Introduction
The primary purposes of this study were to evaluate how locally delivered zoledronate affects the longitudinal stability of miniscrew implants (MSIs) and the healing of bone around MSIs.
Methods
Using a randomized split-mouth design, 60 unloaded MSIs (5 × 1.6 mm) were placed in skeletally mature male foxhound-mixed breed dogs. The MSIs were randomly assigned to bilateral pairs of pilot holes (1.1 mm) that had been injected with either bisphosphonate zoledronate (n = 30, experimental group) or buffered saline solution (n = 30, control group). MSI stability was evaluated weekly for 8 weeks using resonance frequency analyses (Osstell Mentor; Integration Diagnostics, Göteborg, Sweden). Microcomputed tomography (6-μm voxel size) was used to determine the bone volume fractions of 3 layers of bone (6-24, 24-42, and 42-60 μm) surrounding the MSIs.
Results
Resonance frequency analysis showed that the control MSIs were significantly ( P <0.05) less stable than the experimental MSIs. Although there was little or no change in stability over time for the MSIs treated with zoledronate, the stability of the control MSIs decreased during the first 4 weeks, increased through week 6, and then decreased again. The 6- to 24-μm layer closest to the MSIs, on both the experimental and the control sides, showed significantly ( P <0.05) less bone than did the 24- to 42-μm and the 42- to 60-μm layers. After 8 weeks, there was significantly more cortical bone surrounding the control than the experimental MSIs. In contrast, there was significantly more trabecular bone surrounding the experimental than the control MSIs.
Conclusions
One small locally delivered dose of zoledronate maintained the stability of MSIs over time, primarily because of greater amounts of trabecular bone surrounding the MSIs. Even though zoledronate enhanced the stability of MSIs in dogs, it should not be used clinically until further studies confirm its safe use in patients.
Achieving anchorage control is one of the most important goals of clinical orthodontists. Orthodontic miniscrew implants (MSIs) make it possible to selectively control tooth movement and minimize the side effects. MSIs are small, versatile, minimally invasive, and relatively affordable; they also can be placed and removed by the orthodontist. Reported success rates range from less than 50% to over 95%. The variable predictability of MSI stability limits their usefulness as a treatment modality because of patient discomfort, delay in treatment, alterations in treatment mechanics, and changes of treatment plans.
MSI failures have been associated with the absence of keratinized mucosa, anatomic location of placement, peri-implant tissue inflammation, poor oral hygiene, heavy loading forces, and thin cortical bone. Since mobility is the most common cause for the MSI failures that have been reported, secondary stability depends on primary stability. Primary stability is mechanical and due to the physical properties of the implant, placement technique, and host factors ; secondary stability is due to new bone formation and remodeling around the implant.
Pharmacologic methods hold potential for enhancing the stability of MSIs. Among the most powerful pharmacologic agents available are the bisphosphonates, a class of synthetic drugs that limit bone resorption by inhibiting osteoclastic activity. Bisphosphonates are typically used to treat bone diseases such as osteoporosis, Paget’s disease, multiple myeloma, and metastatic bone cancer. The newest class of nitrogen-containing bisphosphonate with a heterocyclic ring (eg, zoledronic acid) is up to 10,000 times more potent than etidronate.
Bisphosphonates have been used in orthopedic surgery and dentistry to increase the mechanical retention of orthopedic implants, accelerate fracture healing, improve the stability of joint replacements, and increase bone surrounding orthopedic screws. A pilot clinical study showed greater increases in the implant stability quotient values over the first 6 months for endosseous implants coated with bisphosphonates (pamidronate and ibandronate) than for the control implants. Higher pullout forces have also been reported for zoledronate-coated orthopedic miniscrews than for control screws coated with pamidronate. Whereas several animal studies have indicated that it might be possible to obtain a significant local therapeutic effect with small amounts of bisphosphonates around anchorage devices, no studies have measured the changes of stability over time for MSIs coated with zoledronate.
The primary purpose of this study was to evaluate, in vivo, the potential of locally delivered zoledronate for enhancing the stability of MSIs. The aim was to determine MSI stability over time using resonance frequency analysis. A secondary aim was to determine the effects of bisphosphonate on bone around MSIs using microcomputed tomography.
Material and methods
Three periodontally healthy, skeletally mature, male foxhound-mixed breed dogs, 1 to 2 years of age and weighing between 55 and 65 pounds, were used as the experimental model. The Institutional Animal Care and Use Committee at Texas A&M University Baylor College of Dentistry (Dallas, Tex) approved the care of the animals and the experimental protocols. Foxhounds are an established model for investigating peri-implant osseous dynamics; their large mandibles provide space between teeth for the placement of multiple MSIs.
The MSIs were Ancoragem Ortodontica screws (Neodent, Curitiba, Paraná, Brazil); they were self-drilling, made of titanium alloy, and 5 mm long, with a 1.6-mm outer diameter, a 1.1-mm internal diameter, and a 0.7-mm pitch. The head of the screw had a 1-mm-high collar and a 1.1-mm internal thread fabricated to accept the Osstell Mentor Smartpeg type A3 (Integration Diagnostics, Göteborg, Sweden), which was used to measure the implant stability quotient over time. The implant stability quotient/resonance frequency analysis method has proven to be a useful tool for determining the stability of MSIs and endosseous implants. Resonance frequency analysis uses a small transducer that is screwed onto the implant. The transducer has 2 piezoceramic elements attached to it; one vibrates the transducer with a sinusoidal signal, and the other measures the response. The data are represented as the implant stability quotient, which ranges from 1 to 100. Greater implant stability quotient values indicate increased stiffness or stability. Bone-to-implant contact was evaluated 3 dimensionally with microcomputed tomography (model 35; Scanco Medical, Bassersdorf, Switzerland).
The animals were quarantined for 10 days. On the day of MSI placement, they were sedated with a mixture of 2.2 mg per kilogram of body weight of ketamine (Bioniche Teoranta, Galway, Ireland) and 0.22 mg per kilogram of xylazine (IVX Animal Health, St Joseph, Mo). Prophylaxis was performed with ultrasonic scaling instrumentation. The surgical sites were irrigated with 0.2% chlorhexidine solution. The dogs were intubated and given 1% to 1.5% isoflurane (Butler Animal Health Supply, Dublin, Ohio) in oxygen at 1 L per minute. Atropine (0.05 mg/kg) (IVX Animal Health) was given subcutaneously to prevent bradycardia.
Using film size number 4, digital radiographs (Air Techniques, Melville, NY) were taken at various angles, and measurements were taken to determine MSI placement locations ( Fig 1 , A ). Gingival tissue around the surgical sites was irrigated and lavaged with 0.2% chlorhexidine before the administration of local anesthesia (2% lidocaine with 1:100,000 epinephrine; Patterson Dental, St Paul, Minn) with a 27-gauge needle. Radiographic measurements were transferred intraorally via a periodontal probe ( Fig 1 , B ), and implant locations were marked with a tissue marker (3M Unitek, Monrovia, Calif). Gingival tissue was removed using a 1.5-mm tissue punch (3M Unitek) ( Fig 1 , C ), and pilot holes were drilled through the cortical bone only at 16,000 rpm with a 1.1-mm surgical drill (Neodent) under copious irrigation with saline solution ( Fig 1 , D ).
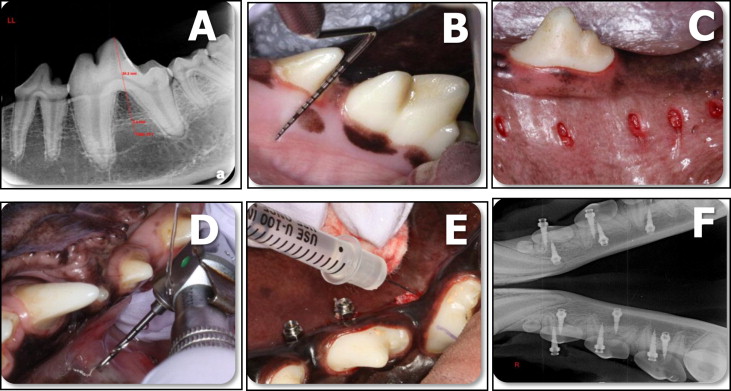
Using random assignment, either 50 μL of phosphate-buffered saline solution (pH 7.1) containing 16 μg of zoledronate (Zometa; Novartis, Basel, Switzerland) or 50 μL of phosphate-buffered saline solution was injected by a blinded operator (C.C.) into bilateral pairs of pilot holes using a 3/10 mL syringe. To prevent contamination of adjacent tissues, the MSIs were spaced apart, the substances were carefully injected into the pilot holes, and gauze was used around every pilot hole to absorb any overflow ( Fig 1 , E ). Immediately after substance injection, the MSIs were hand-inserted until the head of the screw was within 0.5 mm of the cortical bone to standardize the depth of insertion and to prevent countersink friction. They were placed perpendicular to the cortical plate and parallel to the occlusal plane.
Twenty MSIs were placed in each dog. There were 30 experimental and 30 control MSIs. MSI placement was individually planned according to each animal’s anatomy. Ten of the mandibular MSIs were placed from the lingual aspect. All MSIs were placed in the interradicular and interdental areas around the second, third, and fourth premolars, and the first molars, in both jaws. Postoperative peri-implant sites were irrigated with 0.2% chlorhexidine. Periapical radiographs were taken to ensure proper MSI positioning and to verify that the roots had not been contacted ( Fig 1 , F ). Most of the buccal MSIs were placed in nonkeratinized gingiva because of the absence of attached gingiva.
Both analgesics (torbugesic, 0.2 mg/kg [2 mg/mL with 1 mL per animal]) and antibiotics (penicillin G, 60,000 units/kg) were administered postoperatively. Inspection of the MSIs, photographs, prophylaxis with a toothbrush and 2% chlorhexidine gluconate oral gel, and measurement of the implant stability quotient were performed weekly for 8 weeks. For the interim cleanings and measurements, the dogs were sedated with ketamine, 1.1 mL per kilogram, and xylazine, 0.1 mg per kilogram.
Before the study, the Osstell transducer was calibrated according to the manufacturer’s instructions. After MSI placement, the Smartpeg mount was magnetically connected to the Smartpeg type A3, screwed into the miniscrew’s head, and tightened with finger pressure according to the manufacturer’s instructions. To minimize possible MSI movement while screwing and unscrewing the Smartpeg, the MSI’s head was secured with a hemostat ( Fig 2 , A ). The Osstell’s transducer was oriented perpendicular to the long axis of the MSI ( Fig 2 , B ), and 4 blinded implant stability quotient measurements were made around the MSI, each 90° apart. The 4 measurements were averaged. Each MSI had 9 implant stability quotient values: the initial one, taken at placement, plus 8 weekly measurements. The reliability of the repeated measurements was high, with an intraclass correlation of 0.959.
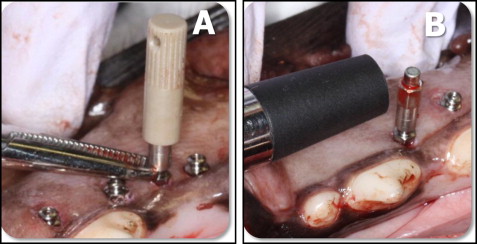
Eight weeks after MSI placement, the animals were euthanized with 2 mL of Beuthanasia-D (Schering Corp, Kenilworth, NJ) given intracardially and perfused with 1 to 2 L of normal saline solution, followed by 1 L of 70% ethanol. The maxillae and mandibles were resected en bloc and stored in 70% ethanol until analysis.
All specimens underwent microcomputed tomography analysis. Each bone-implant specimen was retrieved with a 10-mm trephine bur (ACE Dental Implant System, Brockton, Mass) under copious irrigation. The specimens were trephined parallel to the long axis of the implant, ensuring that all 3 layers of the bone (cortical, medullary, cortical) remained intact and undamaged during the retrieval process, and stored in 70% ethanol. A maximum of 5 bone-implant specimens was placed (oriented parallel to the long axis of the holder) in each holder, along with 70% ethanol ( Fig 3 , A ). A 2-dimensional radiographic image of the specimens was used to determine the volume of interest to be scanned ( Fig 3 , B ). The specimens were then scanned using microcomputed tomography with a high isotropic resolution of 6 μm. X-ray energy levels were set to 70 kVp, current was set to 114 μA, and integration time was 800 ms. A 0.5-mm aluminum filter and a high-resolution setting of 1000 projections per 180° were used to ensure the highest-quality scans with minimal metal implant artifacts.
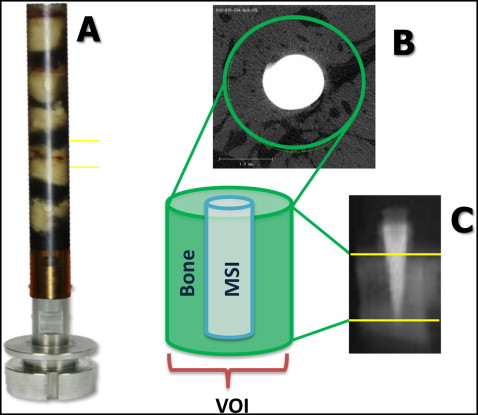
The volume of interest of each specimen was defined as a cylinder (430 pixel diameter), with the implant positioned in the center ( Fig 3 , C ). The apical limit of the volume of interest was set to 10 slices (0.06 mm) coronally from the MSI tip ( Fig 3 , D ). The average scanning time was 3.8 hours per specimen. After scanning, thresholds (1 gray scale or “brightness” number, above which all voxels were considered bone, and below which all voxels were considered nonbone) were determined using 10 randomly chosen specimens.
From the reconstructed 3-dimensional images, cortical and trabecular bone areas were delimited for each specimen; the mixed bone between was not evaluated ( Fig 4 , A ). Four Scanco Medical proprietary programming scripts with different parameters for bone-implant contacts were processed per sample. For each specimen, separate bone volume fractions were calculated for the cortical and trabecular layers. The coronal and apical limits of cortical bone within the volume of interest were visually defined as the first and last slices with cortical bone completely surrounding the MSI ( Fig 4 , A ). The same rules were used for the trabecular bone. The calculated areas of interest included 3 layers of bone, each 3 voxels (18 μm) thick, 6 to 24, 24 to 42, and 42 to 60 μm from the MSI surface ( Fig 4 , B ). The voxel of bone adjacent to the MSI (0-6 μm) was excluded because it was subject to metallic halation artifacts. The software made it possible to separate the MSI and the mineralized bone, allowing for better visualization of each layer ( Fig 4 , C ); a 3-dimensional image of bone-implant contact, with the MSI and supporting bone removed, was also obtained ( Fig 4 , D ).
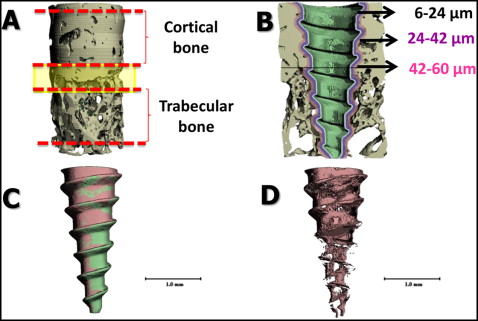
Statistical analysis
The data collected with the Osstell Mentor were not normally distributed. The skewness and kurtosis were statistically significant ( P >0.05). To control for initial differences, changes in stability were evaluated relative to baseline values. Wilcoxon signed rank tests were used to compare changes in stability over time and also to evaluate differences between the experimental MSIs and the controls. All statistical testing was performed with SPSS software (version 17.0; SPSS, Chicago, Ill).
To standardize the relative number of slices between the most coronal and apical aspects of each layer, the slice numbers were divided by the total number of slices in each layer, producing relative section thicknesses from 0% to 100%, with 100% representing the most apical slice of each layer. Multilevel statistical models were used to determine the changes within each layer and to statistically evaluate differences between the control and the zoledronate groups. The models were developed with MLWiN software (Center for Multilevel Modeling, Institute of Education, London, United Kingdom) using iterative generalized least squares. The preliminary analysis showed that the repeated measurements of bone volume fractions were best described by a simple linear regression model. The intercept term described the bone volume fraction at 100% relative section thickness (ie, the most apical slice), and the slope described the changes in bone volume fractions for each percent of change of relative section thickness. Three-level multilevel models were used, with random variation partitioned between dogs at one level, between MSIs at the second level, and between slices at the next level.
Multilevel procedures were chosen because the models used make no assumptions as to the equality of the intervals used for the independent variable. Most importantly, multilevel procedures make it possible to statistically determine the appropriate models and to evaluate group differences.
Results
Of the 60 MSIs that were placed ( Fig 5 , A ), 2 failed (3.3%) during the study. Two (3.3%) more MSIs had to be removed a few days after placement because of root proximity, and prophylactic root canals had to be performed. Four (6.7%) MSIs were considered “mobile” or unstable, with implant stability quotient readings less than 25 and exhibiting clinical signs of mobility. Of the 58 MSIs that remained in place throughout the experiment, 52 (89.7%) showed implant stability quotient readings above 25 for all 8 weeks of the experiment.
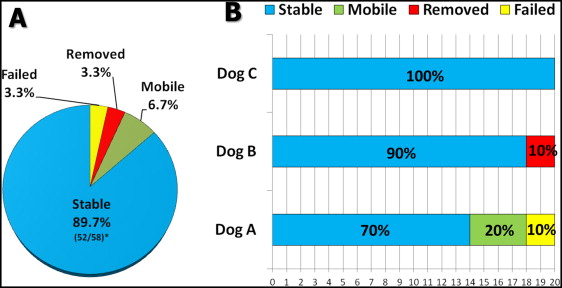
Seventy percent of the MSIs placed in dog A (14 MSIs) showed implant stability quotient readings higher than 25 throughout the experiment ( Fig 5 , B ). Two MSIs (1 experimental, 1 control) in dog A failed. Both showed low implant stability quotient readings 4 weeks after placement and came loose during the fifth week while the Smartpeg was being unscrewed from the MSI head. Both had been placed in nonkeratinized tissue in the posterior region of the right mandible.
Four MSIs placed in dog A showed implant stability quotient readings below 25 and clinical signs of mobility. Two of those screws were placed in antimeric anterior regions of the maxilla between the lateral incisor and the canine (1 control, 1 experimental). Lower implant stability quotient readings were recorded as early as 1 week after placement. No implant stability quotient readings were obtained in the sixth and seventh weeks from 1 MSI because of mobility. The other 2 mobile MSIs were located in the posterior region of the mandible, in the furcation of the third molar (1 control, 1 experimental). Their implant stability quotient values dropped below 25 during the third and fourth weeks. Of the 14 MSIs that remained stable in dog A throughout the experiment, 2 that had been placed in nonkeratinized tissues in the maxillary first molar furcation area were buried because of excessive tissue overgrowth; both remained under the tissues for the rest of the experiment and showed excellent stability at the last week before the dog was euthanized.
There were no failures in dogs B and C. Two screws placed in dog B were removed because of root proximity. The remaining 18 MSIs (90%) in dog B had implant stability quotient readings above 25 throughout the experiment, as did the MSIs placed in dog C ( Fig 5 , B ).
The implant stability quotient values of the experimental and control MSIs were initially similar ( Table I ). Compared with their initial baseline values, the experimental MSIs showed a statistically significant ( P <0.05) loss of stability during the first week; after the first week, no implant stability quotient value differed significantly from baseline ( Fig 6 ). In contrast, all implant stability quotient values of the control MSIs were significantly less than the baseline values. The implant stability quotient values recorded at the end of the sixth week most closely approximated the baseline values. There were statistically significant differences in implant stability quotient values between the experimental and control MSIs after the fourth, fifth, sixth, and eighth weeks.
Time frame | Control group | Experimental group | Group comparison | ||||||
---|---|---|---|---|---|---|---|---|---|
Percentile | Difference from baseline | Percentile | Difference from baseline | ||||||
25 | 50 | 75 | P value | 25 | 50 | 75 | P value | P value | |
T0 | 37.9 | 45.0 | 52.0 | NA | 40.6 | 44.8 | 49.8 | NA | 0.750 |
T0-T1 | −4.9 | −1.5 | 0.6 | 0.004 ∗ | −3.4 | −0.8 | 0.3 | 0.007 ∗ | 0.434 |
T0-T2 | −4.0 | −2.0 | 2.0 | 0.049 ∗ | −1.4 | 0.3 | 2.0 | 0.716 | 0.084 |
T0-T3 | −7.5 | −3.5 | 0.5 | 0.002 ∗ | −3.9 | −0.5 | 1.0 | 0.065 | 0.151 |
T0-T4 | −7.2 | −4.3 | −1.5 | <0.001 ∗ | −4.5 | 0.0 | 1.5 | 0.187 | 0.018 ∗ |
T0-T5 | −6.5 | −3.5 | 1.0 | 0.004 ∗ | −2.0 | 1.0 | 3.5 | 0.791 | 0.029 ∗ |
T0-T6 | −5.9 | −1.4 | 1.6 | 0.045 ∗ | 0.0 | 2.0 | 4.0 | 0.069 | 0.010 ∗ |
T0-T7 | −9.1 | −3.8 | 1.5 | 0.011 ∗ | −1.8 | 0.0 | 3.0 | 0.710 | 0.075 |
T0-T8 | −10.3 | −6.0 | 1.4 | 0.003 ∗ | −2.3 | 1.1 | 3.7 | 0.548 | 0.017 ∗ |
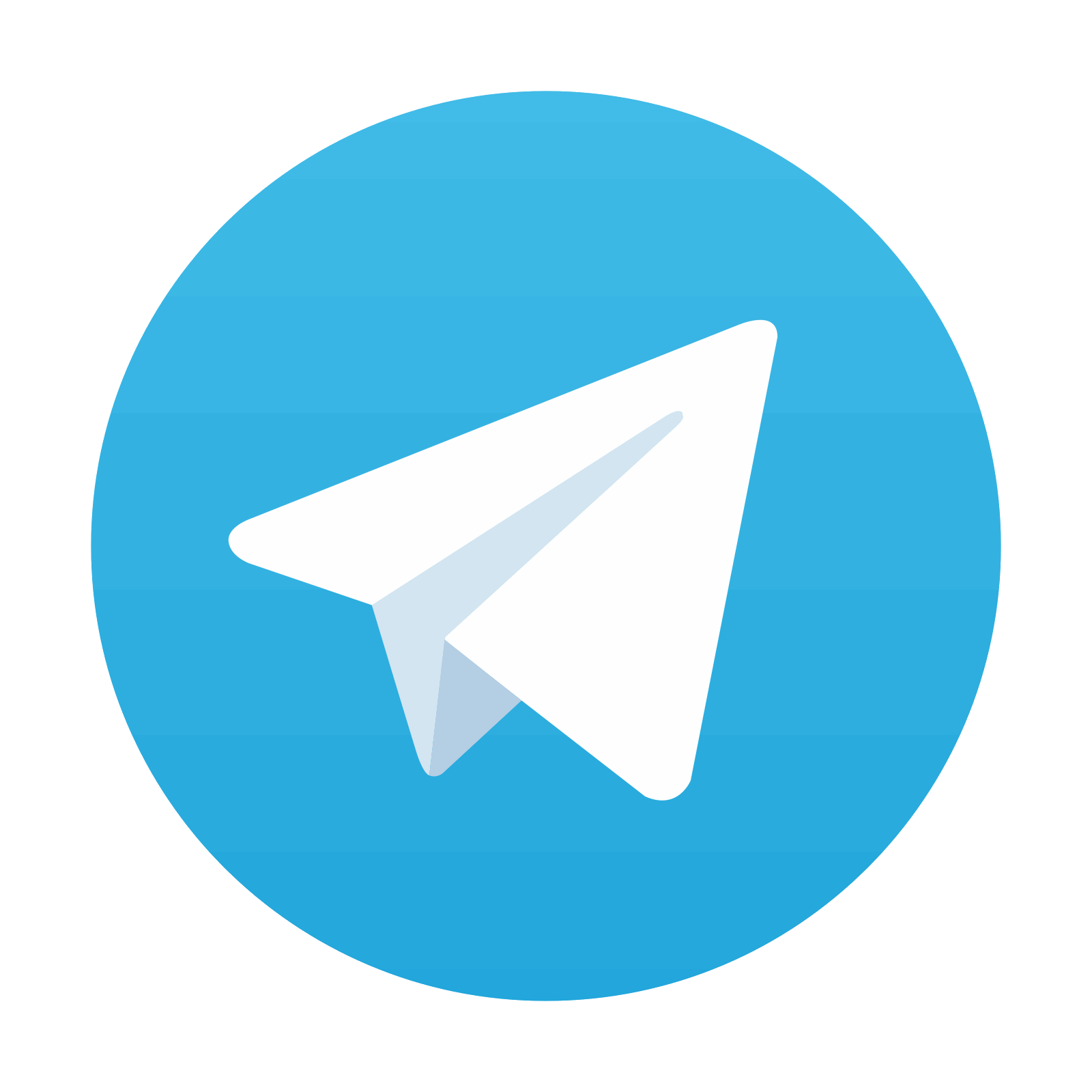
Stay updated, free dental videos. Join our Telegram channel

VIDEdental - Online dental courses
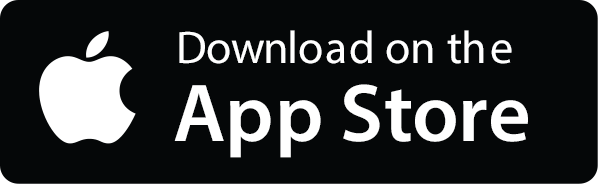
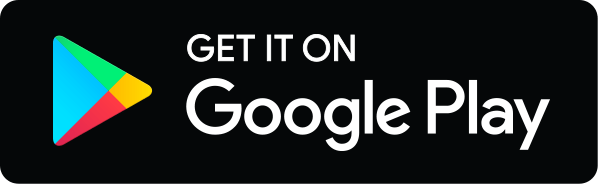