Fig. 8.1
Scanning electron micrographs of microhybrid composites Filtek Z350 (a–c) and TPH Spectrum (d–f); nano-based Ketac N100 (g–i), and resin-modified GIC Vitremer (j–l). The first column shows the relative humidity storage groups (a, d, g, j), with porosities (small spherical and irregular shapes) indicated by arrows. The second column represents the S mutans biofilm storage groups (b, e, h, k), with a severe degraded aspect of the matrix. The third column corresponds to biofilm storage plus abrasion groups (c, f, i, l), with many exposed particles at the surface of materials (squares) (Reprinted from De Paula et al. [43]. With permission from Operative Dentistry, Inc.)
The fluoride release of Ketac Nano (3M) has also been compared to other commercially available GICs for a period of 360 days. The amount and pattern of fluoride release rate of nano-ionomer is similar to that of other resin-modified (Fuji II LC, GC and Vitremer, 3M) [20] and conventional (Fuji II, GC) GICs [46], with a burst effect of fluoride release at shorter times. The application of a primer as surface protection does not affect the fluoride release rate [20]. Although smaller glass particles have a larger surface area, which would theoretically increase the acid-based reactivity and release fluoride from the powder more effectively, that effect has not been clearly demonstrated. In spite of the smaller particle size, the filler content on Ketac Nano (69 %) is lower than that on Fuji II LC (76 %) and Vitremer (71 %). Therefore, mechanical properties as well as fluoride uptake/release characteristics are somewhat lower than those presented by other resin-modified GICs [47].
The clinical performance of Ketac Nano (3M) has been assessed and compared to a resin-modified GIC (Fuji II LC, GC America) and a nanofilled restorative composite (Filtek Supreme, 3M). Non-carious cervical lesions were restored and evaluated after 6 and 12 months. For Ketac Nano, marginal staining after 1 year of clinical service was significantly worse than baseline and also worse than Fuji II LC within the same period of time. Marginal adaptation and colour match of nano-based GIC were also significantly inferior than its baseline and other materials [48]. Indeed, the micromechanical interaction between Ketac Nano and dentine/enamel is very superficial, without structural evidence of demineralization or hybridization since the monomer and photoinitiator of the primer form a resin coating that avoids deeper infiltration of GIC [49].
Another application of nanotechnology in glass ionomer cements is the development of a nano-filled light curing varnish (G-Coat Plus, GC Europe), which is applied onto the surface of a highly viscous GIC (Fuji IX GP Extra, GC Europe). This combination has been commercially branded as EQUIA (‘Easy-Quick-Unique-Intelligent-Aesthetic’). The main purpose is to provide surface protection in the early maturation phase of the cement to avoid both water uptake and dehydration [50]. The longer the protective varnish is in contact with the restoration, the smaller the chance that GIC will have its mechanical properties reduced [51]. A retrospective cohort study evaluated anatomical form, surface texture, marginal integrity and marginal discoloration of EQUIA restorations in class I and class II preparations in either molars or premolars. A significant correlation between cavity size and volume loss of material was observed. Chipping and volume loss were mainly observed in the proximal surface, where varnish could not be applied. Wear of the occlusal surface was not significant during the 2 year observation period. Although the authors concluded that the application of EQUIA restorations was considered acceptable for class I and small class II preparations [50], 2 years is a relatively short period of evaluation, and results should be examined with care.
The influence of the nano-filled surface varnish (G-Coat Plus, GC) on wear resistance and flexural strength has also been investigated in coated and uncoated conventional GICs (Fuji IX GP Extra, GC; Ketac Molar, 3M) [52]. Flexural strength of Fuji IX is significantly improved by the application of the nano-filled varnish, but it is still lower than the flexural strength of Ketac Molar, either coated or uncoated. There is no chemical interaction between Ketac Molar and the varnish layer, forming a deposited layer that is easily removed by the wear test. Although the nano-filled varnish coating may be considered very promising to extend longevity of GIC restorations, decrease in wear rate as a function of time was observed for all groups, with or without coat [53]. In spite of being called ‘nano varnish’, there is no information available about particle size distribution neither in any of the research papers investigated nor in the manufacturer’s brochure, which makes it difficult to correlate the performance of the material with the presence of nanofillers.
Some authors observe that GIC is still a very interesting option in public health systems, especially considering the economic aspects. Besides being an aesthetic and safe alternative for those patients who refuse the placement of an amalgam restoration, the bulk placement of the material reduces clinical time and, consequently, the cost of the procedure. Additionally, fluoride release is a very unique characteristic of this class of material. Therefore, there is still demand for the improvement of glass ionomer cements in an attempt to develop a ‘permanent’ restorative material, instead of the current status of the GICs as being semi-permanent restorations.
8.3 Application of Nanoparticles in Resin-Based Materials
By definition, composite materials (also called resin composites or shortened to composites) are materials made from two or more constituent materials with significantly different physical or chemical properties that, when combined, produce a material with characteristics different from the individual components. The current chemistry of restorative composites involves a Bis-GMA organic matrix combined with some other monomers (TEGDMA, HEMA, UDMA – urethane dimethacrylate and others) [54] and inorganic–organic fillers of different sizes [55]. The treatment of the fillers’ surface with 3-methacryloxypropyl trimethoxy silane (MPTMS) allows the formation of covalent links between the inorganic filler particles and the organic resin matrix [55]. Typically, nano-based restorative composites contain 13–30 wt% of polymerizable organic matrix and 70–87 wt% mixture of different inorganic fillers [54] in addition to a photoinitiator system or other curing systems [56].
The types of nanofillers in dental composites include silica [57, 58], tantalum thoxide [59], zirconia-silica [3], alumina [60], nano-fibrillar silicate [61] and titanium oxide [62], among others. Those nanoparticles may be used as the sole filler of the adhesive [63, 64] or in combination with other types of fillers for composites [65]. However, the wide spectrum of fillers and dimensions has made it difficult to classify different materials within the same category. Resin composites with particles smaller than 100 nm have been conveniently named nanocomposites [66, 67]. Nonetheless, this classification is controversial, and some authors name the same material nanonybrids [68] based on the association of nanoparticles, nano-filled clusters and microparticles. Neither information provided by the manufacturers nor research articles have been successful in reporting the precise amount of nanofillers in the final composition of resin composites, informing only the overall amount of fillers (Table 8.1). Considering the composition of the materials containing nanoparticles that are currently available, if the filler loading is composed only of particles smaller than 100 nm, the resin composite shall be considered nanofill or nanocomposite. Restorative composites containing filler particles smaller than 100 nm associated with microfillers (particles larger than 0.1 μm) are hybrid materials and may be precisely considered nanohybrids.
Table 8.1
Materials currently available making use of nanotechnology
Category
|
Brand name
|
Classification
|
Particles
|
Characteristics (reference #)
|
---|---|---|---|---|
Glass ionomer materials
|
Ketac Nano
3M Espe
St Paul, MN, US
|
Nano-GIC
|
1 μm clusters containing 5–25 nm spherical particles [41]
|
Good resistance to biomechanical degradation [44]
Fluoride release similar to other GIC’s [46]
Marginal staining under clinical conditions [48]
|
G–Coat Plus
GC America
Alsip, IL, USA
|
Nano-filled light curing varnish
|
No information available
|
||
Enamel/dentin bonding
|
Prime&Bond NT
Dentsply International
York, PA, USA
|
Nano-filled adhesive
|
7–12 nm SiO2 particles
|
Higher microtensile bond strength to dentin than one-bottle self-etch adhesive systems [69]
Low microtensile bond strength to feldspathic porcelain [70]
|
Composite resin
|
Filtek DEB
3M Espe
St Paul, MN, USA
|
Nanofill
|
Zirconia-silica nano-filled clusters (0.6–1.4 μm; 90 %) and nanoparticles (5–20 nm; 10 %) dispersed in the matrix
79 wt%
|
Fracture strength lower than for ormocer-based composite [66]
Higher polymerization shrinkage, water sorption and solubility [65]
Decrease in flexural strength and flexural modulus after 30 days water storage [65]
Decreased Knoop hardness after 6 months water storage [74]
Color instability after additional heat post-curing [75]
Good resistance to biomechanical degradation [43]
|
Filtek translucent
3M Espe
|
Nanofill
|
Nanoparticles (~75 nm) and minor amount of silica nano-filled clusters (0.6–1.4 μm; 50 %)
70 wt%
|
High polymerization shrinkage, water sorption and solubility [65]
Decreases flexural strength and flexural modulus after 30 days water storage [65]
|
|
Tetric EvoCeram
Dentsply
|
Nanohybrid
|
Barium glass (1 μm), Ba-aluminum-silicate glass (0.4–0.7 μm) and Ytterbium trifluoride (550 nm)
82–83 wt%
|
Fracture strength lower than for ormocer-based composite [67]
Low roughness values [67]
Reduced polishability after cyclic loading [73]
|
|
Grandio/Grandio Nano
Voco
Briarcliff Manor, NY, USA
|
Nanohybrid
|
Silica dioxide (20–60 nm) and barium-aluminaborosilicate
(0.1–2.5 μm)
87 wt%
|
Minimum polymerization shrinkage when compared to nanofill and Tetric EvoCeram, lower water sorption and solubility, high and stable flexural strength and flexural modulus after 30 days water storage [65]
Higher degree of conversion and lower color stability than microhybrid materials [76]
|
|
Clearfil Majesty
Dentsply
|
Nanohybrid
|
Silanated Barium glass filler and pre-polymerized organic filler including nanoparticles (0.2–100 μm) with average particle size of 0.7 μm
78 wt%
|
Color instability after additional heat post-curing [75]
|
|
Ceramic
|
NanoZr
Matsushita Electric Works, Tokyo, Japan
|
Nanocomposite
|
ZrO2, 67.9 mass%; Al2O3, 21.5 mass%; CeO2, 10.6 mass%; MgO, 0.06 mass%; TiO2, 0.03 mass%
|
High flexural strength after airborne-particle abrasion and acid etch [77]
Good shear bond strength to veneer material, without the need of a liner [78]
|
The key challenges in the development of dental nanocomposites include (1) effective dispersion of nanoparticles in the resin to avoid agglomeration and (2) achieving high loading of nanofiller level to reduce polymerization shrinkage while maintaining good handling characteristics and manufacturing costs [79]. It is also paramount to understand the effect of nanofiller size, morphology, composition and filler hybridization on composite properties as well as the long-term durability of those restorations in vivo [79].
The adhesion between a resin-based restorative material and tooth structure is required to avoid microleakage, secondary caries, post-operative sensitivity and discoloration [80]. The quality of the bonding between tooth structure and restoration relies on the impregnation of the fluid resin into a superficially decalcified zone of enamel rods and dentine collagen fibrils [81] to form the hybrid layer [82]. Due to the humidity and dynamic characteristics of dentine substrate, adhesion to dentine is more complex than to enamel [83]. A high-quality and durable hybrid layer can be only achieved if the demineralized dentine collagen matrix is fully resin infiltrated [84]. When collagen is not fully enveloped by the adhesive or porosity is present in the hybrid layer, micro- and nano-pathways can expedite the process of interfacial degradation [85, 86], reducing the resin–dentine interface durability [87, 88].
There are currently two methods to create dentine bonding and promote the hybrid layer formation: etch-and-rinse and self-etch techniques. Regardless of the selected strategy, the mechanism available for adhesive resin infiltration is diffusion of the resin into whatever fluid is in the spaces of the substrate and along the collagen fibrils [89]. The elastic modulus of the hybrid layer formed at the resin–dentine interface is the lowest compared to dentine and resin composite [80]. This low elastic modulus can cause the failure of the restoration when the occlusal loading is higher than the strength of the layer [90]. The mechanical properties of the hybrid layer may be improved by the incorporation of fillers, which also increase viscosity and radiopacity [91]. However, filler aggregates resultant of nanoparticle clusters block the penetration of the nanoparticles into the collagen network, thus compromising the mechanical properties at the hybrid layer [92].
Different rationales justify the application of nanotechnology in adhesive systems: (1) to stimulate the remineralization of the demineralized/etched collagen below the hybrid layer [85]; (2) to improve mechanical properties, avoiding the early failure of the adhesive interface subjected to cyclic loading, and (3) to develop a bioactive resin-based adhesive capable of eliminating residual bacteria in the prepared tooth cavity as well as bacteria invading the tooth–restoration interface via microleakage [63].
Are the restorative resin composites resistant enough to endure a long-lasting clinical application? This is an important clinical question posed while optimizing the bonding technique. Till date, none of the composite resin materials has been shown to have the ability to meet both the functional requirements of a posterior Class I or II restoration and the superior aesthetics required for anterior restorations [3, 93]. The clinical requirements for a successful composite resin restoration are so many [55] that manufacturers can barely meet half of them and the average longevity of a composite resin restoration is about 4 years [94].
The inorganic fillers incorporated into resin composites directly affect the material’s radiopacity, wear resistance, flexural modulus and thermal coefficient of expansion [55, 95]. Considering that the polymerization shrinkage of the organic matrix is largely correlated with the volume fraction of fillers in the composite [56], the synergistic use of releasing nanofillers and reinforcing fillers may result in nanocomposites with the potential of having both stress-bearing and caries-inhibiting capabilities, a combination not available in current dental materials [79, 96].
8.3.1 Dentin Bonding Systems
As previously mentioned, are added to the composition of dentine bonding systems in an attempt to stabilize the hybrid layer by improving its mechanical properties [90, 97]. Although nano-filler particles are supposed to present a colloidal behaviour, by which each particle dispersed is surrounded by oppositely charged ions called the ‘fixer layer’, the filler content of the bonding agents frequently agglomerate into clusters [92]. Then, the demineralized dentine serves as a screener that prevents from resin penetration in the underneath dentine by collecting fillers on the top surface of the hybrid layer [98]. This outcome is somewhat controversial, as shown by Wagner et al. [15], who observed that nano-filled agglomerates were dispersed throughout the adhesive layer instead of being deposited on the top of the demineralized dentine layer (Fig. 8.2). The presence of additional solvent (water or ethanol) underneath the hybrid layer resultant from a self-etch technique may also generate filler aggregation. The effect of solvent on cluster formation in several commercial self-etch adhesive systems (three two-step and three all-in-one self-etching systems) modified with nanoparticles has been investigated. When adhesive resin is placed on wet dentine, the residual solvent existing within the dentine (either water or ethanol) will produce filler aggregation, with cluster size ranging from 19.6 to 103 nm [92], whilst interfibrilar collagen space on etched dentine surface is in the range of 12.1–19.8 nm when total etch technique is applied [99]. Even when smaller nanofillers are employed (average size 7–12 nm) in commercial (Prime&Bond NT, Dentsply) or experimental adhesives, there is some controversy as to whether the particles can penetrate into the restricted space of the hybrid layer or not [100]. Fluoralumino-silicate glass filler nanoparticles also present larger cluster size and more cluster frequency than silanated colloidal silica [92]. This is probably due to the thermal instability and other unknown factors of the nanoparticle–organosilane bond [101].
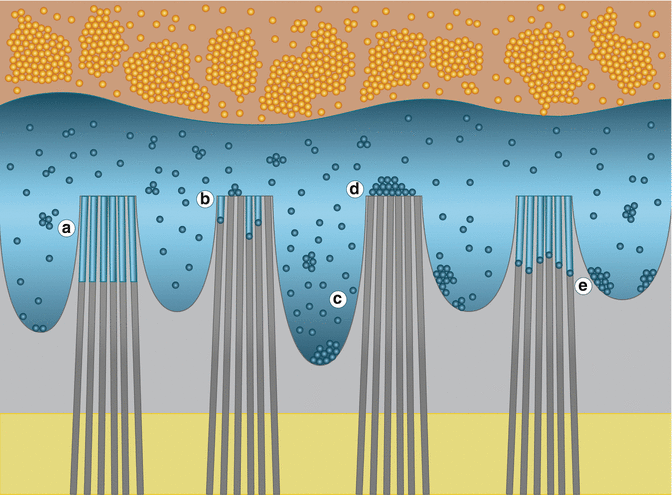
Fig. 8.2
Schematic representation of the dentin/adhesive hybrid layer (orange composite resin, blue adhesive resin, gray demineralized dentin, yellow intact dentin) with the effect of different nanoparticles application: (A). Wagner et al. 2013: Hydrothermally-grown HA with smaller surface area are embedded within the adhesive and not deposited on top of the hybrid layer. (B) Besinis et al. 2012: Hydrophobic diluent combined with smaller nHA improves infiltration ability of demineralized dentin. (C) Hoshika et al. 2010: Longer and denser resin tags obtained when CPN nanoparticles are added to the primer composition, by either improving monomer infiltration or enhancing monomer conversion. (D) Lohbauer et al. 2010: Spherical zirconia nanoparticles are unable to penetrate the interfibrilar spaces, compromising hybrid layer formation. (E) Osorio et al. 2011: Zinc oxide nanoparticles infiltrate the interfibrilar spaces and remain at the bottom of the hybrid layer, in contact with the demineralized dentin (Courtesy of Grace M De Souza and published with permission from University of Toronto)
A quaternary ammonium dimethacrylate (QADM) has been recently synthesized and presents strong antibacterial characteristics without compromising the mechanical properties of the restorative resin [102, 103]. In an attempt to reduce the residual bacteria counting in the surface of the tooth cavity preparation as well as to limit the invasion of bacteria at the tooth–restoration interface, quaternary ammonium salt monomers were copolymerized in resins to yield antibacterial activities. The incorporation of QADM and nano-silver particles (nAg – ~2.7 nm) to the primer and/or the adhesive of a commercial adhesive system (Adper Scotchbond Multi-Purpose, 3M) does not negatively affect the bond strength to dentine [63, 64]. Furthermore, the association of the two modified materials (primer and adhesive) has the potential to combat residual bacteria in both the cavity preparation, due to primer diffusion to deeper layers, and at the tooth–restoration interface, where invading bacteria are reduced due to the more potent and long-lasting antibacterial effect [63, 64]. QADM inhibits S. mutans growth on the material’s surface, while nAg-resin inhibits S. mutans both on its surface and away from the surface [104]. The effect of Ag is related to the inactivation of the bacteria’s vital enzymes, causing the DNA in the bacteria to lose its replication ability and leading to cell death [105]. The association of both QADM and nAg in primer suspension also reduces the bacteria count by three orders of magnitude (Fig. 8.3) [106, 107]. This novel antibacterial product has two advantages: it acts as a cavity disinfectant without compromising bond strength and has long-term antibacterial effect in the cured state [106, 107]. Considering that there is no noticeable difference in colour when Ag concentrations are up to 0.2 % mass fraction and that the mass fraction currently being used is in the order of 0.1 %, there is limited concern with regard to colour alteration in the final restoration [63, 64].
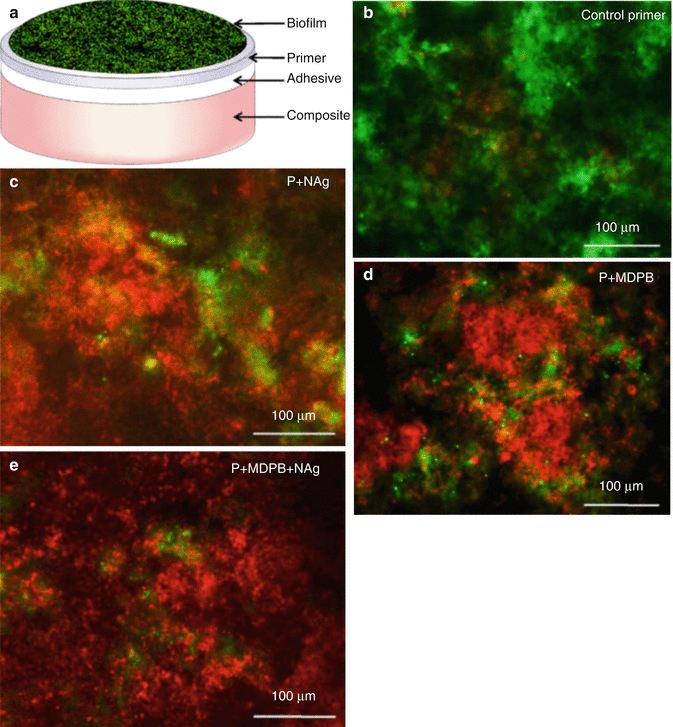
Fig. 8.3
Live/dead bacterial staining assay of biofilms adherent on layered discs: (a) schematic experimental setup; (b–e) representative images of biofilms for control primer, P + nAg, P + MDPB, and P + MDPB + nAg. Live bacteria were stained green, and dead/compromised bacteria were stained red. Live and dead bacteria in close proximity of each other yielded yellow/orange colours (Reprinted from Zhang et al. [106]. With permission from Elsevier)
Besides QADM and nAg incorporation into dentine adhesives, nanoparticles of amorphous calcium phosphate (nACP) have the potential to release Ca and P ions to remineralize tooth structure [108]. The association of nACP with QADM and nAg in dentine adhesives is an attempt to obtain the best of different approaches [109]. It remineralizes demineralized collagen and reduces bacterial counting at the adhesive interface [110]. A new experimental bonding agent containing 0.1 % nAg, 10 % QADM and 40 % nACP (% by mass) reduces biofilm viability and CFU (colony-forming units) to about one-third of the control without affecting bond strength [110]. The incorporation of nACP and nAg to a commercial bonding system (Scotchbond Multi-Purpose, 3M) also does not affect bond strength and presents reduced lactic acid produced by biofilm [109]. NACP may efficiently release Ca and P ions at lower filler levels for composites [96], but this effect has not yet been investigated in dental adhesives. Furthermore, the relatively large size of the particles (~100 nm) [109] represents a problem to their diffusion through dentinal tubules until they reach the demineralized collagen layer. Indeed, even HA nanoparticles with size varying from 20 to 70 nm do not penetrate into the hybrid layer even though they are well dispersed in the matrix [15]. However, high bond strength values are observed because nHA promotes cohesive reinforcement of the adhesive layer since they are homogeneously distributed over it [15]. Besinis et al. [111] observed that besides particle size, dispersion plays a fundamental role in the infiltration of the demineralized dentine matrix as well as on the enamel matrix [112]. The authors demonstrated that the use of a hydrophobic diluent such as acetone for the dilution of nHA instead of distilled water increases the infiltrative ability of nHA in solution by displacing water molecules. Hydrophobic diluent and smaller particles obtained by sol-gel methods appear to be the key to successful infiltration of the demineralized dentine (Fig. 8.2) [111].
Synthesized HA nanorods have also the potential of reinforcing the bonding in one-bottle dentine adhesive systems [113], but there is an inverse correlation between filler concentration and bond strength [15, 113]. With particle length between 30 and 145 nm, 50 % of dentine tubules are fully occluded and, 40 % are partially occluded by the nanorods [114]. The low bond strength may also be related to the incomplete cure of the adhesive [113]. Investigators observed that HA has the potential to remineralize the collagen demineralized by the acid etch. However, the size of the nanorods and their consequent inability to penetrate through the dentinal tubules is a serious limitation for this class of particles.
Colloidal platinum nanoparticles (CPN) are reported as the next generation of antioxidants [115] as they efficiently quench reactive oxygen species [116]. They have been used to manufacture cosmetics and to treat pulmonary inflammation [116]. It is also a low-allergy metal and is widely used as a catalyst in diverse applications [117]. The possibility of increasing the bond strength twice as much as that presented by control groups bonded with 4-META/MMA-TBB (4-acryloyloxyethyl trimellitate anhydride/methyl methacrylate-tri-n-butylborane)–based resin, when the dentine surface was treated with CPN (average particle size ~2 nm), was first evidenced by Nagano et al. [118]. The effect of CPN application on bond strength between 4-META/MMA-TBB–based adhesive (Super-Bond C&B, Sun Medical, Japan) and tooth structure, either prior to or after acid etch (10–3 solution: 10 % citric acid and 3 % FeCl3), has been investigated. The highest bond strength is observed when CPN is applied before etching, evidencing that the platinum effect remained after etching [119]. Denser and longer resin tags are associated with CPN-treated groups [117], and high bond strength may be a consequence of one or more factors: increased monomer infiltration, enhanced adhesive resin conversion in the hybrid layer or better micromechanical interlocking caused by denser and longer resin tags (Fig. 8.2) [117].
The improvement of the mechanical properties in the adhesive/hybrid layer is an alternative to stabilize the bonding, which would then withstand the cyclic loading over the restorative interface [120]. Nevertheless, the incorporation of nanofillers must not compromise initial bond strength values. Spherical zirconia (ZrO2) nanoparticles (size range ~20–50 nm) have been added to either primer or adhesive of a commercial system (Adper Scotchbond Multi-Purpose, 3M Espe) in different concentrations and result in significantly higher bond strength probably due to the reinforcing effect of the adhesive system promoted by filler incorporation, which acts as a barrier to crack propagation initiating from the bottom of the hybrid layer during a microtensile strength test [91]. The incorporation of ZrO2 into the primer aimed to reinforce the hybrid layer by filler infiltration within the collagen network. However, the particles are unable to penetrate the interfibrilar spaces (~20 nm) due to their increased size (~20–50 nm – Fig. 8.2). The incorporation of ZrO2 and ZrCl2 (zirconium chloride) nanoparticles into a self-priming/dentine adhesive (Adper Single Bond Plus, 3M) stabilizes the hybrid layer by maintaining steady values of nanohardness and nanoelasticity after 3 months of storage [120].
Zinc (Zn) is another metal of interest in the dental field. It is widely used as a component of toothpastes, denture retention adhesives, oral rinsing solutions, dental amalgams and others [121]. It has been speculated that Zn and some other divalent metals [122] may inhibit some proteinases. Zn-doped adhesives indeed exert a therapeutic/protective process by which the matrix metalloproteinase (MMP)–induced collagen degradation in the hybrid layer is prevented due to the local release of Zn+2 [120, 123], resulting in stable bond strength by reducing degradation of exposed collagen over time [123], and the effect is more pronounced on self-etch systems, where lesser collagen is left unprotected due to the mildness of the self-etchant [85]. The effect of zinc chloride (ZnCl2) on human dentine evidenced that the collagen MMP–mediated degradation is inhibited not only by zinc’s role in enzyme catalysis but also in protein folding/stability [124]. Interestingly, zinc oxide (ZnO) nanoparticles preferentially remain at the bottom of the hybrid layer in direct contact with the demineralized dentine (Fig. 8.2) [121], but no information about the size of the nanoparticles employed was provided.
The effect of poly(methacrylic acid)-grafted-nanoclay (PMAA-g-nanoclay) as a reinforcing filler and the potential chemical interaction with Ca+2 ions from hydroxyapatite has also been investigated [125–127]. Although significantly high diametral tensile strength and flexural strength [126] as well as superior bond strength to dentine [125] are observed when 0.5 % PMAA-g-nanoclay is added to a commercial dentine bonding, higher concentration of particles should be avoided as this causes agglomeration of PMAA-g-nanoclay, which acts as weak points prone to crack initiation, compromising mechanical properties of the material as well as its bond strength to dentin [125–127]. The agglomeration also results in an inverse correlation between microshear bond strength and concentration of PMAA-g-nanoclay in the adhesive [127].
Apart from filler addition, the polymeric chain of the adhesive may also be reinforced by adding functionalized prepolymers into the adhesive formulation. Nanogels are 10- to 100-nm cross-linked globular particles that can be swollen by and dispersed in monomers such as Bis-GMA and HEMA [128] and are anticipated to carry nanoparticles into the demineralized dentin when added to adhesives [129]. Experimental dentin adhesives containing either UDMA or ethoxylated bisphenol-A dimethacrylate (BisEMA)–based nanogel (particle size range ~10–80 nm) evidenced that the size of the nanogel particles allows their dispersion into dentinal tubules and sometimes into the interfibrilar spaces [128]. The nanogel addition restricts oxygen diffusion rates at the material’s surface, which may reduce oxygen inhibition during adhesive layer polymerization [129]. It also provides significantly higher bond strength to dentin and stable mechanical properties (flexural modulus and flexural strength) after 7 days water storage [129].
The literature search evidences that the results of incorporating bioactive and reinforcing nanofillers to dental adhesives are very promising. Based on the information presented above, the ideal bonding agent should provide high and stable mechanical properties to the hybrid layer and have some level of bioactivity, which would somewhat decrease the incidence of pulpal damage and the occurrence of secondary caries. The high surface area of nanoparticles as opposed to microparticles can contribute to a higher level of ion release and a highly bioactive restorative material. Just for illustration, considering a particle size of 53 nm, the specific surface area is 35.5 m2/g, whereas in a hypothetical 1 μm-sized particle, the specific surface area would be 1.9 m2/g [130]. However, the high surface area of those particles results in two major outcomes: agglomeration of particles that lay on the top of the hybrid layer obliterating the interfibrilar spaces and therefore, avoiding monomer infiltration and hybridization; and the higher the ion release, the less stable is the material. Much effort is still required to develop a material based on ideal ratios between nanoparticles with different sizes and characteristics to ensure high bond strength values and mechanical properties at the hybrid layer that will remain stable over time while reducing bacterial count on the restorative interface.
8.3.2 Restorative Composite Resins, Sealants and Luting Systems
In general, dental composites contain a mixture of at least two different fillers so that reasonable mechanical properties may be combined with radiopacity and some degree of fluoride release [56, 103]. Different manufacturing techniques are employed to obtain the inorganic fillers. Radiopaque glass, quartz or ceramics are generally ground from larger particles until a size between 0.2 and 5 μm is obtained. Other fillers are prepared by the sol-gel route, starting from tetraalkyl orthosilicates or metal alkoxides such as titanium and zirconium ethoxide [131] or different mixtures [56]. The shape of the filler is dependent on the manufacturing process employed [56, 131] and influences the characteristics of the resin-based material [132]. The sol-gel method, for example, results in spherical particles of approximately 5–100 nm (referred to as nanoparticles) with a large specific surface area, which can show a pronounced thickening effect of the resin matrix [72, 132]. This effect can be reduced by using unassociated nanoparticles well dispersed in the matrix [3]. Furthermore, the large surface area still hinders the incorporation of great amounts of nanoparticles, compromising the mechanical properties of the restorative composite [132, 133]. So, it seems necessary to associate nanofillers with other particles capable of reinforcing the matrix and guaranteeing good handling properties [96].
The current composites available for posterior restorations do not release ions capable of remineralizing the tooth structure such as Ca, PO4 and F. In contrast, restorative materials that release those ions are relatively weak and cannot be employed in stress-bearing areas [79, 133]. The incorporation of octyl alkylated quaternary ammonium poly (ethylene imine) (QA-PEI) particles in dental restorative composites has been investigated as a possible strategy to develop antibacterial composites [134, 135]. QA-PEI (1 % w/w) powder (~320 nm) added to a commercial resin composite (Filtek Flow, 3M) demonstrated at least 3 months antibacterial effect against S. mutans, probably due to damage to the bacterial cellular membrane [135], which happens at the material’s surface and not by releasing cytotoxic components [134]. S. aureus inoculated on 1 % QA-PEI nanohybrid composite was eliminated after 24 h, probably due to the loss of their defined cell boundaries, indicating that QA-PEI-based composites are bactericidal and not bacteriostatic [136]. The in situ analysis of QA-PEI-based commercial composites showed biofilm formation on both surfaces. Nonetheless, the biofilm on QA-PEI-based materials had fewer bacteria and indistinct membranes, indicating that cell death at the surface may have triggered a mechanism named ‘programmed cell death’ in the surrounding bacteria [137]. Biocompatibility of QA-PEI nanoparticles has already been evidenced by cell viability and agar diffusion tests [135, 136].
As previously mentioned for dental adhesive systems, amorphous calcium phosphate (ACP) has the potential to remineralize the surrounding tooth structure by transferring mineral ions (Ca and PO4) into the body of the lesion and therefore restoring the mineral lost to acid attack [138]. However, CaP-based composites have low mechanical properties and are not adequate to be employed as bulk restorative materials [108]. The larger surface area of nano-ACP particles (nACP: 17.76 m2/g) [96] as opposed to the surface area of micro-ACP particles (0.5 m2/g) [139] allows for the incorporation of significantly smaller amounts of nACP, leaving room for the placement of reinforcing non-releasing glass fillers [96]. Evaluation of different ratios of nACP indicates that flexural strength and modulus of experimental nanohybrid composites containing either 10 or 20 % nNACP fillers are initially similar to those of hybrid commercial materials [96, 140] and superior after 2 years water storage [141]. Furthermore, the higher the concentration of nACP, the higher the ion release [96], acid neutralization capacity and the bactericidal effect [140]. Interestingly, those materials present a ‘smart’ performance, whereby greater ion leaching is observed in cariogenic pH of 4.0 [96]. Results indicate that experimental nACP-based composites would withstand mechanical challenges of clinical applications where hybrid composites have been recommended [96], but this requires specific clinical investigations. Moreau et al. [140] observed that nACP-based composites may be indicated to areas where complete removal of caries tissue is contraindicated, in early carious lesions and in patients at high caries risk as in the case of patients undergoing radiation therapy and consequently presenting dry mouth [141].
When the resin matrix is modified with quaternary ammonium dimethacrylate (QADM), a strong anti-bacterial monomer, in addition to nACP particle incorporation, pronounced anti-biofilm capacity is observed up to 180 days [102]. The degree of conversion of the experimental material is also higher than that of nACP-based composite without QADM, probably due to the low viscosity of QADM, which improves the mobility of the reactive species. Elastic modulus and flexural strength of nACP-QADM composites are similar to those of commercial composites (Renamel, Cosmedent – microhybrid; Heliomolar, Ivoclar – microfill) after 180 days water immersion [102]. To maximize the caries-inhibiting capabilities of a restorative composite resin, silver nanoparticles (nAg) have been added to nACP-QADM-based composites. The nACP + QADM + nAg composite greatly reduces S. mutans biofilm growth, metabolic activity, colony-forming unit (CFU) viable bacterial counts and lactic acid production when compared to commercial materials (Renamel and Heliomolar) and to QADM and nAg separately. Mechanical properties are comparable to those of the commercial materials [142], but further studies are needed to investigate the long-term effect of that association (nACP-QADM- nAg).
Silver is a safe antibacterial metal that is not toxic for eukaryotic cells, while it is severely toxic and lethal to prokaryotic cells [143]. Addition of nAg to commercial microhybrid composite resins (P90 and Z250 – 3M) increases the contact angle at the materials’ surface, thereby limiting the adhesion and proliferation of bacterial pathogens [144]. However, the synthesis and dispersion of silver nanoparticles are challenging because of the strong propensity for particle agglomeration, reducing the surface energy [145]. The synthesis of cross-linking dimethacrylate/silver nanohybrid composites by coupling photopolymerization particles evidenced that ~3 nm particles were well dispersed throughout the matrix [130]. However, only concentrations as low as 0.02 % do not affect mechanical properties due to the aggregation of nanoparticles in higher nAg concentrations. Higher concentrations are not beneficial to the antibactericidal effect of the resin composites as well [130] and tend to compromise the degree of conversion of the light-cured restorative material [146]. Chemically cured composites with nAg incorporated via benzoate (Bz) may present higher degrees of conversion and higher release of Ag+ due to the slower curing process, which allows for a greater number of nAg nucleation sites to form, thereby generating more particles, a better dispersion of the particles and smaller particle sizes [146]. Light-cured nAg-based materials seem not to be biologically safe since a small concentration of nAg triggers the release of (co)monomers TEGDMA, BisEMA and camphorquinone (CQ) [147]. It is hypothesized that the reflection and scattering of the light by the silver particles would be responsible for the less amount of light reaching out the surface, which reduces the degree of conversion [146, 147].
The effect of nano-zinc oxide (nZnO) incorporation into dental composites may also have a clinically relevant antibacterial effect. Although an experimental material presents mechanical properties similar to those of hybrid materials and S. mutans strains are inhibited at the surface, the penetration of visible light is compromised by the opacity of ZnO, adversely affecting the curing process [148].
Another alternative to control the occurrence of recurrent caries is to increase the dissolution resistance of the tooth structure by the incorporation of fluoride (F) released from restorative materials [149], which is capable of promoting remineralization and inhibiting microbial growth and metabolism [26]. Resin-based materials containing calcium fluoride nanoparticles (nCaF2) present high F release rates within a period of 10 weeks [150]. The stability of CaF2-based composite resins has been recently investigated [151]. The average particle size distribution is about 10 nm, but particles are likely to aggregate, forming clusters as large as 100–300 nm. The mechanical properties of the experimental material are highly dependent on the glass filler particle levels; the higher the glass filler level, the higher the strength during thermal cycling and 2-year water ageing and the better the wear resistance [151]. The nCaF2 paste has a greyish colour due to the metal ion incorporated during the spray-drying process, indicating that the technique needs to be optimized before those particles can be incorporated into clinical restorations [150]. Chlorhexidine (CHX) particles have been combined with nCaF2 and nACP in experimental composites [142] to release CHX in an attempt to achieve a restorative material that promotes remineralization, is antibacterial and withstands load-bearing applications [152, 153]. However, all experimental materials presented decreased flexural strength from day 1 to day 28 in water storage [142]. CHX release rate after 30 days (about 2 %) is similar between nACP- and nCaF2-based composites. This concentration is optimal to slow down or eliminate bacterial growth and to reduce acid production.
Whiskers are single crystals possessing high degrees of structural perfection and high mechanical properties [154]. Silicon carbide whiskers have the potential to reinforce the resin matrix by pinning and bridging cracks during flexural strength tests [29, 155]. The impregnation of whiskers with nano-dicalcium phosphate anhydrous (nDCPA) results in fast release of Ca and PO4 ions due to the large surface area of the nDCPA particles [29], which has been shown to reprecipitate and form hydroxyapatite inside tooth lesions outside the tooth–restoration interface [156]. The smaller the particle size, the larger the surface area and the higher the amount of ion released [30, 157]. The employment of unsilanized nDCPA also releases significantly more ions than silanized nDCPA because the silane coupling agent hinders the diffusion of water and ions through the matrix/filler interface [158]. However, the effect of incorporating unsilanized particles to the restorative materials is not known yet, and it may vary from compromising the mechanical properties to the degradation of the resin matrix due to water/saliva seepage. There appear to be three main factors influencing the Ca and PO4 ion release from the composite: a higher volume fraction of nDCPA in the composite increases (1) the source of ions and (2) the filler–matrix interfacial area, which serves as a path for water and ion diffusion; (3) the resin matrix may have a slightly lower polymerization conversion [29, 79]. Additionally, the opacity of an experimental composite with silica-impregnated whiskers caused by the refractive index mismatch between the whiskers and the resin matrix limits the application of the material [79].
Hannig and Hannig [159] have recently hypothesized that prior to the less abrasive diet of modern days, nano-sized crystallites were found in the oral cavity as a result of physiological wear of the enamel surface due to abrasion and attrition with the food bolus. Those crystallites would be responsible for promoting tooth remineralization and ‘bio-film management’. In a biomimetic approach, hydroxyapatite nanocrystals which resemble the structure of the nano-scale abraded enamel have been employed to reduce bacterial adherence and have an impact on biofilm formation [160]. The comparison among HA whiskers, nanoparticles (~50–100 nm) and sphere particles (~1–3 um diameter) evidenced that the type of HA filler employed has a significant effect on mechanical properties of experimental dental composites and the higher the concentration of HA, the lower the concentration of glass particles and the lower the hardness [161]. Nano-HAs are not homogeneously distributed throughout the matrix, and it is suggested that different sizes of HA fillers should be combined in future studies so that ideal handling characteristics and high mechanical properties can be achieved [161].
Glyoxylic acid (GA), a natural product present in fruits, leaves and sweet beet, has been used to modify the surface of nHA and reduce formation of agglomerates in the resin matrix [133]. Although the nanoparticle dispersion is slightly better in comparison to untreated nHA, agglomerates are noticed when concentration of treated nHA increases to 5 %. The treatment with GA resulted in higher water solubility of the resin matrix, probably due to the weak hydrogen bond established between carboxyl and hydroxyl groups on the GA-modified nano-HA and the resin matrix [133].
Nanotechnology has opened a wide variety of possibilities to improve the clinical performance of resin-based materials. For instance, the association of antibacterial monomers and bioactive glass nanoparticles (~45 nm) may improve fluoride release and reduce microleakage scores of experimental dental sealants [146]. Halloysite nanotubes (HNTs), which are naturally occurring minerals, are easy to purify and available in nature in a large quantity. They are safe and biocompatible and have a diameter of tens of nanometers and length ranging from ~200 nm to 1–2 μm [132]. HNT mixed to an experimental resin matrix indicates that increase in HNT concentration results in higher polymerization shrinkage and low mechanical properties while associated with cluster formation [132]. The effective dispersion of HNT and a consequent improvement of the mechanical properties require further investigation.
The application of nanotechnology into resin-based luting agents has also been investigated. The incorporation of nanoparticles in dental cements is somewhat desirable since it may improve the mechanical properties of the luting systems without increasing the film thickness of the material. However, the incorporation of 2.5 % silica nanoparticles (~7 nm) compromises the flexural strength and film thickness [162
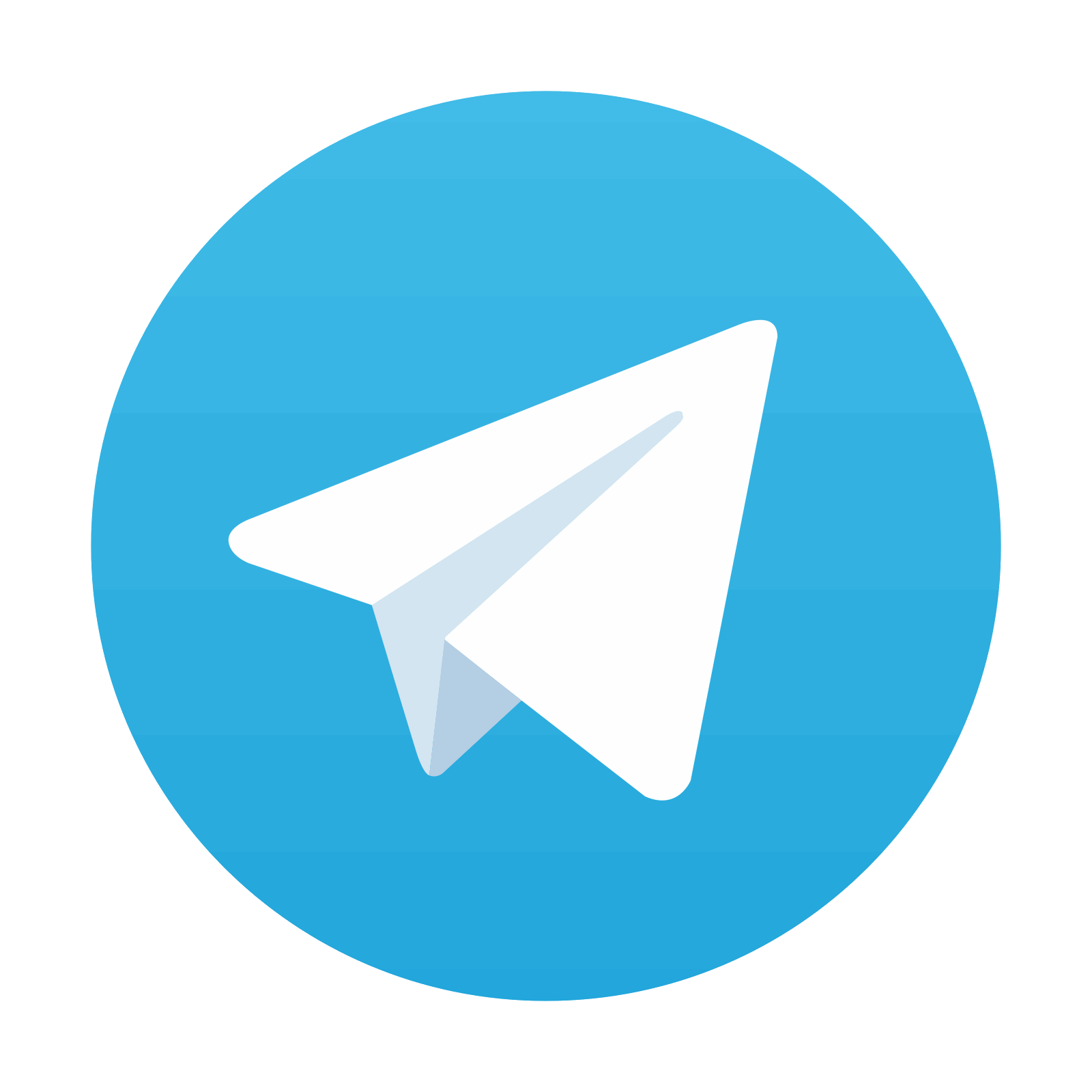
Stay updated, free dental videos. Join our Telegram channel

VIDEdental - Online dental courses
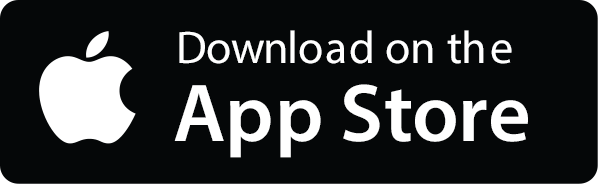
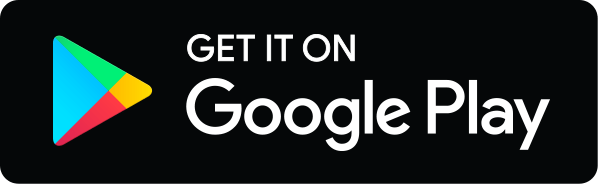