© Springer International Publishing Switzerland 2016
Jorge Perdigão (ed.)Tooth Whitening10.1007/978-3-319-38849-6_3
3. Overall Safety of Peroxides
(1)
Center for Dental Research, Loma Linda University School of Dentistry, 11175 Campus Street, Loma Linda, CA 92350, USA
(2)
Microbiology and Molecular Genetics, Loma Linda University School of Dentistry, Loma Linda, CA, USA
Abstract
Current tooth whiteners contain peroxides as active ingredients, which release hydrogen peroxide (H2O2) in the process of application. The primary source of safety concerns with the peroxide-based tooth whiteners is the capability of H2O2 to produce oxidative free radicals or reactive oxygen species (ROS), which have been associated with various pathological consequences including carcinogenesis and degenerative diseases. This chapter will review and discuss toxicology of H2O2, its presence in the human body, and its potential systemic effects, genotoxicity, and carcinogenicity on the basis of evidence available in the literature.
3.1 Background
Safety concerns with peroxide-based tooth whiteners are primarily originated from their content of peroxide compounds (Li 1996, 1997, 2011; Li and Greewall 2013). Carbamide peroxide (CH6N2O3) and hydrogen peroxide (H2O2) are the most commonly used peroxide compounds as the active ingredient in current extracoronal tooth-whitening products, while sodium perborate (NaBO3) is primary for intracoronal bleaching procedures (Rotstein and Li 2008). Carbamide peroxide, or urea hydrogen peroxide, is a white crystal or a crystallized powder. Chemically, carbamide peroxide is composed of approximately 3.5 parts of H2O2 and 6.5 parts of urea; a tooth whitener of 10 % carbamide peroxide thus contains approximately 3.5 % H2O2. Sodium perborate is also a white powder available either as monohydrate, trihydrate, or tetrahydrate. The monohydrate and tetrahydrate forms are commonly used for intracoronal bleaching, with H2O2 content theoretically around 34 % and 22 %, respectively. In an aqueous medium, both carbamide peroxide and sodium perborate decompose to release H2O2, which, therefore, is the true active ingredient of the peroxide-based tooth-whitening products.
3.2 Toxicology of Hydrogen Peroxide
H2O2 as a chemical was first identified in 1818, and the well-known Fenton reaction was proposed in 1894. Two enzymes, peroxidase and catalase, found in 1898 and 1901, respectively, were quickly recognized to play important roles in H2O2 metabolism in humans. Shortly after the discovery of another important enzyme, superoxide dismutase (SOD), in 1969, research efforts on biological properties of H2O2 have significantly increased (Li 1996).
The toxicology of H2O2 has been investigated extensively, and there are a number of comprehensive reviews on the topic available in the literature (IARC 1985; ECETOX 1993; Li 1996; SCCP 2005; CEU 2011). A key characteristic of H2O2 is its capability of producing reactive oxygen species (ROS), which are known to induce various toxicities, including hydroxyl free radicals that have been implicated in various stages of carcinogenesis (Floyd 1990; Li 1996). Oxidative reactions of ROS with proteins, lipids, and nucleic acids are believed to be involved in a number of potential pathological consequences; the damage by oxidative free radicals may be associated with aging, stroke, and other degenerative diseases (Harman 1981; Floyd et al. 1988; Lutz 1990; Li 1996).
The major mechanism responsible for the observed toxicity of H2O2 is believed to be the oxidative reactions and subsequent damage in cells by ROS. In cell culture studies, H2O2 is highly cytotoxic at concentrations ranging from 1.7 to 19.7 μg/mL or 0.05 to 0.58 mmol/L (Rubin and Farber 1984; Bates et al. 1985; Ramp et al. 1987; Tse et al. 1991; Hanks et al. 1993; Li 1996, 2003). Hepatocytes were less sensitive to the cytotoxicity of H2O2 than fibroblasts and endothelial cells (Sacks et al. 1978; Simon et al. 1981; Rubin and Farber 1984), while human gingival fibroblasts derived from primary cultures and L929 mouse fibroblasts (ATCC CCL 1; Manassas, VA) were found to respond similarly to the cytotoxicity of H2O2 (Li 1996).
On the other hand, the human body is equipped with various defensive mechanisms available at cellular and tissue levels to prevent potential damage of H2O2 to cells during oxidative reactions and to repair any damages sustained. A number of enzymes, such as catalase, SOD, peroxidase, and selenium-dependent glutathione peroxidase, exist widely in body fluids, tissues, and organs, to effectively metabolize H2O2 (Floyd 1990; Li 1996). Simply adding iron chelators and antioxidants or increasing serum concentration in culture media effectively reduces or eliminates the cytotoxicity of H2O2 (Sacks et al. 1978; Rubin and Farber 1984). In a cell culture study, 20 mM H2O2 was undetectable after 30 min in the culture media alone and after 15 min in the media with bone tissues, indicating decomposition and inactivation of hydrogen peroxide in cell culture systems (Ramp et al. 1987). These enzymes also exist in human saliva; in fact, salivary peroxidase has been suggested to be the body’s most important and effective defense against the potential adverse effects of H2O2 (Carlsson 1987). Marshall and coworkers (2001) found that the human oral cavity, including that of adults, juveniles, infants, and adults with impaired salivary flow, was capable of eliminating 30 mg H2O2 in less than one and a half minutes.
3.3 Peroxides in the Human Body
The detection of H2O2 in human respiration was first reported in 1880; however, it was not until 1969 when SOD was discovered H2O2 was recognized as an important by-product in oxygen metabolism of humans (Li 1996, 2011). H2O2 is now known as a normal intermediate metabolite in humans. It exists in human serum, and it is present in human breath at levels ranging from 0.34 to 1.0 μg/L (Sies 1981; Williams et al. 1982). The daily production of H2O2 in human liver is approximately 6.48 g in a period of 24 h (FDA 1983). An important source of endogenous H2O2 is from phagocytic cells, such as neutrophils and macrophages, which play an essential role in defense against various pathological microorganisms.
3.4 Systemic Effects
Systemic effects of H2O2 have been investigated for both acute and chronic exposures. A unique characteristic of H2O2 in inducing systemic toxicity is its concentration in addition to the dosage.
The reported acute systemic toxic effects of H2O2 in animals vary widely, according to the H2O2 concentration as well as the application mode. In rats, the intravenous 50 % lethal dose (LD50) of H2O2 was found to be 21 mg/kg (Spector 1956). Using the up-and-down method, in which the dosing is adjusted up or down according to the outcome (death or survival) of the animal that received the previous dosage, the oral LD50 of 4 % H2O2 solution in male and female rats was estimated at 780 and 600 mg/kg, respectively (Li 1996). The LD50 for percutaneous application of H2O2 is much higher at >7,500 mg/kg (FDA 1983). The values of LD50 are inversely related to the concentrations of H2O2, and they vary markedly between different animal species and strains (IARC 1985; FDA 1983; ECETOX 1993; Li 1996). Tissue responses to topical application of H2O2 are also related to the H2O2 concentration, but they are usually minimal at low concentrations of ≤3 %.
Acute toxicity, including fatalities, has been reported in humans who accidentally ingested large amounts of concentrated H2O2 solutions (Spector 1956; Giusti 1973; Giberson et al. 1989; Humberston et al. 1990; Rackoff and Merton 1990; Christensen et al. 1992; Cina et al. 1994; Sherman et al. 1994; Asanza et al. 1995; Ijichi et al. 1997; Rider et al. 2008; Byrne et al. 2014). A retrospective survey of a regional poison control center found that over a 36-month period, 325 cases were caused by H2O2 poisoning, which accounted for 0.34 % of all the reported causes (Dickson and Caravati 1994); however, the majority of the 325 cases (71 %) was pediatric population (age <18 years), with ingestion of H2O2 solution being the most common route of exposure (83 % of cases). One major factor associated with the toxicity of H2O2 is its concentration. Ingestion of H2O2 solutions of less than 10 % usually produces no significant adverse effects, although it may cause mild irritation to mucous membranes, which results in spontaneous emesis or mild abdominal bloating (Humberston et al. 1990; Dickson and Caravati 1994). Exposure to H2O2 concentrations higher than 10 %, however, can result in severe tissue burns and significant systemic toxicity. In addition to the tissue damage caused by oxidative reactions, gas embolism is responsible for various pathological consequences of H2O2 ingestion (Rackoff and Merton 1990). Each milliliter of 1 % H2O2 releases 3.3 mL oxygen; therefore, 10 mL of 30 % H2O2 can produce 1 liter oxygen (Giberson et al. 1989; Humberston et al. 1990). Common symptoms observed in acute toxicity of H2O2 include stomach and chest pain, retention of breath, foaming at the mouth, loss of consciousness, motor and sensory disorders, fever, gastric hemorrhage, and liver damage. Although rare, death can occur.
Several animal studies have been conducted on acute systemic toxicity of tooth whiteners containing carbamide peroxide. Oral gavage of 5 g/kg tooth whiteners containing 10 and 22 % carbamide peroxide produced no evidence of acute systemic toxicity in rats (Cherry et al. 1993; Adam-Rodwell et al. 1994). One study reported unusually low LD50 (87.18–143.83 mg/kg) of two products containing 10 % carbamide peroxide in female Swiss mice (Woolverton et al. 1993). The reasons for the low LD50 values are unclear but may be attributed to differences in animal species, materials, and method. Using the up-and-down method, the LD50 of a tooth-whitening gel with 10 % carbamide peroxide was estimated at 23.02 g/kg in female rats (Li et al. 1996).
Chronic systemic toxicity of H2O2 has been investigated using animal models. No visible abnormalities were detected in mice drinking 0.15 % H2O2 (about 150 mg/kg/day) ad libitum for 35 weeks, and their growth was also normal (FDA 1983). Necropsy results, however, showed changes in the liver, kidney, stomach, and small intestine. Solutions of >1 % H2O2 (>1 g/kg/day) caused pronounced weight loss and death of mice within two weeks. A rat study by Ito et al. (1976) found that when administered by an oral gastric catheter 6 days weekly for 90 days, the dose of 506 mg/kg suppressed body weight gain, decreased food consumption, and caused changes in hematology, blood chemistry, and organ weights. The principal tissue affected was gastric mucosa, and the effects were local. The no-observed-effect level (NOEL) of H2O2 was 56.2 mg/kg/day. Another rat study found that the NOEL of H2O2 was 30 mg/kg/day when animals were treated by oral gastric catheter daily for 100 days (Kawasaki et al. 1969). The same study showed no adverse effects in rats receiving the diet containing 6 mg H2O2 in 20 g of food.
3.5 Genotoxicity
The genotoxic potential of H2O2 has been investigated extensively using microbes, plants, insects, cultured mammalian cells, and animals (IARC 1985; ECETOX 1993; Li 1996; SCCP 2005). In a number of bacterial systems, H2O2 induced point mutations or single-strand breaks in DNA. Positive mutagenicity of H2O2 has also been detected in some newer tester strains of the Ames Salmonella mutagenicity test; however, effects are eliminated when tested with S9 activation. S9 is a rat liver microsomal preparation that contains various enzymes. It has been found to increase the sensitivity and overall performance of the Ames Salmonella mutagenicity test, and therefore, experiments both with and without S9 are required for the Ames Salmonella mutagenicity test (Maron and Ames 1983). The results obtained from mammalian cells are similar to those from the Ames Salmonella mutagenicity test; that is, the genotoxic effects of H2O2 are detected only in test systems without S9 activation. The effect of S9 on H2O2-induced DNA or chromosomal changes in mammalian cells in vitro is believed to originate from the H2O2-degrading enzymes in the S9, which is the same as that observed in the Ames Salmonella mutagenicity test.
The genotoxicity of H2O2 has also been examined using in vivo systems, and results indicate that H2O2 is not genotoxic in various animal models (IARC 1985; ECETOX 1993; Li 1996; SCCP 2005). The overall data available so far show that H2O2 is genotoxic only in in vitro systems without enzymatic activation. When enzymatic activation is incorporated into in vitro systems or when tested in animals, H2O2 is nongenotoxic.
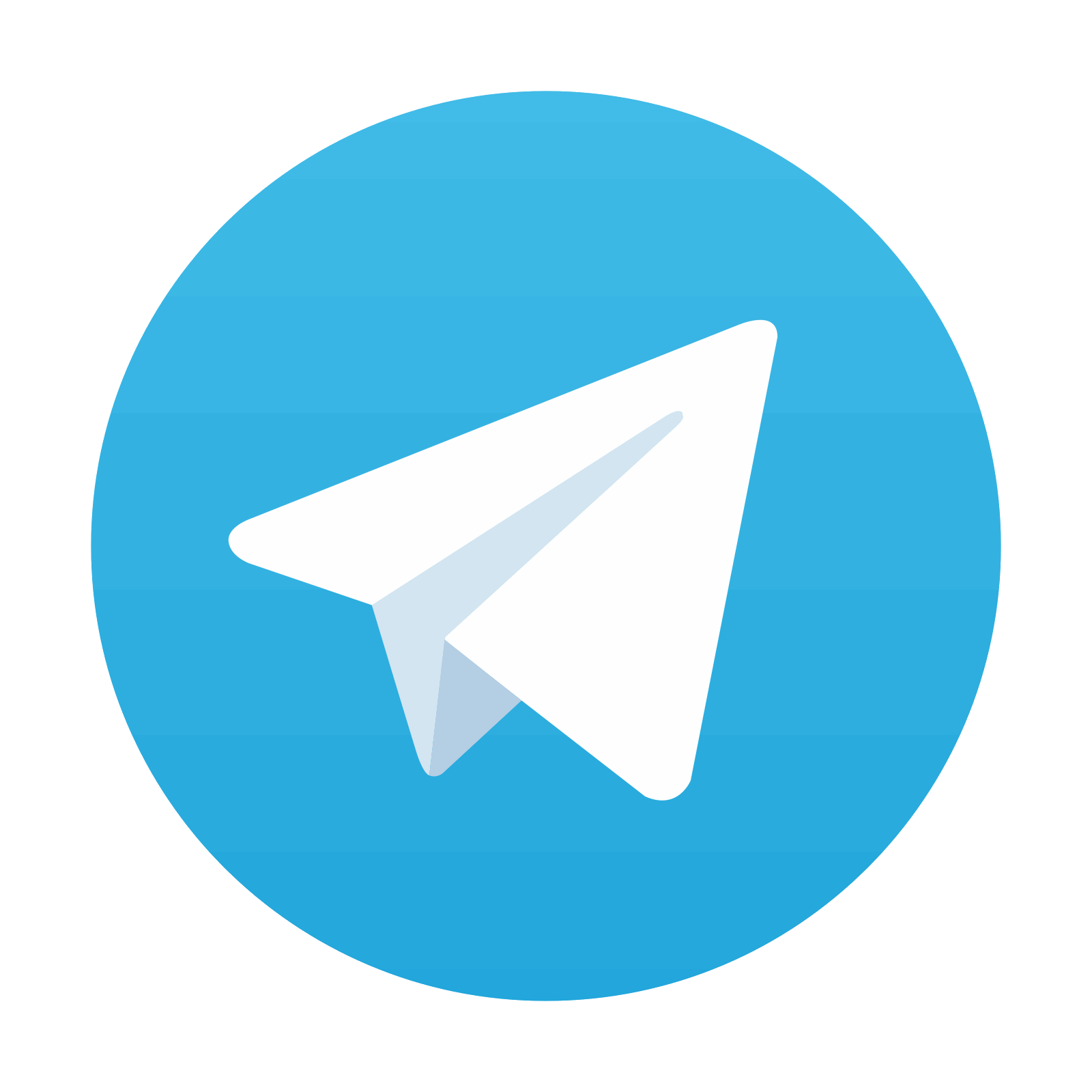
Stay updated, free dental videos. Join our Telegram channel

VIDEdental - Online dental courses
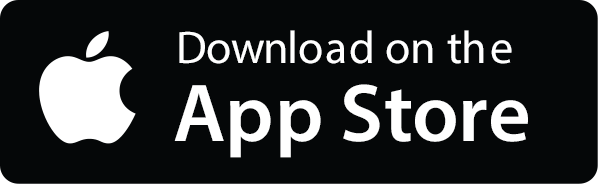
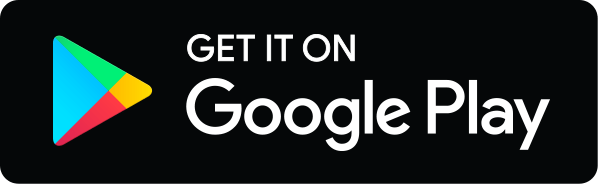