Graphical abstract

Abstract
Objective
Maxillary sinus floor augmentation (MSFA) is commonly used to increase the alveolar bone height in the posterior maxilla before implant placement. In the present study, we evaluated if the injectable thermosensitive chitosan/β-sodium glycerophosphate disodium salt hydrate/gelatin (CS/GP/GA) hydrogel carried erythropoietin (EPO) could enhance the new bone formation for MSFA in vivo .
Methods
EPO-CS/GP/GA hydrogel was prepared by ionic crosslinking. Then, characteristics of EPO-CS/GP/GA were evaluated by morphology, injectable property and pH on the gelling time (GT). The release profile of EPO was evaluated by enzyme linked immunosorbent assay (ELISA), and effects of EPO on proliferation and osteoblastic differentiation of bone marrow stromal cells (BMSC) were analyzed by 3-[4,5-dimethylthiazol-2-yl]-2,5-diphenyl tetrazolium bromide (MTT) and reverse transcription quantitative real-time PCR (RT-qPCR), respectively. Finally, EPO-CS/GP/GA was injected into the maxillary sinus floor of the rabbit to test the potential application for MSFA.
Results
Results showed that GT was decreased with the increase of pH value. The GT was 110 ± 15 s at pH 7.0. SEM images showed that the CS/GP/GA hydrogel had a sponge network structure. Results from ELISA assay revealed that the cumulative release of EPO from the EPO-CS/GP/GA hydrogel reached 67% at 4 h, and 94% at 15 days. MTT assay showed that EPO within EPO-CS/GP/GA hydrogel could significantly promote proliferation of BMSCs compared to control group ( p < 0.001) . Results of RT-qPCR assays demonstrated that the expression of Sp7 , Runx2 , Col I and Alp were significantly increased from EPO-CS/GP/GA group compared to control group on day 14 ( p < 0.001). Importantly, EPO-CS/GP/GA hydrogel could significantly induce bone formation (81.98 mm 3 ) compared with control group (43.11 mm 3 ) after 12 weeks post-implantation in vivo . The calculation of thickness of mesenchymal condensation indicated that thickness of mesenchymal condensation was significantly increased from EPO-CS/GP/GA group (∼121.4 μm) compared to control group (∼37 μm) resulting in enhancing intramembranous ossification.
Significance
The EPO-CS/GP/GA hydrogel provides a novel strategy for MSFA with a minimally invasive way.
1
Introduction
The pneumatization of the maxillary sinus and atrophy of the alveolar bone is still an important factor to affect implantation of posterior maxilla . Maxillary sinus floor augmentation (MSFA) is regularly used to increase the alveolar bone height in the posterior maxilla before implant placement . Bone-grafting materials are simultaneously implanted into the elevated maxillary sinus with tooth implant . Many synthetic bone-grafting materials, such as biphasic calcium phosphate (Straumann Boneceramic®) , hydroxyapatite crystallites (NanoBone®) , deproteinized bovine bone (Bio-Oss®) have been widely used for MSFA to enhance bone formation . These commercially available bone-grafting materials, however, have some disadvantages or limitations, such as prolonged inflammatory response, mucosa membrane tears especially for trans-crestal sinus floor augmentation, host limitations in dealing with large volumes of materials and longer conversion times . Most of these materials are not a good carrier for growth factors to achieve sustained release of the growth factors in order to effectively accelerate bone formation using the growth factors in vivo . Therefore, a novel implant material is still needed.
Hydrogel is a remarkable crosslinked 3D polymeric network material and conducive to biomedical applications . Interestingly, hydrogel is an injectable material leading to simple surgical procedure and less trauma, and easy to fulfill the irregular lacunae of the maxillary sinus due to its good fluidity before gelation with good degradability and bio-compatibility. Importantly, the hydrogel is a good carrier for sustained release of growth factors
Recently, a thermosensitive hydrogel was developed . This kind of hydrogel has broad applications, such as carrying bioactive molecules and cells, and filling irregular bony lacunae . Natural polymers are more favorable for bone regeneration over synthetic polymers due to their structural resemblance with natural bone components and high biocompatibility . Chitosan (CS) is natural poly-saccharide which is structurally similar to glycosaminoglycans with excellent biocompatibility . Chitosan based hydrogels have thermal and pH-triggered gelation properties, which can be applied with a minimally invasive manner in clinic . The injectable chitosan-based hydrogel has been used for nerve, cartilage and bone regeneration . Since chitosan is a cationic polymer, it can bind to other glycosaminoglycans (GAGs) or proteoglycans through electrostatic interactions, while GAGs can regulate the expression and release of osteogenesis-associated cytokines and growth factors . Thus, chitosan is a good scaffold material for bone tissue repair.
The key process for preparing chitosan hydrogel is ionically or covalently cross-linking by different compounds resulting in a biocompatible and well-tolerated hydrogel . Chitosan is a polycationic polymer that can reaction with negatively charged components, such as β-sodium glycerophosphate disodium salt hydrate (GP), citrate and calcium phosphate, which lead to the formation of a network through ionic bridges between polymeric chains. GP is one of the most commonly used crosslinker due to its biological role . GP can promote multiple osteogenesis-related gene expressions, such as collagen I (Col I), alkaline phosphatase (Alp) and osteopontin (OPN). Besides, GP can also increase amounts of phosphate resulted in increasing alkaline phosphatase activity, collagen content and calcification . High concentration of GP in the CS/GP hydrogel, however, can cause cytotoxic effects .
The performance of CS/GP hydrogel can be enhanced by introducing of a third components, such as gelatin (GA) or starch, which can increase the interconnected pore size and degradation time. The presence of starch in the system reduces the gelation time and increased the water absorption of the hydrogel leading to a good cytocompatibility . GA can make CS/GP system to accelerate crosslinking between chitosan and sodium glyceryl phosphate with shorter gelation time by electrostatic interaction . GA is an amorphous complex, and can obtain by thermal denaturation of collagen and proteins (most type I collagen) extracted from skin or bones of land animals . In addition, type I collagen, as main organic component of the extracellular matrix of bone, serves as the template for bone formation . Therefore, incorporation of GA can mimic bone extra cellular matrix (ECM) and create an osteogenic microenvironment. Gelatin has been widely used for bone tissue engineering or improving osteogenic effects and biocompatibility of synthetic biomaterials .
BMP2/7 are well-known growth factors to possibly regenerate bone tissue for the bone diseases . Data from previous clinical trials, however, indicate that BMP2 can cause certain side effects, such as inflammation, tissue swelling and cyst in patients . The erythropoietin (EPO) is another interesting growth factor for the bone repair. Our previous study has demonstrated that EPO can promote bone regeneration by regulating the EphrinB2/EphB4 signal axis between osteoblasts and osteoclasts . In addition, numerous studies have confirmed that EPO can promote osteoblasts proliferation, differentiation and angiogenesis . EPO can also directly stimulate osteoclast precursors resulting in bone loss or cause bone loss in mice due to lacking prolyl hydroxylase domain 2 (PHD2) in EPO-producing cells . Moreover, little is known about the potential regenerative effects of EPO on MSFA in vivo .
Therefore, in our current study herein, we evaluated if our novel CS/GP/GA hydrogel system plus EPO has extraordinary effects on bone regeneration for the MSFA in vivo . Briefly, results showed that our hydrogel delivery system, EPO-CS/GP/GA could sustain EPO release of and effectively promote the maxillary sinus lifting in rabbits through intramembranous osteogenesis in vivo .
2
Materials and methods
2.1
Preparation and characterization of CS/GP/GA thermosensitive hydrogel
CS solution was prepared by dissolving 2 g of chitosan (Millipore Sigma, St Louis, MO USA) into 100 ml of 2% (v%) HCL solution and stirred overnight. GA and GP solutions were prepared using 0.5% (w/v) gelatin (type A, Millipore Sigma) and 56% (w/v) β-glycerophosphate disodium salt hydrate (Mw 216.04, Merck, Darmstadt, Germany) and filtered with 220 nm filter. To check the effects of pH on gelation time, CS, GP and GA solutions were uniformly mixed together with a fixed volume ratio of 10:2:0.25 at room temperature. The gelling time was determined at various pH values (4.0, 5.0, 6.0, 6.5, and 7.0, respectively) at 37 °C in a water bath. For cell cultures or in vivo experiments, CS/GP/GA mixture was filtered first for sterilization.
Briefly, 5 ml of CS was added into a 20 ml sterile ampule, then 1 ml of GA and 0.125 ml of GP were added dropwise to the CS solution under continual stirring. The pH value was adjusted with a 0.1 M NaOH solution. After the solution was uniformly mixed, the ampoule was placed in a 37 °C water bath. The ampoule was checked every 15 s by taking ampoule out and tilting 45 degrees to observe if the liquid level of the mixed solution was inclined with the ampoule. When the liquid level was not inclined, the ampoule was incubated for extra 30 s, then took the ampoule out, completely inverted, and did not undergo significant morphological changes within 15 s, which deemed to have hydrogel cured. The time required from liquid to the solid hydrogel was the gelling time (GT). The experiments were repeated three times under the same conditions.
In order to verify the injectable property of the hydrogel, the mixed solution was aspirated in 5 ml syringes using 21G needle, then squeezed out to write the word “gel” in a culture dish. Surface morphology of lyophilized CS/GP/GA hydrogel was evaluated by scanning electron microscope (SEM, JSM-5600 LV, JEOL Ltd., Tokyo, Japan).
2.2
Release profile of EPO from the hydrogel
To evaluate release profile of EPO, 1000 unit of EPO (Sansheng Pharmaceutical Co., Ltd., Shenyang, China) was loaded into 2 ml of CS/GP/GA thermal sensitive hydrogel at pH 7.0. Two g of EPO-CS/GP/GA hydrogel was added in 10 ml of phosphate buffer saline (PBS, Millipore Sigma) and incubated in a 37 °C water bath. Supernatant was aspirated and replaced with 10 ml of PBS at 2 h, 4 h, 8 h, 24 h, 2 days, 4 days, 10 days and 15 days after incubation. The same experiment was performed and collected supernatants at 2 h, 4 h, 8 h, 24 h, 2 days, 4 days, 10 days and 15 days were used for proliferation and differentiation assays in Section 2.4 . The EPO concentration in each supernatant was determined by ELISA (STEMCELL Technologies Inc., Vancouver, British Columbia, Canada) and the cumulative drug release was calculated.
2.3
Isolation and culture of bone marrow stromal cells
All animals used in this study were approved by Jilin University Animal Care and Use Committee. Animal experiments were carried out in accordance with requirements of the Experimental Animal Ethics and Welfare guidelines (Permit Number: 201611). Rat bone marrow stromal cells (BMSCs) were isolated from femurs of 4-week-old rats. Briefly, bone marrow cells were flushed out using a 20 mL syringe and plated in a 25 mL cell culture flask (NEST, Wuxi, China).Cells were cultured in low-glucose DMEM (L-DMEM; Thermo Fisher Scientific, Waltham, MA, USA) supplemented with 10% fetal bovine serum (FBS, Thermo Fisher Scientific), 100 mg/mL of streptomycin (Thermo Fisher Scientific), and 100 U/mL of penicillin (Thermo Fisher Scientific) at 37 °C, in humidified 5% CO 2 incubator. The medium was replaced on day 4, then changed every 3 days. The third passage of BMSCs was used for all experiments in this study.
2.4
Cell proliferation and osteogenic differentiation assays of BMSCs
To evaluate effects of EPO-CS/GP/GA hydrogel on BMSCs proliferation, BMSCs were cultured at 1 × 10 4 cells/well in a 96-well culture plate for 24 h. Then, the medium was replaced with fresh medium plus supernatant collected as mentioned in Section 2.2 at 50 U/ml of EPO and cultured for 48 h. BMSCs with extracts of PBS collected from CS/GP/GA hydrogel was used as a negative control group (control group). EPO only was used as a positive control group (EPO group). After 48 h, MTT (thiazolyl blue tetrazolium bromide) assay was used to determine BMSC proliferation (Baotek Biotechnology Co., Ltd., China).
BMSCs were cultured at 2 × 10 4 cells/well in a 6-well cell culture plate for 24 h. Then, medium was replaced with fresh medium plus supernatant collected as mentioned in Section 2.2 at 50 U/ml of EPO and cultured for 3, 7 and 14 days, respectively. BMSCs plus PBS collected from CS/GP/GA hydrogel was used as a negative control group (control group). At different time points, cells were collected, and total RNAs were extracted using Qiagen RNeasy mini purification kit (Qiagen, Valencia, CA, USA). The cDNAs were synthesized using iScript™ cDNA synthesis kit (Takara Bio Inc., Dalian, Liaoning, China). RT-qPCR was performed to determine expression profiles of osteoblastic marker genes, Alp, Runx2, type I collagen (Col I), and Sp7 (MxPro Mx3005P; Agilent Technologies, Santa Clara, CA, USA) using SYBR-Green premix Ex Taq kit (Takara Bio Inc., Dalian, Liaoning, China). Housekeeping gene β-actin was used as the internal control. All primers used for this analysis were purchased from Takara Bio ( Table 1 ).
Genes | Forward sequence/reverse sequence |
---|---|
β-actin | 5′-GGAGATTACTGCCCTGGCTCCTA-3′/ 5′-GACTCATCGTACTCCTGCTTGCTG-3′ |
Col I | 5′-GACATGTTCAGCTTTGTGGACCTC-3′/ 5′-AGGGACCCTTAGGCCATTGTGTA-3′ |
Runx2 | 5′-CATGGCCGGGAATGATGAG-3′/ 5′-TGTGAAGACCGTTATGGTCAAAGTG-3′ |
Alp | 5′-CATCGCCTATCAGCTAATGCACA-3′/ 5′-ATGAGGTCCAGGCCATCCAG-3′ |
Sp7 | 5′-AAGTTATGATGACGGGTCAGGTACA-3′/ 5′-GAAATCTACGAGCAAGGTCTCCAC-3′ |
2.5
Animal model
In this study, New Zealand white rabbit was used to create animal model because its maxillofacial region has a large area, and the maxillary sinus surgical approach is simple . Ten New Zealand white rabbits (2.5 ± 0.2 kg) were randomly divided into two groups, EPO-CS/GP/GA group and PBS-CS/GP/GA group. The experimental model of MSFA was established based on a previous study . Rabbit was anesthetized using pentobarbital sodium at 30 mg/kg body weight intravenously. A 2 cm incision was made at the median nasal line and 1.5 cm above the tip of the nose after the skin been sterilized with iodine. Then, the skin was pulled laterally, and periosteum was peeled off to fully expose bone surface. Two circular bone defect windows with a diameter of 0.5 cm drill were created in the anterior region of naso-frontal suture and 0.5 cm lateral from the median line. Injury of maxillary sinus mucosa should be avoided while drilling. Finally, the maxillary sinus mucosa was pushed backwards to prepare 5 mm × 5 mm × 5 mm of space ( Fig. 1 ).

Freshly prepared EPO-CS/GP/GA (25 μl/rabbit contained 62.5U EPO) or PBS-CS/GP/GA (25 μl/rabbit) hydrogels were pipetted to micro-injector, then injected into the maxillary sinus space prepared above. Periosteum and skin were sutured sequentially after hydrogel coagulation ( Fig. 1 ).
2.6
Radiographic analysis
Rabbits were sacrificed at 6 or 12 weeks after hydrogel injection. Skull specimens were collected and fixed with 4% paraformaldehyde for 2 days. Cone Beam Computed Tomography (CBCT) was used (field 5 × 5 cm, resolution 90 μm, voltage 84 kV, current 4.0 mA, time 20 s, total dose 478 mGy.cm 2 ) to determine the amount of new bone formation and sinus membrane elevation in the maxillary sinus floor. Materialise Mimics V17.0 software was used to analyze the new bone volume of sinus floor areas (Materialise, Leuven, Belgium).
2.7
Histological analysis
After CBCT scanning, the maxillary sinus was dissected in sagittal at the surgical site, then the samples were decalcified in 10% EDTA for 3 months and the solution was changed every week. The samples were embedded in paraffin and sectioned at 5 μm of thickness for H&E (hematoxylin and eosin) staining. The H&E staining slides were evaluated under an optical microscope, and the images were captured under the Olympus microscope BX51 and DP73 CCD Olympus Imaging System (Olympus Corporation, Tokyo, Japan). Two independent examiners performed histological evaluation for new bone formation and tissue responses of hydrogel.
2.8
Statistical analysis
Data analyses were done by GraphPad software 7.0 (GraphPad, San Diego, CA, USA). Results are presented as mean ± standard deviation (SD). Statistical analyses were performed by one-way ANOVA. p < 0.05 was considered statistically significant.
3
Results
3.1
Preparation and characterization of CS/GP/GA thermosensitive hydrogel
Results showed that CS/GP/GA mixture could not form a gel and was still fluidity within 300 s when the pH was less than 6 ( Table 2 ). The GT, however, was decreased as the pH increases ( Table 2 ). When the pH was adjusted to 6.5 and 7.0, the GTs were 135 ± 15 s and 110 ± 15 s, respectively ( Table 2 ). For further in vivo experiments, the GT at pH 7.0 looked like suitable for injection because the hydrogel could leak out with long gelation time, and also surgical preparation of MSFA was insufficient with shorter GT. Therefore, CS/GP/GA hydrogel prepared at pH 7.0 was selected to use for all in vitro and in vivo experiments herein.
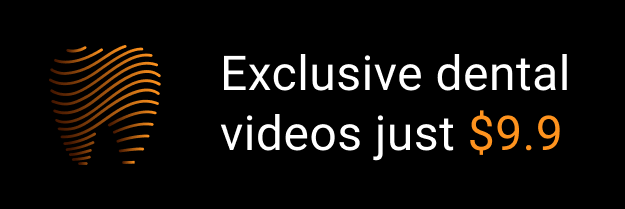