Fig. 2.1
Basic chemical structure of bisphosphonates
2.3 Mechanism of Action and Pharmacodynamics
In humans, PPi is released as a by-product of many of the body’s synthetic reactions; thus, it can be readily detected in many tissues, including blood and urine [4]. Pioneering studies from the 1960s demonstrated that PPi was capable of inhibiting calcification by binding to hydroxyapatite crystals, leading to the hypothesis that regulation of PPi levels is the mechanism by which bone mineralization is regulated [5]. Although the various bisphosphonates share many pharmacological features with PPi, important biochemical differences exist among them, particularly in the way in which they bind to bone mineral and their effect on bone resorption. Accordingly, bisphosphonate skeletal retention depends on the availability of hydroxyapatite binding sites. Bisphosphonates are preferentially incorporated into sites of active bone remodeling, as typically occurs in conditions characterized by accelerated skeletal turnover. They are rapidly cleared from the systemic circulation and deposited on bone mineral surfaces, particularly at sites of osteoclast activity. In fact, osteoclasts in the resorption phase are in a highly acidic microenvironment, which may facilitate bisphosphonate release from the bone surface, giving rise to high local bisphosphonate concentrations [2]. The bisphosphonate may then be internalized into the osteoclast, culminating in direct inhibition of osteoclast activity. This inhibition may be a consequence of a direct toxic effect involving osteoclast retraction, condensation, and cellular fragmentation, resulting in apoptosis. Alternatively, inhibition may be due to a disturbance of intracellular vesicular trafficking by the bisphosphonate [6], which may then lead, for example, to disorganization of the actin cytoskeleton and loss of actin rings or to disturbances of osteoclast ruffled-border formation [2] (Fig. 2.2).
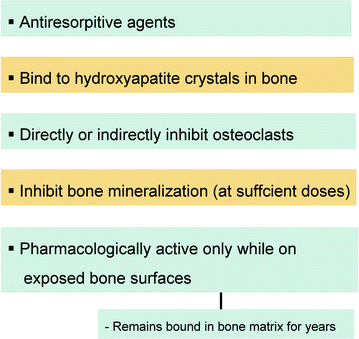
Fig. 2.2
Summary of mechanisms of action of bisphosphonates
Other cell types that internalize bisphosphonates by endocytosis are osteoblasts, macrophages, epithelial and endothelial cells, circulating monocytes, and neoplastic cells, such as myeloma and prostate tumor cells [7].
Based on recent discoveries concerning their molecular mechanism of action, bisphosphonates can be grouped in different classes: first-generation non-nitrogen-containing bisphosphonates (BPs), second- and third-generation nitrogen-containing bisphosphonates (N-BPs) (Fig. 2.3) and the phosphonocarboxylate analogue 3-PEHPC, previously known as NE10790 [8] (Fig. 2.4). First-generation BPs, such as clodronate and etidronate, are metabolized intracellularly to analogues of ATP. The intracellular accumulation of these metabolites within osteoclasts inhibits their function and induces apoptosis, very likely by inhibiting ATP-dependent enzymes [7]. In contrast, second-generation N-BPs, such as pamidronate and alendronate, and third-generation N-BPs, such as risendronate, ibandronate, and zoledronate, interfere with other metabolic reactions, notably those in the mevalonate biosynthetic pathway, by inhibiting farnesyl diphosphate (FPP) synthase. The inhibition of FPP synthase prevents the prenylation of small GTPases (e.g., Ras, Rho, Rab), which are important signaling proteins (Fig. 2.5). Phosphonocarboxylate analogue of the bisphosphonate risendronate, 3-PEHPC, in which one of the phosphonate groups is replaced with a carboxylate group, inhibits other enzymes of the mevalonate pathway that use isoprenoid lipids (Fig. 2.6). In fact, 3-PEHPC specifically inhibits prenyl transferase Rab GGTase, thus preventing the prenylation and membrane localization of only the Rab GTPases, with no effect on FPP synthase. Due to the loss of one of the phosphonate groups, thus allowing the binding of only one calcium ion, 3-PEHPC is a comparatively weak inhibitor of bone resorption [8, 9].
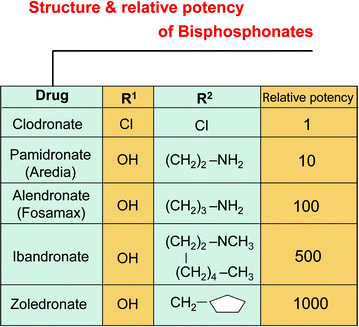
Fig. 2.3
Bisphosphonates are classified as first-, second-, and third-generation, implying progressively greater potencies. Clodronate is in the first category, pamidronate and alendronate in the second, and zoledronate in the third
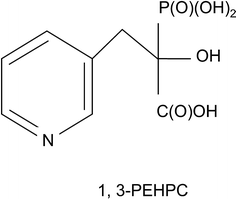
Fig. 2.4
Basic chemical structure of the phosphonocarboxylate analogue 1,3-PEHPC
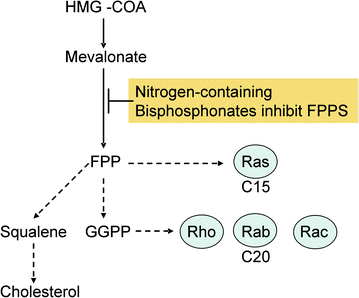
Fig. 2.5
Mechanism of action of nitrogen-containing bisphosphonates
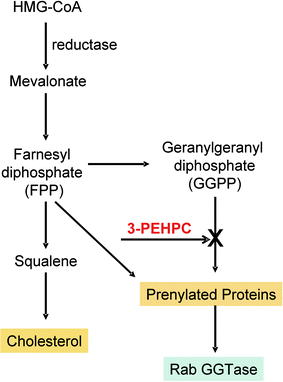
Fig. 2.6
Mechanism of action of 1,3-PEHPC
2.4 Pharmacokinetics
Bisphosphonates have several common properties, including poor intestinal absorption, high affinity for bone mineral, inhibitory effects on osteoclastic bone resorption, prolonged bone retention, and elimination in the urine.
Absorption is impaired by food, especially foods containing calcium, so bisphosphonates should be given when fasting and then only with water, without dairy products, orange juice, or drinks containing caffeine. The patient should not eat for at least 30 min (alendronate, risendronate) or even 60 min (ibandronate) after taking oral bisphosphonates [10]. This, of course, does not apply to intravenous bisphosphonates (ibandronate, pamindronate, zoledronate), whose administration is not similarly restricted. Approximately 40–60% of the absorbed dose is concentrated in the skeleton, depending on the rate of bone turnover and the type of bisphosphonate, and the remaining amount is excreted, unaltered, in the urine [11]. The plasma half-life of bisphosphonates is short; given intravenously or orally, all bisphosphonates are eliminated quickly from the circulation, within 6 h of administration [12]. However, bisphosphonates stay embedded in bone for a very long time; for example, the terminal half-life of alendronate in humans has been estimated to be about 10 years [13]. Bisphosphonates may bind to plasma proteins, and some of them (especially N-BPs) are eliminated by a renal tubular secretory mechanism [11].
The suppressive effect of bisphosphonates on bone resorption is delayed by at least 1–2 days, in contrast to the more rapid effect of calcitonin. Both the concentration of bisphosphonate present in bone mineral at any time and the total dose administered over a long period of time seem to be important for the magnitude of the reduction in bone turnover [14, 15].
Drug interactions are limited to aminoglycoside antibiotics, in which case severe hypocalcemia can occur [16]. Calcium and magnesium salts impair the intestinal absorption of oral bisphosphonates.
2.5 Adverse Effects
Bisphosponates are generally well tolerated, but side effects have been described. Oral bisphosphonates (alendronate, risedronate, and ibandronate), mainly used for the treatment of osteoporosis, have been associated with adverse events involving the upper gastrointestinal (GI) tract, such as nausea, vomiting, epigastric pain, and dyspepsia, due to mucosal irritation of the upper GI tract. In fact, several cases of esophagitis with esophageal erosions or ulcerations associated with the use of alendronate were reported through post-marketing surveillance of the drug [17]. Accordingly, Barrett’s esophagus should be a contraindication for bisphosphonates. Other adverse events are hypocalcaemia and secondary hyperparathyroidism, musculoskeletal pain, osteonecrosis of the jaw, atypical fractures of the femoral diaphysis, acute phase response (APR), and ocular events. The most common ocular side effect of bisposhonate is non-specific conjunctivitis [18], which usually improves without specific therapy and despite continuing treatment with bisphosphonates. However, the most serious ocular side effects are uveitis and scleritis, both of which require the discontinuation of bisphosphonate treatment [19].
Compounds in the group N-BPs are potent inhibitors of osteoclastic bone resorption. Six weeks after alendronate therapy is started, serum calcium and phosphorus decrease [20] and parathyroid hormone (PTH) significantly increases in a dose-dependent fashion. The increased PTH antagonizes the effect of bisphosphonates in bone and conserves calcium by increasing its tubular reabsorption in the kidneys and by stimulating the kidneys to produce 1,25-dihydroxyvitamin D.
Early clinical studies of bisphosphonates demonstrated strong anti-fracture efficacy for these agents. However, many investigators expressed concern that the anti-fracture benefit would be limited, because such striking reductions in bone remodeling might interfere with the microdamage repair process. This sequence of events did not seem to occur within the time frame of clinical anti-fracture trials, which lasted a minimum of 3 years and produced no evidence of resurgent fractures (femoral diaphysis) [21]. As reported by some authors, femoral-shaft fractures are rare among bisphosphonate users, suggesting that the osteoclasts of patients suffering from these fractures are genetically susceptible to over-suppression by bisphosphonates [22].
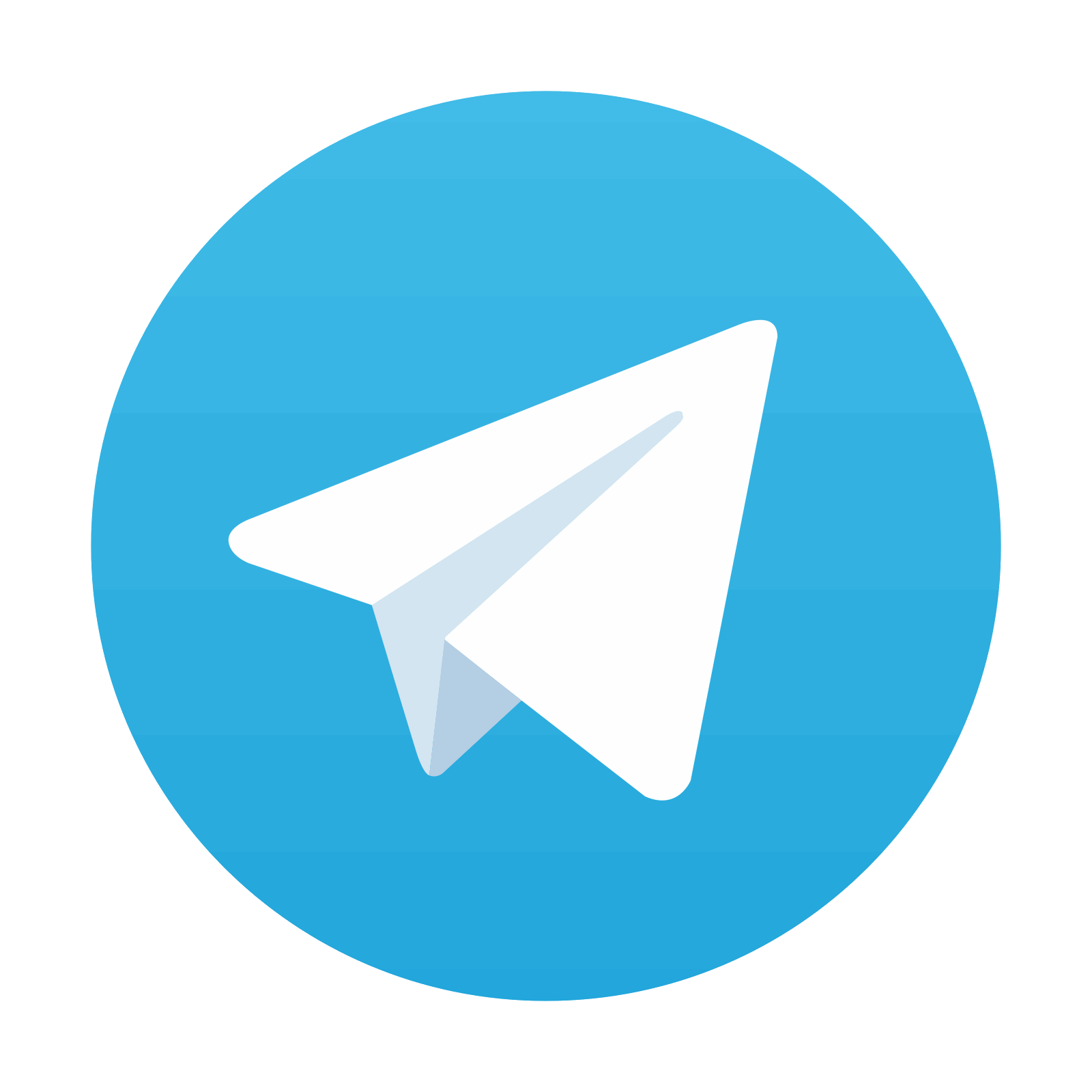
Stay updated, free dental videos. Join our Telegram channel

VIDEdental - Online dental courses
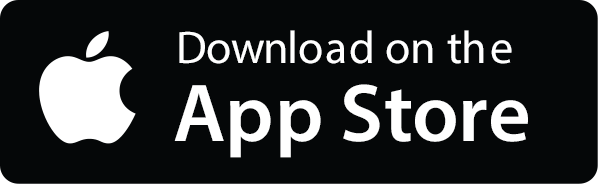
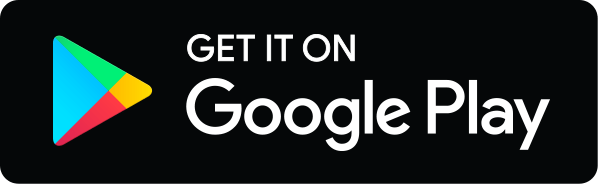