8
Materials and Clinical Techniques for Endodontic Therapy of Deciduous Teeth
Nastaran Meschi, Mostafa EzEldeen, Gertrude Van Gorp, and Paul Lambrechts
Department of Oral Health Sciences, KU Leuven & Dentistry, University Hospitals Leuven, Leuven, Belgium
8.1 Introduction
The necessity of guiding the primary dentition in order to reduce or eliminate functional disorders in the permanent teeth underlines the importance of paediatric dentistry. Morphological differences from the permanent dentition, such as a thinner enamel and wider pulp chamber, render deciduous teeth more prone to pulpal inflammation in cases of carious lesions and traumatic dental injuries. The treatment and preservation of diseased primary teeth aim to fulfil the following objectives: (i) enhancement of aesthetics and mastication; (ii) preservation of arch space, since premature loss of primary teeth may cause aberration of the arch length, resulting in mesial drift of the permanent teeth and consequent malocclusion; (iii) prevention of abnormal tongue habits and promotion of proper speech; and (iv) prevention of the psychological effects associated with tooth loss [1]. The ultimate goal is to preserve the deciduous teeth until the point of physiological exfoliation. An arsenal of treatment modalities has been developed to this end. Compliance of children and their parents or guardians, field isolation, magnification, a tight coronal seal, and biocompatible, nonallergenic, and easily applicable biomaterials are all indispensable in paediatric endodontics. The paediatric clinician requires not only knowledge of endodontic filling materials and a well‐considered assessment of the treatment protocol, but also well‐developed clinical and behavioural skills.
This chapter summarizes the properties and clinical applications of the current most applied endodontic filling materials used in deciduous teeth. Clinical cases with long‐term follow‐up will highlight their technical applicability and outcomes.
8.2 The Primary Dentine–Pulp Complex
The pulp of a primary tooth is histologically similar to that of a permanent one [2]. However, the mesenchymal stem cells available in deciduous teeth – the stem cells of human exfoliated deciduous teeth (SHED) – can differentiate amongst others into neural cells and odontoblasts. Additionally, SHED have the highest multipotency and proliferative capacity of all adult mesenchymal cells investigated to date [3]. This is of paramount importance, as any external factor affecting the dentine will subsequently affect the pulp. Even if the primary dentine–pulp complex response to external stimuli is similar to that of the permanent dentition (namely a reduction in the number of odontoblasts and increase in the number of inflammatory cells), the high proliferative potential of SHED will favour healing of the injury. On one hand, the main factor affecting the dental pulp is bacterial infection via trauma, caries, or microleakage at the restorative material margins [2]. On the other, the toxicity of dental restorative materials is deleterious for the pulp as well. The remaining dentine thickness (RDT) acts as a buffer for the pulp, defines the depth of bacterial penetration, and hence defines the degree of pulpal inflammation. When decay is extended for more than 50% of dentine thickness, more extensive pulpal inflammation is noted for proximal as compared to occlusal carious lesions with similar depth [4]. However, the pulpal diagnosis for primary teeth is less reliable than that for mature permanent teeth, as young patients are less responsive on pulp vitality assessment than adults [5]. Pulp vitality assessment involves pain history and clinical and radiographic examination. For carious lesions implying a deep cavity preparation (RDT < 0.5 mm), the treatment indication depends on the clinical and radiographic assessment. Clinically asymptomatic primary teeth with no lesion periapically or on the furcation level have in general solely coronally inflamed vital pulps and can be saved by indirect pulp treatment or pulpotomy – vital pulp therapy (VPT) techniques (Figure 8.1) [6]. Direct pulp capping of an exposed pulp due to a deep carious lesion is contraindicated in deciduous teeth [7]. If deep carious lesions evoke clinical or radiographic signs, then pulpectomy or extraction is more appropriate, depending on the stage of root resorption (Figure 8.1).
8.3 Pulp Treatments in Deciduous Teeth
8.3.1 Vital Pulp Therapy
8.3.1.1 Incomplete Caries Removal
If a deciduous tooth with a deep carious lesion (RDT < 0.5 mm) is clinically asymptomatic and no bone loss (periapical and on furcation level) is visible radiographically, attempts can be made to keep the pulp vital (Figure 8.1). The International Caries Consensus Collaboration (ICCC) recommends treating deep cavities either biologically by means of selective caries removal to the deepest soft dentine or using the Hall technique, in order to avoid pulp exposure [8]. Direct pulp capping due to a small pulp exposure after complete caries excavation in deciduous teeth has less reliable results than pulpotomy and is thus contraindicated [7]. The Hall technique involves no caries excavation; instead, a stainless‐steel crown is emplaced to stop the carious process [9]. In case of selective caries removal, indirect pulp treatment can be performed by means of stepwise excavation (two sessions) or incomplete caries removal (one session). In both treatment modalities, caries is removed on the coronal cavity walls until hard dentine is reached, in order to allow a good adhesion with the restorative material. Furthermore, the soft dentine on the pulpal floor is left untouched. In cases of stepwise excavation, 8–12 weeks after the first session, the temporary filling is removed and the carious lesion on the pulpal floor is reentered until firm dentine is reached. This reduces the chance of pulp exposure, but working in two sessions requires more compliance from the patient [10]. Field isolation is always recommended unless it is not accepted by the young patient. Magnification by means of loupes or an operating microscope is advisable. In deprived communities, or where there is anxiety over drilling, atraumatic restorative treatment (ART) can be performed [11]. This involves caries removal by means of manual instruments, often without local anaesthetics.

Figure 8.1 Treatment decision tree for deciduous teeth with deep carious lesions, with recommended endodontic/restorative filling material.
As bacterial infection is a critical aetiological factor in pulp necrosis, the restorative methods and materials applied in VPT must reduce or eliminate bacteria. In order to obtain an optimal adhesion with the restorative material, dentine conditioning is advisable. Cleaning of the debris produced during caries removal is possible via application of ethylenediaminetetraacetic acid (EDTA), phosphoric acid, or polyacrylic acid. Due to the chelation of calcium promoted by EDTA and the surface etching caused by all acids, growth factors embedded in the dentine (e.g. transforming growth factor beta‐1 (TGF‐β1) and vascular endothelial growth factor (VEGF)) are released [12, 13], triggering odontoblasts to form tertiary dentine.
Controversy exists over the disinfection of the cavity. The ICCC states that there is no evidence to support the use of cavity disinfectants, as the application of etching agents such as 37% phosphoric acid is enough to remove the smear layer and reduce the number of bacteria left in the prepared cavity [8]. Nevertheless, etching of the soft dentine in deep carious lesions is not advisable. This thin demineralized dentine layer is not an adequate buffer for the pulp against the acidity of etching agents and the toxicity of restorative materials. Hence, a biocompatible buffer should be placed underneath the restorative material, or else the restorative material itself should be biocompatible.
8.3.1.2 Complete Caries Removal
Pulpotomy is the most common treatment for cariously exposed pulps in clinically symptom‐free primary molars. Radiographically, no internal or external root resorption, furcation, or periapical radiolucency should be visible (Figure 8.1) [1, 6, 14]. Magnification by means of loupes or an operating microscope is advisable. After administration of local anaesthetic and rubber dam isolation, all caries is removed with a high‐speed access bur (round end cutting tapered diamond bur) and copious water spray. After lowering the cusps (to remove all undercuts and provide optimal access to the pulp chamber), the entire roof of the pulp chamber is cut away with another high‐speed access bur (round end cutting tapered diamond bur) to avoid carrying bacteria from the carious lesion to the pulp chamber. Copious water spray is used. Coronal pulp is then removed with a #6 or #8 round bur on a slow‐speed handpiece or with a spoon excavator. The pulp chamber is thoroughly washed with sodium hypochlorite 2.5% to remove all debris and dried by aspiration (without air) and application of sterile paper points #130 or #140. Haemorrhage is controlled by use of thick paper points #130 or #140 or a sterile cotton pellet placed against the stumps of the pulp at the openings of the root canals (Figure 8.2).
Pulpotomy can be classified into three main approaches, depending on the material used: (i) preservation of the radicular pulp in a healthy state (by use of ferric sulphate (FS)); (ii) fixation of the radicular pulp by rendering it inert (by use of formocresol (FC); also known as Sweet’s technique [15]); and (iii) encouragement of tissue regeneration and healing at the site of radicular pulp amputation (by use of biocompatible materials) [1, 14, 16].
FS is increasingly used, due to its haemostatic effect, ease of use, low cost, and good long‐term success [14, 17]. It shows a clinical success rate ranging between 78 and 100% and a radiographic success rate between 74 and 100% [14, 16, 18, 19]. Moreover, the success of FS pulpotomy is dependent on the type of cement (pulpotomy base) used to fill the pulp chamber.
Historically, FC was the material of choice as a pulpotomy base in primary molars. It had a clinical success rate ranging between 83 and 100% and a radiographic success rate between 77 and 100% [14, 18]. However, studies have shown that it is mutagenic, genotoxic, and carcinogenic [20–23]. FC is a solution of cresol 35% and formaldehyde 19% in a vehicle of glycerine 15% and water (Buckley’s formocresol). Formaldehyde is classified by the International Agency for Research on Cancer (IARC), part of the World Health Organization (WHO), as a known human carcinogen [24]. Following reports of wide systemic distribution of the medicament after application [1, 14], it has been replaced by other materials.
A biocompatible material is placed at the site of radicular pulp amputation in order to encourage tissue regeneration and healing [1, 14, 16]. Afterwards, the tooth is restored by means of a glass ionomer cement (GIC) or composite restoration or else by a stainless‐steel crown, depending on the restorability of the tooth structure and the caries risk. However, no guidelines exist concerning the restorability of deciduous teeth post‐pulpotomy. Hence, the restorative decision making remains at the clinician’s discretion. If the pulp is hyperaemic and haemostasis cannot be obtained, then pulpectomy or extraction is indicated (Figure 8.1).
VPT requires periodic clinical and radiographic assessment of the treated tooth. The assessment generally should be performed every six months as part of the patient’s periodic oral examinations. Radiographic evaluation of primary tooth pulpotomies should occur at least annually. Bitewing radiographs obtained as part of a patient’s periodic examinations may suffice. However, if they do not display the inter‐radicular area, a periapical radiograph will be necessary [25].

Figure 8.2 Pulpotomy technique. (a, b) Radiographic and clinical presentation for a deep carious lesion on the distal surface of the mandibular second primary molar. (c) Caries removal. (d)–(f) Coronal pulp amputation and control of bleeding. (g) Placement of hydraulic tricalcium silicate cement (Portland cement). (h) Subsequent placement of a layer of zinc phosphate cement. (i) Restoration by means of a composite resin filling. (j) Radiograph directly after treatment.
Source: Josette Camilleri, Courtesy to Gertrude Van Gorp.
8.3.1.3 Restorative Materials for VPT of Deciduous Teeth
To prevent leakage of unreacted monomers from resin‐based materials towards the pulp and enhance pulpal healing, a protective liner or biocompatible material is recommended in deep cavities (indirect pulp treatment) or on the radicular pulp (pulpotomy) [26]. Table 8.1 compares the advantages and disadvantages of several such filling materials. After placement of the cavity liner or pulpotomy base cement, the rest of the cavity must be sealed with a well‐bonded composite restoration or well‐sealed steel crown to prevent any microleakage. Tight sealing of the cavity against bacterial microleakage seems to be the most important success factor in indirect pulp treatment, not the material or technique applied (Figure 8.3) [27].
Table 8.1 Advantages and disadvantages of various filling materials for the endodontic treatment of deciduous teeth.


Figure 8.3 Indirect pulp treatment in two deciduous molars of a healthy 5.5‐year‐old boy without history of spontaneous pain. (Note: Before the age of three, removal of a throat cyst under general anaesthesia involved the administration of a lot of antibiotics.) (a) Bitewing radiograph of grossly decayed right lower and upper second primary molars. Notice a very small visible remaining dentine layer. (b, c) Clinical view (after local anaesthesia and rubber dam isolation) of the right mandibular second molar with a deep carious lesion. (d) Selective removal of soft carious dentine without pulp exposure and with clean cavity walls. The remaining ‘firm’ dentine in the depth of the lesion and the peripheral areas are cleaned with EDTA to enable a good restorative seal. No protective layer is placed. (e) Postoperative bitewing radiograph of the restored (composite filling) right lower and upper second primary molars, serving as a baseline for comparisons with future radiographs to determine treatment success. (f) Three‐year follow‐up showing stable restorations in teeth 85 and 75 (also indirect pulp treatment in the same period). Note the well‐erupted first permanent molars. (g) Panoramic view on four‐year follow‐up, showing teeth 54, 75, and 85 with long‐term stability of the restorations and healthy periapical tissues due to the hermetic seal, a critical condition in the success of an indirect pulp treatment. Note: Full coronal‐coverage restoration with stainless‐steel crowns is preferred, certainly in case of hypomineralization.
Source: Josette Camilleri, Courtesy to Gertrude Van Gorp.
8.3.1.3.1 Calcium Hydroxide
The first materials applied in pulp capping were dentine chips and pastes, including calcium hydroxide (CH) [28, 29]. Historically, CH played an important role in the evolution of VPT. It is antimicrobial and has the ability to extract growth factors from mineralized dentine, which induce reactionary dentine deposition [30]. However, it is caustic to the pulp due to its alkaline pH [29]. In indirect pulp treatment, CH is generally used as a liner. A comparison of the clinical and microbiological effects of CH and gutta-percha when applied in indirect pulp treatment (incomplete caries excavation) in primary teeth after four to seven months found bacterial counts had decreased significantly in both groups [31]. However, the dentine turned hard after treatment in 85% of cases in the CH group, as compared with 68.4% in the gutta-percha one. These results were consistent with a randomized controlled clinical trial in which wax was applied in the control group instead of gutta-percha [32]. Nevertheless, inconsistent conclusions are drawn from reviews; the most evidence exists for the application of CH liners in indirect pulp therapy in primary teeth [33], but CH shows a higher probability of failure when compared with resin-modified glass ionomers and adhesive systems [34].
When used in pulpotomy, CH is applied in a thicker layer. It shows a clinical success rate ranging between 77 and 87% and a radiographic success rate between 66 and 77% [1, 14, 16]. Nevertheless, it has consistently higher rates of internal resorption [1, 14, 16, 17], reducing the chances of preserving the tooth until physiological exfoliation.
8.3.1.3.2 Zinc Oxide‐Eugenol
Due to its low pulpal toxicity and antibacterial properties, zinc oxide‐eugenol (ZOE) is recommended in caries‐free deep cavities [35]. ZOE prevents bacterial penetration towards the pulp in indirect pulp treatment [35]. Hence, the partially decalcified dentine remineralizes and the normal tissue pH is regained [36]. However, in pulpotomy studies, a higher success rate was reported following the use of FS/mineral trioxide aggregate (MTA) in comparison to FS/ZOE or FS/zinc oxide (eugenol‐free) [19].
8.3.1.3.3 Glass Ionomer Cement (Indirect Pulp Treatment Only)
Conventional or auto‐cure GICs consist primarily of alumina, silica, and polyalkenoic acid and are self‐curing restorative materials. GIC is known for its chemomechanical adhesion with dentine and enamel. It is the only restorative material that forms a chemical bond to tooth structures. Additionally, the newly developed chlorhexidine‐containing GIC seems to reduce the level of bacteria in the soft carious dentine [37, 38]. However, all GICs lack the physical properties required for use in load‐bearing areas [39].
Resin‐modified GICs were developed to overcome the disadvantages of conventional GIC. They have a fast set due to polymerization after placement. Furthermore, the resin protects the GIC from dehydration and improves its physicomechanical properties. Resin‐modified GICs do not require a dentine bonding agent. Nevertheless, because they contain hydrophilic resin monomers, they are not as biocompatible as conventional GIC [40].
An observational study of indirect pulp treatment in deeply decayed primary molars applied 2% chlorhexidine gluconate disinfectant after removal of infected dentine. Affected dentine on the pulpal floor remained untouched. A resin‐modified GIC liner was placed and the teeth were restored with composite or stainless‐steel crowns. After 12 months, the treatment was successful in 97% of the teeth. However, appropriate case selection was emphasized [41]. Furthermore, in a systematic review, the clinical and radiographic success of different materials (CH, adhesive systems, resin‐modified GIC, and placebo) applied in indirect pulp treatment in primary teeth was questioned. Follow‐up was conducted at between 24 and 48 months, and the authors concluded that the material type did not significantly affect the risk of treatment failure and that none of the mentioned materials was superior when used in indirect pulp treatment in primary teeth [34].
8.3.1.3.4 Hydraulic Calcium Silicate Cement
The quest for new materials offering optimal clinical performance is never‐ending in the field of dental science. One novel category is the hydraulic calcium silicate cements (HCSCs), which are known for their biocompatibility and bioactivity potential. Several HCSCs introduced and investigated since the early 1990s are discussed in this section (see also Table 8.1).
MTA is mixture of Portland cement and bismuth oxide. It generally comprises tricalcium silicate, dicalcium silicate, tricalcium aluminate, aluminoferrite (in the grey version), gypsum, and bismuth oxide [42]. Bismuth oxide powder is added (17–18 wt%) to make the aggregate radiopaque. The MTA paste is obtained by mixing three parts of powder with one part of water, producing a putty‐like consistency. Mixing can be done on paper or on a glass slab using a plastic or metal spatula. This mix is then placed in the desired location using a large amalgam carrier or spatula and condensed lightly with a moistened cotton pellet [43, 44].
MTA has a pH of 10.2 immediately after mixing, increasing to 12.5 after three hours of setting – similar to that of CH [44]. The mixing time should be less than 4 minutes, and the working time is 70 (±2.58) minutes [43]. Grey MTA has a setting time of about 2 hours and 45 minutes [44], whilst white MTA has one of 2 hours and 20 minutes [45]. It has been used in VPT as it shows excellent biocompatibility [14, 43, 45, 46], promoting tissue regeneration and mineralization ability [1, 14, 43, 46, 47]. In vivo, it has proved better than CH at stimulating reparative dentine formation and maintaining pulpal integrity. It also consistently demonstrates less inflammation, hyperaemia, and necrosis, as well as a thicker and less porous calcified bridge, compared to CH [43]. Clinically, it shows superior results in terms of tissue regeneration ability and long‐term success rates, with a clinical success rate ranging between 94 and 100% and a radiographic success rate ranging between 93 and 100% [1, 6, 14, 16, 18, 19, 43, 48]. Drawbacks include its high price, the blue‐greyish to black discolouration it induces due to its bismuth oxide [49, 50], and its long setting time, which necessitates an additional session for proper setting in order not to jeopardize its physicochemical characteristics [44]. Moreover, clinicians are aware of its poor handling properties: even if the manufacturer’s instructions are strictly followed, mixing the water and powder components in order to obtain a putty consistency is challenging, as is obtaining proper transportation to the tooth and proper adaptation to the region where the MTA is required [51].
Portland cement is produced by grinding cement clinker to a fine powder together with gypsum. Like MTA, it is mainly composed of tricalcium silicate and dicalcium silicate [52, 53]. Both MTA and Portland cement release CH when hydrated, which increases their pH to 12 and makes them antimicrobial [47]. Both show similar compressive strengths, dimensional changes, and setting times [47, 51, 54, 55], and both have similar regeneration and mineralization abilities and biocompatibilities, in vitro and in vivo (animal studies) [45, 47, 54,56–59]. Furthermore, human dental pulp cells show calcified bridge formation following exposure to Portland cement [47, 58]. Hence, it has recently been suggested as an alternative and moderately inexpensive HCSC for use in VPT [47, 51].
One of the drawbacks of Portland cement is that it has a lower radiopacity (similar to dentine) than MTA due to its lack of bismuth oxide [47, 54, 60] (Figures 8.3 and 8.4). On the other hand, this gives it the favourable property of not discolouring the tooth [47, 51, 60]. Studies evaluating leachable elements from different types of MTAs and Portland cements showed high quantities of acid‐extractable trace elements (arsenic, chromium), especially from grey MTA and grey Portland cement. However, the release was negligible in a physiological‐like solution [54, 61, 62].
Clinical trials on indirect pulp treatment with follow‐up intervals ranging from six months [63] to two years [64] show a superior performance of MTA in comparison with CH. For pulpotomy procedures, MTA has been the most clinically applied and investigated HCSC. The current most complete data come from a Cochrane systematic review by Smail‐Faugeron et al. [65], who concluded based on 12 trials (n = 740) that MTA reduced both clinical and radiographic failures when compared with FC, with a statistically significant difference at 12 months clinically and at 6, 12, and 24 months radiographically. When the comparison was made between CH and MTA (4 trials, n = 150), MTA showed significantly fewer clinical failures at 12 and 24 months and radiographic failures at 6, 12, and 24 months. Hence, to date, MTA is the most efficacious and biocompatible pulpotomy base cement for primary teeth, despite its high cost.
In 2008, a novel endodontic biomaterial was introduced as ‘calcium‐enriched mixture’ (CEM; BioniqueDent YektazistDandan, Iran Polymer & Petrochemical Institute Tehran‐Karaj, Iran; US Patent No. 20080206716) [66]. CEM is a newer‐generation hydraulic cement with additives that enhance its material properties (Type 2 material). It consists mainly of calcium salts, calcium oxide, calcium silicate, and calcium phosphate compounds [67]. Like MTA, it is delivered as a powder and a liquid, which are mixed manually just before application. No powder–liquid ratio is specified in the manufacturer’s instructions, but they do mention that the amount of liquid may be varied depending on the required consistency of the cement (putty or creamy). This might jeopardize the material physical properties, since the addition of excess water increases the setting time and reduces the material strength [68–70]; however, it does not seem to affect the biological ones [71]. CEM sets in an aqueous environment and is as antibacterial as CH [72]. Its sealing ability, biocompatibility, pH, mixing time, and dimensional changes are comparable to those of white MTA [73, 74]. However, its initial setting time (50 minutes) and discolouration potential seem to be lower [66, 75] – and these are advantageous properties [66]. CEM is bismuth oxide‐free. Also, it has the ability to maintain the pulp’s integrity and to induce a calcified bridge [76]. Like MTA, it is based on the well‐known tri‐ and dicalcium silicate phases [77]. Furthermore, its radiopacity results from the presence of barium sulphate. It is reported to be 2.2 mm Al – lower than that of white MTA (7.7 mm Al) [43, 67]. The minimum value required by the ISO standards is 3 mm Al [78].

Figure 8.4 Pulpotomy of deciduous molars with various HCSCs: radiographic presentation (pre‐op and one year post‐op) and clinical image (one year post‐op; green arrow = treated tooth). A difference in radiopacity is visible amongst the HCSCs (Portland cement < Biodentine < MTA). With regard to MTA‐pulpotomy one year post‐op, root canal obliteration is remarkable on the radiograph and greyish discolouration can be seen on the clinical image.
Source: Josette Camilleri, Courtesy to Gertrude Van Gorp and Nastaran Meschi.
Biodentine (Septodont, Saint Maur des Fosses, France) was introduced on the market in 2010. It is a newly formulated calcium silicate‐based restorative cement indicated as a dentine replacement material [79, 80]. Hence, VPT is its most suitable clinical indication. Biodentine is available in a capsule containing the optimal ratio of powder (0.7 g) and a single‐dose container of liquid (0.178 mg) [78, 81
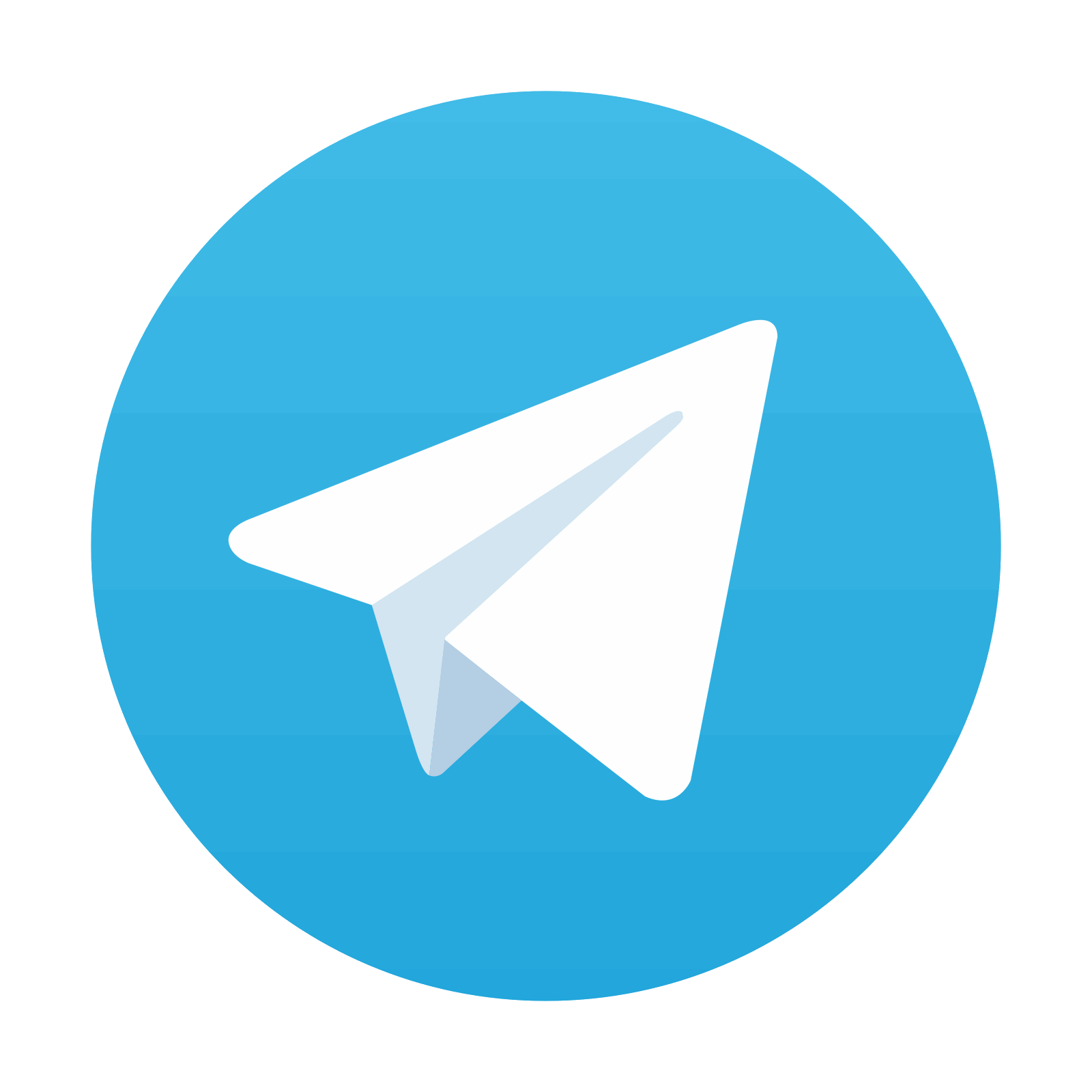
Stay updated, free dental videos. Join our Telegram channel

VIDEdental - Online dental courses
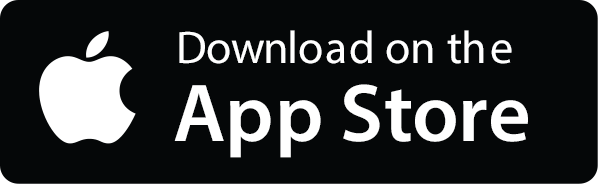
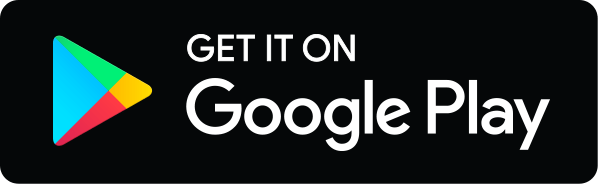