tween 80) [186]. It has been recommended for a final five‐minute rinse (instead of EDTA) following chemomechanical preparation with 1.3% NaOCl in order to remove the smear layer [186] and supplement the disinfection of the root canal system [187, 188]. The removal of the smear layer has been attributed to the CA and doxycycline, but also to the low pH of the mixture (~2) [116, 187, 189]. Although some studies found that it caused only minimal changes in the dentinal tubule structure [187] and limited erosion [190], others reported that it demineralized dentine more aggressively than EDTA [191].
The antimicrobial activity of MTAD stems from the doxycycline, which has a bacteriostatic effect as well as a residual antimicrobial one over time (substantivity) [192, 193]. However, there is some controversy regarding the magnitude of these effects. Some studies – especially the earlier ones – reported very effective elimination of root canal bacteria [188,194–197], but several others could not detect any advantage over commonly used irrigants such as NaOCl [98,198–205]. It was also shown that irrigation with NaOCl prior to MTAD could reduce its substantivity considerably [201].
MTAD cannot dissolve pulp‐tissue remnants [205]. Moreover, despite its good biocompatibility [206], it may cause tooth discolouration [207]. It is contraindicated during pregnancy, in children under eight years of age, and in patients with an allergy to doxycycline. It is also more expensive and has a shorter shelf‐life than other commonly used root canal irrigants [208].
5.2.10.2 Tetraclean
Tetraclean (TC) (Ogna Laboratori Farmaceutici, Muggiò, Italy) is a mixture similar to MTAD but with a lower concentration of doxycycline (1%), a higher concentration of CA (10%), and two different surfactants: propylene glycol and cetrimide [168, 209–213]. It has also been proposed for a final five‐minute rinse after NaOCl [209, 210] because it can remove the smear layer [168, 211] and it exerts an antimicrobial effect against the residual bacteria in the root canal [168, 209, 211]. This antimicrobial effect seems to be stronger than that of MTAD – probably because of the CTR in its composition [209, 210] – but still weaker than that of NaOCl [209]. Its substantivity appears to be greater than that of MTAD [212, 213], but it is decreases by a prior irrigation with NaOCl [214]. It has no tissue‐dissolving capacity [209, 210].
The presence of doxycycline in the mixture can lead to similar problems to those of MTAD, such as tooth staining, bacterial resistance, and hypersensitivity [189, 207]. In order to overcome these, a modified mixture without any antibiotic was developed (Tetraclean NA; Ogna Laboratori Farmaceutici). Despite the absence of tetracycline, the modified mixture appears to exert a stronger antimicrobial effect against bacteria inside dentinal tubules compared to MTAD [203].
5.2.10.3 QMix
QMix (Denstply Sirona, Charlotte, NC, USA) is a mixture of 2% CHX, 17% EDTA, and CTR. It has a slightly alkaline pH and has been recommended as a final irrigating solution applied for two to five minutes after NaOCl in order to remove the smear layer and kill the remaining bacteria [205, 215]. It is as effective as EDTA in regard to chelation [205, 215]. It reduces the microhardness of dentine but doesn’t seem to cause erosion [89]. It is more effective against biofilms than CHX and MTAD [205, 216], but less so than NaOCl [217–219]. It has also demonstrated a prolonged substantivity because of the presence of CHX in its composition [220], as well as good biocompatibility [221, 222].
5.2.11 Suggested Irrigation Protocol
In the absence of a single solution possessing all the characteristics of an ideal irrigant, it is necessary to use at least two in order to accomplish all the aims of root canal irrigation. There is a wide consensus that NaOCl should be used as the primary irrigant during access and chemomechanical preparation of the root canal [1, 2, 4, 6]. Frequent exchange with copious amounts of fresh irrigant and an extended application time may be required for the effective diffusion of the solution, its antimicrobial action against bacteria organized in biofilms, and the dissolution of pulp‐tissue remnants and of the organic components of the smear layer. NaOCl is also considered an excellent means of lubrication for rotary instruments [94, 95]. Although hard‐tissue debris packed in uninstrumented parts of the root canal system and the inorganic components of the smear layer also need to be removed, the alternating use of NaOCl and strong chelators during instrumentation is contraindicated, as already explained [31, 92, 132].
A chelator such as EDTA can be applied for a short time after instrumentation to achieve these goals and to partially disrupt the biofilm [57, 82, 100], but this step should not be considered a final rinse. Hard‐tissue debris and the smear layer need to be removed mainly in order to allow NaOCl to penetrate deeper into uninstrumented areas and inside dentinal tubules, and NaOCl should always be reapplied after their removal in order to maximize its antimicrobial effect. Alternatively, a mixture of NaOCl and a weak chelator (e.g. HEDP) could be used throughout chemomechanical preparation for continuous chelation [16, 31, 132], but more evidence is needed regarding its performance and possible adverse effects.
It should be emphasized that the final irrigation protocol determines the condition of the dentine surface immediately prior to obturation, so it should take into account the requirements of the obturation materials. For instance, a final rinse with NaOCl seems to dissolve the exposed dentine collagen, which may be detrimental for certain types of root canal sealers that bind to these collagen fibres [90, 223, 224]. However, opposing views have also been expressed [225, 226]. A possible workaround could be to irrigate again with a chelator as a final step before root canal filling in order to expose a new layer of collagen.
5.3 Irrigation Techniques
Rather than categorizing irrigation techniques as either ‘manual’ or ‘mechanically assisted’ – a distinction that has very little practical use – it is more reasonable to categorize them as either ‘irrigant delivery’ or ‘irrigant activation/agitation’, because these two types of techniques serve different purposes. Delivery techniques aim to transport large amounts of irrigant primarily into the main root canal, whilst activation/agitation techniques aim to distribute an irrigant into the rest of the root canal system and to enhance cleaning and disinfection.
There seems also to be some confusion in the literature regarding the terms ‘activation’ and ‘agitation’, which are often used interchangeably. Several irrigation techniques are able to agitate an irrigant inside the root canal, albeit with varying degrees of effectiveness, but not all of them can actually activate one in a chemical way. Therefore, in this chapter, the term ‘activation’ will be reserved for those techniques that can produce transient cavitation bubbles which, upon collapse, may accelerate chemical reactions in their vicinity [227, 228]. All the irrigants described in the previous section may be transported with any of the delivery techniques described here, and most can be agitated/activated without problems; some notable exceptions are detailed later.
5.3.1 Irrigant Delivery Techniques
5.3.1.1 Syringe Irrigation
Syringe irrigation is a widely used technique for the delivery of irrigants inside root canals. It remains the most popular technique amongst both endodontists and general dentists [6, 81, 229, 230], possibly due to its simplicity and low cost and the wide availability of syringes and needles. Thus, it can be considered the current clinical standard. During syringe irrigation, an irrigant is transferred from the syringe to the root canal through a needle. The irrigant flows inside the root canal from the apical third towards the coronal third and normally exits through the root canal orifice, where it is usually evacuated by an aspirator tip. The cause of the flow is the pressure built up inside the syringe barrel due to the force applied to the plunger by the clinician. The positive pressure drives the irrigant through the needle, for which reason syringe irrigation is categorized as a positive‐pressure irrigation method [231, 232].
5.3.1.1.1 Syringes
Syringes used for root canal irrigation have a capacity between 1 and 20 ml (Figure 5.4) [233–240]. Apart from practical considerations such as the frequency of refilling, the choice of the syringe also depends on the strength of the clinician, the size and length of the needle, and the desired flow rate [170]. A larger syringe, a finer or longer needle, and a higher desired flow rate all require a stronger force to be applied by the clinician on the syringe plunger during irrigation [170]. Syringes with a capacity of 5–10 ml appear to be a reasonable compromise. Battery‐operated automated syringes have appeared on the market lately and may facilitate irrigation at a stable flow rate, but there are no studies as yet confirming this potential advantage. A secure Luer‐Lock threaded fitting is necessary for all syringes and needles used for root canal irrigation, due to the high pressure inside the syringe barrel [241] which could lead to accidental detachment during irrigation [26].

Figure 5.4 Syringes used for root canal irrigation. From top to bottom: 20, 12, 5, and 2.5 ml. All have a Luer‐Lock threaded fitting (arrow).
Source: Reprinted with permission from Springer. Boutsioukis, C., van der Sluis, L.W.M. Syringe irrigation: blending Endodontics and Fluid Dynamics. Chapter 3. In Basrani, B. ed. Endodontic Irrigation: Chemical Disinfection of the Root Canal System. New York, NY, USA: Springer, 2015:45–64.
5.3.1.1.2 Needles
A wide variety of needles have been suggested for irrigant delivery during root canal treatment [235, 237,242–247]. These are typically made of stainless steel (Figure 5.5), but NiTi [248] and, more recently, plastic (Figure 5.6) have also been used in order to increase flexibility and facilitate irrigation in curved root canals. The diameter or size of a needle is often reported in ‘gauge’ units [248, 249]; a larger gauge number corresponds to a finer needle (Table 5.1). There is still no universally accepted colour coding for needles [248], and each manufacturer is free to decide whether their colour represents their size, length, or even type. Large needles (21–25G) were commonly employed in the past for syringe irrigation [236,250–254]. Evidently, these could only be inserted in the pulp chamber and the coronal third even in wide root canals, so the irrigant was only delivered to these areas and very small amounts actually reached the apical third of the root canals, either carried by instruments or as a result of diffusion [170].

Figure 5.5 Various types of 30G stainless‐steel needles used for root canal irrigation. Open‐ended needles: (a) flat; (b) bevelled; (c) notched. Closed‐ended needles: (d) side‐vented; (e) double side‐vented. Variable views and magnifications have been used to highlight differences in tip design.
Source: Reprinted and modified with permission from Elsevier. Boutsioukis, C., Verhaagen, B., Versluis, M., Kastrinakis, E., Wesselink, P., van der Sluis, L.W.M. Evaluation of irrigant flow in the root canal using different needle types by an unsteady Computational Fluid Dynamics model. J. Endod. 2010;36:875–9.

Figure 5.6 Flexible 30G double side‐vented Irriflex needle made of plastic.
Source: Courtesy Produits Dentaires SA, Vevey, Switzerland.
Nowadays, the importance of irrigant delivery to the full extent of the root canal has been recognized, and the use of smaller needles (27–31G) that can reach farther is widely advocated [2255–259]. At present, the 30G needle could be considered the standard, but slightly larger and smaller ones are also in use. The main disadvantage of smaller needles is that they require a much stronger force to be applied on the syringe plunger during irrigation to reach the same flow rate; even a minor decrease in the diameter of a needle leads to a substantial increase in the force required [170]. Still, given the current trends in root canal instrumentation [260], finer needles (>30G) may become the standard in the future.
Table 5.1 Medical needle specifications according to ISO 9626:1991/Amd.1:2001 [249] and corresponding sizes of root canal instruments (non‐existent instrument sizes rounded up to the next available size).
Source: Modified from ISO 9626. Stainless Steel Needle Tubing for Manufacture of Medical Devices. Amendment 1. Geneva, Switzerland: International Organization for Standardization, 2001:1–5.
Gauge size | Metric size (mm) | Ext. diameter (mm) | Corresponding instrument size | |
Min | Max | |||
21 | 0.80 | 0.800 | 0.830 | 90 |
23 | 0.60 | 0.600 | 0.673 | 70 |
25 | 0.50 | 0.500 | 0.530 | 55 |
26 | 0.45 | 0.440 | 0.470 | 50 |
27 | 0.40 | 0.400 | 0.420 | 45 |
28 | 0.36 | 0.349 | 0.370 | 40 |
29 | 0.33 | 0.324 | 0.351 | 35 |
30 | 0.30 | 0.298 | 0.320 | 35 |
31 | 0.25 | 0.254 | 0.267 | 30 |
Irrigation needles generally belong to one of two categories: needles that allow the irrigant to flow straight through their tip irrespective of its particular shape (open‐ended) and needles with a closed tip that prevents the direct outflow of the irrigant, so that it flows through one or more side vents (closed‐ended) (Figure 5.5) [247]. Open‐ended needles create an intense jet of irrigant, which penetrates apically (Figure 5.7) [247, 261]. The penetration depends on the apical size and taper of the root canal and the irrigant flow rate (Figure 5.8) [247, 256, 261, 262]. The irrigant cannot reach farther than 1 mm apically to the needle tip in root canals with an apical size less than 30 [263, 264], but penetration is greatly improved in larger canals [256, 261, 262, 264, 265]. An increase in the apical size also reduces the risk of inadvertent irrigant extrusion through the apical foramen [266].
Closed‐ended needles can only create a low‐intensity jet, which is diverted towards the root canal wall (Figure 5.7) [247]. The irrigant can generally penetrate up to 1 mm apically to the needle tip (Figure 5.8) [247], and this distance can only be increased very little by increasing the apical size, the taper, or the irrigant flow rate [261, 262, 264, 265, 267]. Thus, closed‐ended needles are considered less effective than open‐ended ones in terms of apical irrigant penetration and exchange [247, 256, 262, 264, 265, 268]. Another disadvantage is the strongly asymmetric flow that concentrates the mechanical cleaning effect on a small part of the root canal wall facing the vent(s) proximal to the tip [244, 247, 257]; most of the irrigant flows through these vents [245, 247, 262]. Therefore, needle orientation must be taken into account during use. However, closed‐ended needles seem to extrude less irrigant through the apical foramen [266, 269].
5.3.1.1.3 Syringe Warmers
Various types of syringe warmers have been suggested in order to heat the irrigant prior to delivery into the root canal [270]. Early studies showed that 1% NaOCl at 45 or 60 °C was able to dissolve as much pulp tissue as a 5.25% solution at 20 °C [18]. Yet, more recent work [271], including a clinical study [42], has shown that the temperature of the irrigant inside the root canal is increased only during delivery; afterwards, it decreases rapidly to 37 °C, which is the physiological core body temperature. Therefore, preheating has only a very short‐term effect with limited clinical significance, and there is little justification for the use of such devices. Moreover, the use of rotary NiTi instruments in contact with preheated NaOCl may decrease their resistance to cyclic fatigue considerably [272–274].

Figure 5.7 Time‐averaged contours (left) and vectors (right) of irrigant velocity in the apical part of a size 45/0.06 taper root canal during syringe irrigation by different types of needles, according to computer simulations. Open‐ended needles: (a) flat; (b) bevelled; (c) notched. Closed‐ended needles: (d) side‐vented; (e) double side‐vented. All needles are positioned at 3 mm short of working length and are coloured in red.
Source: Reprinted and modified with permission from Elsevier. Boutsioukis, C., Verhaagen, B., Versluis, M., Kastrinakis, E., Wesselink, P., van der Sluis, L.W.M. Evaluation of irrigant flow in the root canal using different needle types by an unsteady Computational Fluid Dynamics model. J. Endod. 2010;36:875–9.
5.3.1.1.4 Technique
In order for the irrigant to penetrate to the apical terminus of the main root canal, needles must be placed close enough to working length without binding and the root canal must be adequately enlarged. Binding of a needle – especially an open‐ended one – very close to working length and interruption of the reverse flow towards the root canal orifice could lead to inadvertent irrigant extrusion through the apical foramen, so this should be carefully avoided at all times [269]. Open‐ended needles should be placed at 2–3 mm short of working length, but closed‐ended needles must placed even closer (within 1 mm) [247, 256, 261, 262, 264, 265]. A minimum apical size of 30–35 is required for 30G needles to reach these positions. Enlargement of the root canal up to this size is also important for irrigant penetration apically to the needle due to the viscosity of irrigants, which limits their flow in narrow spaces [170]. Root canal taper is less important for irrigant penetration [264, 265].
During irrigant delivery, the needle should be moved in and out along the root canal without exceeding the desired insertion depth. Apart from preventing needle binding, such movement can improve the mechanical cleaning of the canal, not because of agitation, but because the maximum mechanical cleaning is concentrated on a limited area near the tip of the needle [247, 256, 257, 264, 265]. Finally, the irrigant should be delivered at an adequate flow rate (0.15–0.20 ml/s) – a very low one (<0.05 ml/s) might be unable to drive the irrigant even up to the tip of a closed‐ended needle [267].

Figure 5.8 Time‐averaged irrigant velocity contours (left) and vectors (middle) and stream‐lines (right) depicting irrigant flow in a size 45/0.06 taper root canal when a closed‐ended (top) or open‐ended (bottom) needle is placed at 1–5 mm short of working length, according to computer simulations. Needles are coloured in red.
Source: Reprinted with permission from Elsevier. Boutsioukis, C., Lambrianidis, T., Verhaagen, B., et al. The effect of needle insertion depth on the irrigant flow in the root canal: evaluation using an unsteady Computational Fluid Dynamics model. J. Endod. 2010;36:1664–8.
Syringe irrigation seems to be effective in the main root canal, provided that the requirements concerning root canal size, needle insertion depth, and irrigant flow rate are satisfied. Several ex vivo studies and one clinical trial did not find any significant difference between syringe irrigation and a variety of other methods, including negative‐pressure irrigation, sonic activation, and ultrasonic activation, regarding the removal of soft‐tissue remnants, hard‐tissue debris, bacteria, and biofilm from the main root canal or the healing of apical periodontitis [275–283]. Several further studies could not find any difference between syringe irrigation and other methods, although it was unclear whether the aforementioned requirements were met [284–288]. Studies showing inferior cleaning and disinfection of the main root canal by syringe irrigation compared to other methods generally did not enlarge the canals to an adequate size or placed the needles too far from the working length [257,289–293]. Therefore, syringe irrigation seems to be sufficient for teeth with a single root canal and simple anatomy. Nonetheless, the irrigant appears unable to penetrate anatomic intricacies such as fins and uninstrumented oval extensions [294–302], the isthmus [279, 282, 284,303–306], and accessory canals [307–310], so other irrigation methods – especially irrigant activation methods – may be necessary in cases with more complex anatomy.
5.3.1.2 Negative‐Pressure Irrigation
Negative‐pressure delivery techniques are sometimes categorized erroneously as agitation or activation techniques. During negative‐pressure irrigation, the irrigant is delivered in the pulp chamber and negative pressure (suction) is applied on the distal end of a cannula placed inside the root canal, so that the irrigant is drawn along the canal from the coronal towards the apical third, where it is evacuated [290, 311]. Negative‐pressure irrigation is not a new idea in endodontics [312], but it was reintroduced as an alternative to syringe irrigation in order to remove entrapped air bubbles from the apical third of the root canal (Apical vapor lock) [313] and to minimize the risk of irrigant extrusion through the apical foramen [290, 312] under the assumption that NaOCl accidents are caused by the increased irrigant pressure near the apical foramen. Both of these arguments have since been questioned [165, 314]. Its popularity appears to be much lower than that of syringe irrigation and other supplementary irrigation techniques [6, 230].
5.3.1.2.1 Cannulas and Systems
A variety of cannulas, both open‐ and closed‐ended, can be used for negative‐pressure irrigation, including needles normally used for syringe irrigation (Figure 5.9) [311, 315] as long as there is a suitable adaptor to fit them on the suction hose of the dental unit. Nevertheless, they need to be fine enough to reach the apical third of the root canal, or even full working length, without binding [311]. They are made of stainless steel or plastic and usually have a cylindrical shape, although tapered ones are also available; tapering reduces the resistance to the flow [311]. In order to circumvent the frequent problem of clogging during use, multivented cannulas have been developed, so that if one of the vents is clogged the flow is not completely stopped.
A negative‐pressure irrigation system may also include some means of irrigant delivery to the pulp chamber (usually a syringe and needle) and an additional suction hose to evacuate the overflow, together with the necessary tubes and connectors.
5.3.1.2.2 Technique
Placement of cannulas in the apical third of the root canal and effective suction of the irrigant require sufficient enlargement of the root canal. Closed‐ended cannulas should be placed at working length without binding, so if a 30G cannula is used, the canal should be enlarged at least to an apical size 35–40/0.04 taper [231, 316]. Open‐ended cannulas can be placed slightly farther away, at 2 mm from working length, without compromising irrigant exchange [311], but these are often larger, so the root canal should also be enlarged almost to the same size and taper.
There are no strict protocols for most negative‐pressure irrigation systems [311, 315]. However, the manufacturer of the EndoVac system (Kerr, Brea, CA, USA) recommends a specific protocol for its use [317] in order to ensure consistency between clinicians and between the various studies evaluating it. A larger plastic open‐ended cannula is initially used to irrigate the pulp chamber and the coronal and middle third of the root canal in order to remove larger particles, before applying a finer cannula apically. This additional step reduces the chances of clogging the finer cannula, but even the larger one may be clogged by debris [316]. The finer cannula is subsequently inserted at working length. In both cases, the irrigant is simultaneously delivered in the pulp chamber by a syringe and the overflow is evacuated by a separate suction hose. All of the steps in this protocol are standardized in terms of time (delivery time and rest time) but not in terms of irrigant volume delivered in the root canal, arguably because it is difficult to determine exactly how much irrigant flows through each part of this complex system during irrigation in vivo. Therefore, the protocol relies on a standardized flow rate through each cannula – although in reality this may fluctuate due to clogging, depletion of the irrigant in the root canal, or changes in the suction pressure used to drive the system.

Figure 5.9 Cannulas used for negative pressure irrigation: (a) 30G closed‐ended microcannula of the EndoVac system (Kerr, Brea, CA, USA); (b) 28G open‐ended iNP cannula (Mikuni Kogyo, Nagano, Japan). Variable magnifications have been used to highlight differences in tip design.
Source: Christos Boutsioukis. iNP cannula photo courtesy of Dr Carlos Adorno, Asunción, Paraguay.
Dental suction pumps maintain a negative pressure of approximately 20–25 kPa [231, 311], but this is rarely monitored during everyday use. Any decrease in pressure causes a proportional decrease in the irrigant flow rate through the cannulas, which may be difficult to detect. Moreover, the maximum flow rate though a cannula during negative‐pressure irrigation is approximately 4–16 times lower than that through a needle of the same type and size used for syringe irrigation [231, 241, 311]. This is mainly attributed to the much lower driving pressure (20–25 kPa); the positive pressure developed inside the syringe reaches up to 540 kPa [241]. As a consequence, the irrigant exchange is much slower during negative‐pressure irrigation, and the mechanical cleaning effect is reduced accordingly [261].
Despite claims that negative‐pressure irrigation is generally superior to syringe irrigation [290, 316, 318], there seems to be no solid evidence to support this view. Negative‐pressure systems have been often compared to suboptimal syringe‐irrigation protocols; these comparisons are thus inherently biased [319]. Taking this into account, negative‐pressure irrigation doesn’t seem to be better than syringe irrigation in terms of cleaning and disinfection of the main root canal, although it may result in improved cleaning of the apical part of the isthmus [319]. A notable advantage of negative‐pressure systems is that they extrude much less irrigant through the apical foramen than does syringe irrigation [22]. This difference may not be clinically relevant in most cases, but it could become relevant in cases where a NaOCl accident has already occurred, so that the risk of another one is high [165].
5.3.1.3 Combined Positive‐ and Negative‐Pressure Irrigation
A combination of syringe irrigation and negative‐pressure irrigation has been proposed as a way to improve the cleaning of isthmuses ex vivo [320]. The two techniques are applied simultaneously in the two root canals associated with the isthmus in order to force the irrigant from one, through the isthmus, and into the other. This combination appears to remove more hard‐tissue debris from the isthmus than either technique alone, or than ultrasonic activation [320]. Nonetheless, clinical application of this combined technique may be difficult because the clinician must apply and control irrigant delivery and evacuation in two different root canals using two different techniques at the same time.
5.3.2 Irrigant Activation and Agitation Techniques
5.3.2.1 Ultrasonic Activation
Ultrasonic activation is the most widely used irrigant activation/agitation method [6, 81], so it could also be considered a clinical standard. It relies on the transverse oscillation of an ultrasonic instrument inside an irrigant‐filled root canal [321] at a frequency of approximately 25–32 kHz [322]. Instrument oscillation drives the surrounding irrigant and induces a complicated flow inside the root canal [297, 323, 324], which consists of an oscillatory component and a steady component; the latter is also known as ‘acoustic streaming’ [325]. This intense flow agitates the irrigant in the main canal, transports it to remote areas of the root canal system, and improves the mechanical cleaning [326]. The rapid instrument motion and the resulting irrigant pressure changes may also give rise to acoustic cavitation (formation, behaviour, and collapse of bubbles) [227, 327, 328]. Transient acoustic cavitation may be particularly useful for root canal cleaning because of the shockwaves emitted [327], the high shear stress applied on surfaces, and the local increase of pressure and temperature, which might give rise to sonochemical effects [227, 228,327–329]. A part of the kinetic energy is inevitably converted to heat and increases the temperature of the irrigant [330–332], which may also accelerate chemical reactions [18, 34]. Thus, ultrasonic activation may augment both the chemical and the mechanical effects of irrigation [5].
5.3.2.1.1 Ultrasonic Instruments
Various types of ultrasonic files, tips, and wires made of either stainless steel or NiTi have been used for irrigant activation (Figure 5.10). Smooth wires were proposed for this purpose in order to avoid unwanted dentine removal [333], but it appears that they are not immune to this problem [334]. They are made from either a tapered or a nontapered rod with a circular cross‐section. Ultrasonic K‐files are made by twisting a tapered (2%) blank with a square cross‐section, providing sharp edges along the instrument. It should be emphasized that these files were originally designed for dentine cutting rather than irrigant activation. K‐files show a gradual decrease in the oscillation amplitude of the antinodes from the free end towards the handpiece, due to their taper [322]. Irrisafe files (Acteon Satelec, Merignac, France) were introduced more recently as an attempt to adapt K‐files to the requirements of irrigant activation. They have almost no taper, a larger pitch, rounded edges, and a blunt tip, in an effort to minimize unintentional removal of dentine [335]. Nevertheless, some dentine may still be removed during use [334, 336]. Due to the absence of taper, Irrisafe files have almost equal oscillation amplitude at all antinodes along their length [322, 337]. Ultrasonic K‐files and Irrisafe files appear to be almost equally popular, whilst smooth wires are less so [338].

Figure 5.10 Ultrasonic instruments commonly used for irrigant activation: (a) smooth wire (Endo Soft Instrument (ESI), Electro Medical Systems, Nyon, Switzerland); (b) K‐file (Acteon Satelec, Merignac, France); (c) Irrisafe file (Acteon Satelec, Merignac, France).
Source: Christos Boutsioukis.
5.3.2.1.2 Ultrasound Devices
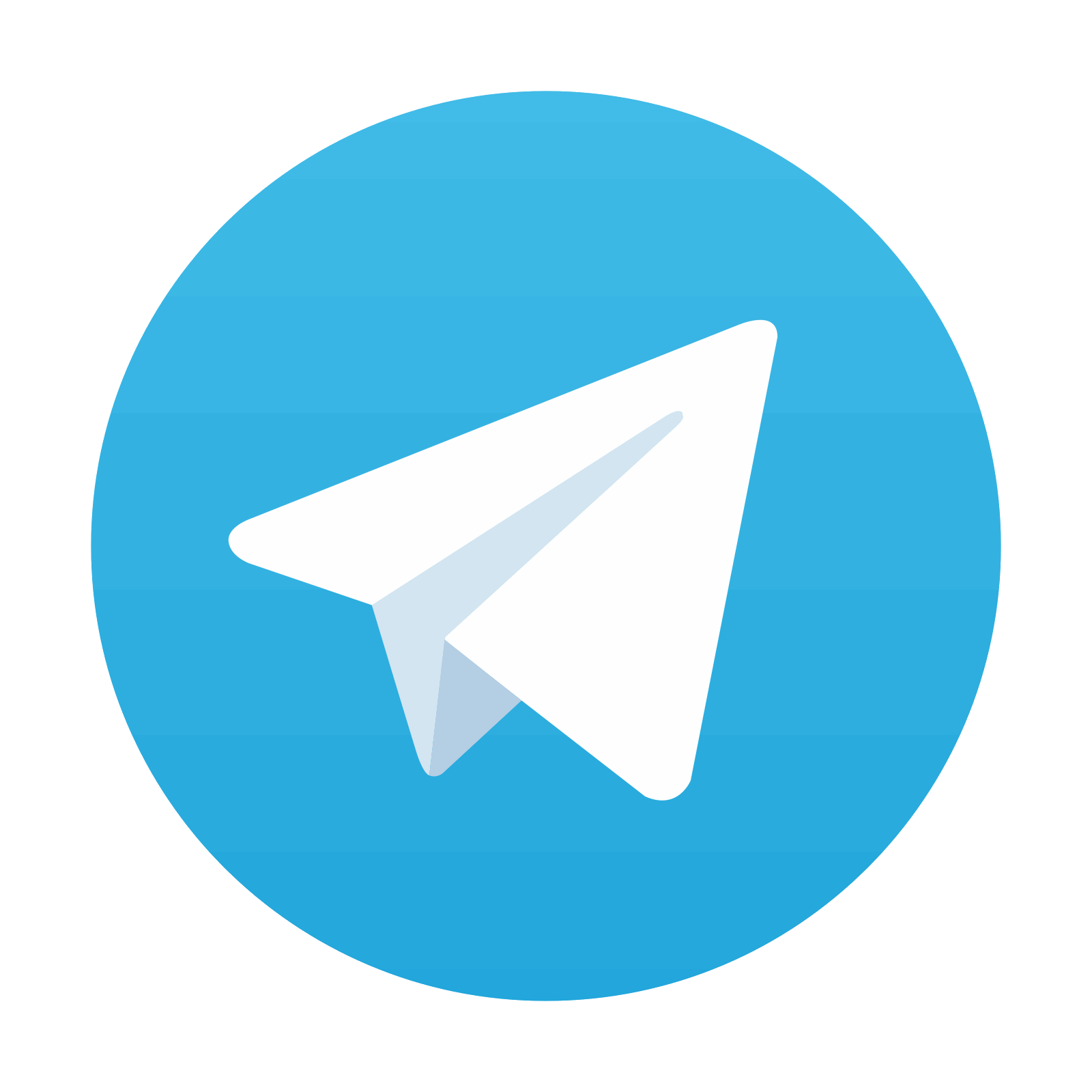
Stay updated, free dental videos. Join our Telegram channel

VIDEdental - Online dental courses
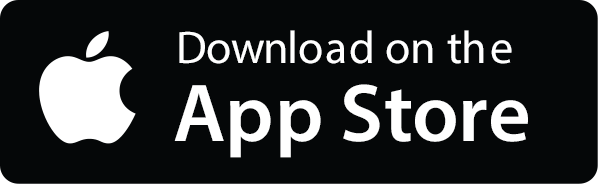
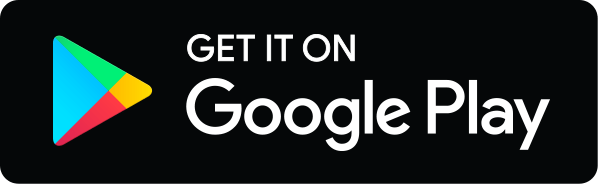