Introduction
The aims of this retrospective study were to use cone-beam computed tomography (CBCT) to assess changes in the volume of the oropharynx in growing patients with maxillary constriction treated by rapid palatal expansion (RPE) and to compare them with changes in age- and sex-matched orthodontic patients.
Methods
The experimental group consisted of 24 patients (mean age, 12.8±1.88 years) with maxillary constriction who were treated with hyrax palatal expanders; the control group comprised 24 age- and sex-matched patients (mean age, 12.8±1.85 years) who were just starting regular orthodontic treatment. Beginning and progress CBCT scans, taken in the supine position, were analyzed with software to measure volume, length, and minimal cross-sectional area of the oropharyngeal airway. The 2 groups were compared with paired t tests.
Results
Only retropalatal airway volume was found to be significantly different between groups before treatment ( P = 0.011), and this difference remained after treatment ( P = 0.024). No other statistically significant differences were found relative to changes in volume, length, or minimum cross-sectional area of the oropharyngeal airway between the groups, but the molar-to-molar width after RPE increased significantly compared with the controls ( P <0.001).
Conclusions
Narrow oropharyngeal airways in growing patients with maxillary constriction was demonstrated. But there was no evidence to support the hypothesis that RPE could enlarge oropharyngeal airway volume.
Transverse maxillary deficiency is associated with many problems that include esthetic issues, dental crossbites, occlusal disharmony, and other functional problems. One functional problem is a narrow oropharyngeal airway resulting from the retroposition of the tongue. Prior studies suggest that maxillary constriction can also play a role in the pathophysiology of obstructive sleep apnea (OSA). Johal and Conaghan concluded that maxillary morphologic differences exist between OSA and control subjects, supporting their role as an etiologic factor. Rapid palatal expansion (RPE) as a treatment modality for OSA has been proposed based on the hypothesis that airway volume increases after maxillary expansion secondary to the tongue’s repositioning more anteriorly in the oral cavity.
RPE was first described in the 1860s by Emerson Angell, who used a jackscrew with contrarotating heads. Since then, RPE has become a common treatment for transverse maxillary deficiency, and its effects have been well described in the literature. Studies have documented the amount of maxillary width increase, nasal resistance reduction, and intranasal capacity increase with RPE treatment. In this regard, Pirelli et al recruited 31 children with OSA, followed them for up to 4months after RPE treatment, and found that all had a decreased apnea-hypoapnea index with a mean maxilla cross-sectional expansion of 4.32 ± 0.7 mm. The specific changes in oropharyngeal airway volume and length are not as clear.
Two-dimensional imaging techniques (lateral and anteroposterior cephalometric images) and 3-dimensional (3D) imaging techniques (magnetic resonance imaging, computed tomography [CT], and cone-beam CT [CBCT]) have been used to evaluate changes in airway volume. Techniques that have been used to compare the change of airway space after RPE treatment include cephalometric analysis and acoustic rhinometric measurement, which is used to evaluate the change of nasal airway volume and nasal airway resistance. However, acoustic rhinometric measurements cannot correctly detect constrictions and expansions less than 3 to 4 mm. Lateral and anteroposterior cephalometric evaluations have been used to compare the dimensional changes in the maxilla and upper airway, but the complex shape change of the airway after RPE treatment is not represented well with 2-dimensional images. Compared with magnetic resonance imaging and traditional CT techniques, CBCT is a better method for airway volume measurement because of its lower cost, easier access, availability to dentists, and much lower overall effective absorbed dose of radiation than CT. The CBCT (NewTom 3G, QA S.R.L., Verona, Italy) imaging system used in this study has an overall effective absorbed dose of radiation of 57 μSv and scanning time of 36 seconds. This compares favorably with traditional dental images such as panoramic radiographs with radiation doses of 6 μSv and a full-mouth series with a range of 33 to 84 μSv to 14 to 100 μSv, depending on variables such as film speed, technique, kilovolts (peak), and collimation.
Therefore, the purpose of this study was to evaluate the changes of oropharyngeal airway volume after RPE treatment in adolescent patients compared with age- and sex-matched controls by using 3D images obtained by CBCT. The null hypothesis was that there is no difference in the mean changes in volumetric oropharyngeal airway (retropalatal or retroglossal) between pairs of patients with RPE and matched controls treated with orthodontics only. We also evaluated differences in changes in the minimum cross-sectional airway, orpharyngeal airway length (retropalatal and retroglossal), and molar-to-molar width between the matched pairs.
Material and methods
In this retrospective study, 24 healthy patients (mean age, 12.8 ± 1.88 years; range, 8.9–15.1 years; 6 boys, 18 girls) who fulfilled the following inclusion criteria were selected: (1) required RPE treatment because of a bilateral or unilateral crossbite, (2) required orthodontic treatment, and (3) had beginning and progress treatment CBCT scans. All patients who fulfilled these criteria were included in the study. Twenty-four controls who had regular orthodontic treatment only (no RPE) and had CBCT beginning and progress scans were selected to match the RPE patients in age (age difference between controls and subjects, 3.0 ± 2.54 months) and sex. The control group’s mean age was 12.8 ± 1.85 years (range, 8.6–15.8 years; 6 boys, 18 girls). All patients were in the late transitional or early permanent dentition stage. No patients were planned for extraction or orthognathic treatment. This study was approved by the institutional review board of the University of Southern California.
A review of the treatment records showed that the patients in the RPE group had a hyrax-type palatal expander banded on the maxillary first premolars and first molars. The patients were monitored weekly for appropriate activation of the appliance. Expanders were turned 1 or 2 times per day until the required expansion was achieved—ie, slight overcorrection of the crossbite (average time, 4–6 weeks)—and then were stabilized. A rapid palatal expander or a transpalatal arch was used for retention for at least 3months postexpansion. Most patients with RPE had no orthodontic treatment until after the fixed retention period, but some patients had appliances placed (eg, bonded brackets on maxillary incisors) during the fixed retention period. The control patients started orthodontic treatment within 6months of the experimental group. CBCT scans were taken of all patients as part of both initial orthodontic treatment records and progress records (midpoint of the total treatment time, from 8months to 2 years; average, 15months).
The patients were scanned in supine position with the Frankfort plane perpendicular to the floor in centric occlusion. The digital imaging and communications in medicine (DICOM) data were imported into the imaging software Vwork (version 5.0, Cybermed USA, Torrance, Calif) and used for the measurements described below. All measurements were made by a blinded operator (Y.Z.).
Standardization of head position between scans was achieved by measuring the angle between the line from anterior nasal spine to posterior nasal spine, and thevertical line predefined by the software on the beginning scan and matching this tothe progress scan (by reslicing the volume accordingto thebeginning head angulation).
To define the oropharynx volume area of interest, an approximate square-shaped prism was defined first to outline the general area, and then, based on that outline, an anatomically well-defined hand-traced volume was created. To orient the prism angulation, the patient’s midsagittal plane was defined as the anterior midpoint of the 2 maxillary incisors and the posterior midpoint of the spine ( Fig 1 ). The total oropharyngeal airway volume was defined as the airway volume between the 2 planes as follows: the superior plane was defined on the midsagittal image as the horizontal line through the posterior nasal spine, and the inferior plane was defined as the horizontal line through the superior point of the epiglottis ( Fig 2, A ). To define the anteroposterior and lateral borders of the volume area of interest, the first step was to define and select a square area containing the entire airway on an axial view. All axial views were checked to ensure that the airway was included in the selected area. Subsequently, the upper and lower borders in the airway volume area of interest were determined, and the specific borders of the segmented airway were defined manually by tracing the soft tissue-air interface at each 1-mm axial slice with the segmentation tool. Once segmentation was performed, the software automatically computed the volume of the oropharyngeal region in cubic millimeters (DICOM header contains the size of the voxel in millimeters plus the thickness of the slices). Last, the oropharyngeal airway volume was divided into retropalatal and retroglossal airway volumes by creating a horizontal plane through the inferior point of the uvula ( Fig 2, B and C ).
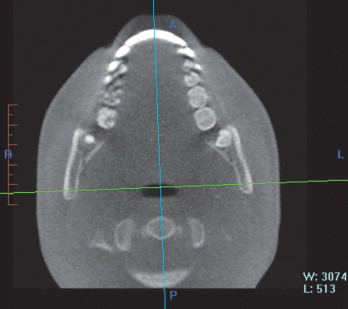
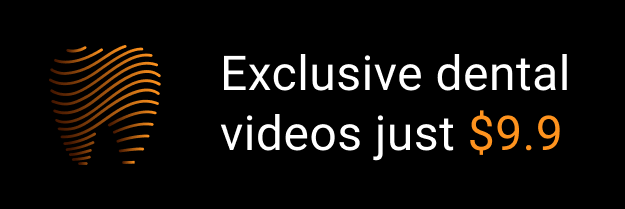