Introduction
The aim of this pilot study was to investigate the effect of long-term bisphosphonate drug use (bone burden) on orthodontic tooth movement in a rat model.
Methods
Sprague Dawley rats were used for orthodontic protraction of the maxillary first molars with nickel-titanium coil springs and temporary anchorage devices as anchorage. Four groups of 5 rats each were included in the study; the first 2 groups were dosed with alendronate or a vehicle during concurrent orthodontic tooth movement. The third and fourth groups were pretreated for 3 months with alendronate or vehicle injections, and bisphosphonate drug treatment was discontinued before orthodontic tooth movement. Tooth movement measurements were obtained at 0, 4, and 8 weeks using high-resolution in-vivo microcomputed tomography, and the tissues were analyzed with histology and dynamic labeling of bone turnover.
Results
Appreciable tooth movement was achieved during the 8-week duration of this study with nickel-titanium coil springs and temporary anchorage devices. Both bisphosphonate treatment groups exhibited reduced tooth movement compared with the vehicle-dosed controls with a tendency toward more severe reduction in the bisphosphonate predosed group. Concurrent dosing of the bisphosphonate drug resulted in 56% and 65% reductions in tooth protraction at the 4-week and 8-week times, respectively. The impact of bisphosphonate bone burden in retarding tooth movement was even greater, with 77% and 86% reductions in tooth movement at 4 and 8 weeks, respectively.
Conclusions
In this study, we used a robust rat model of orthodontic tooth movement with temporary anchorage devices. It has provided evidence that the bone burden of previous bisphosphonate use will significantly inhibit orthodontic tooth movement.
Orthodontic tooth movement occurs through the controlled application of mechanical forces, creating a biologic response on the teeth. The resultant tooth movement is achieved through a myriad of events in the periodontal ligament and the surrounding alveolar bone. Despite the clinical precision of orthodontic mechanics to facilitate the required tooth movement, some pharmacologic agents such as bisphosphonate (BP) drugs commonly used to prevent and treat osteoporosis, a bone disease, can alter orthodontic tooth movement when trapped in bone in sufficient concentrations.
BP drugs are structural nonhydrolyzable analogs of inorganic pyrophosphates and act by selective adsorption to bone mineral surfaces and subsequent inhibition of osteoclasts, the cells responsible for bone dissolution. BP drugs are extensively used for primary osteoporosis in adults along with glucocorticoid-induced secondary osteoporosis, Paget’s disease of bone, hypercalcemia of malignancy, and tumor-induced osteolysis. The nitrogen-containing BP drugs exhibit greater antiresorptive potency because of their ability to interrupt the prenylation of cellular guanidine triphosphatases (GTPases), thus interfering with osteoclast survival and function. Alendronate, a nitrogen-containing BP, is a common antiresorptive drug used for the prevention and treatment of osteoporosis and has been shown to significantly reduce the incidence of new fragility fractures in osteoporotic women. Bone strength is determined by both stiffness (measure of toughness) and flexibility (measure of energy absorption), and an optimal balance between bone quantity and quality is crucial to maintain health. Although BP drugs have been effective in the preservation of bone mass in patients with osteoporosis, recent studies question the effects of their long-term use. They show evidence of poor bone quality with a decrease in energy-absorption capacity compared with normal bone. This sequela predisposes the bone to atypical fractures especially in the femoral neck, lumbar spine, and distal radius. These drugs alter the material properties of the bone along with net changes in the mineral-matrix ratio, thereby leading to an increase in atypical fractures or osteonecrosis of the jaws, particularly in association with high-dose intravenous BP use.
Recent studies investigating the effect of various BP drugs on orthodontic tooth movement have to date been limited to rodent models and used BP drugs either systemically or locally during orthodontic tooth movement. They have shown that BP drugs significantly retard tooth movement in rodent models. In a recent study in rats, alendronate, when administered concurrently with orthodontic tooth movement, inhibited tooth movement by 75% at 2 weeks and by 58% at 4 weeks. Fifty percent of the alendronate that enters the bloodstream after an oral dose is preferentially bound to areas of high bone turnover. Alveolar bone has 10 times more bone turnover than other bones; correspondingly, the accumulation of BP drugs in alveolar bone is significantly higher. The long-term use of BP to treat osteoporosis can lead to substantial accumulation of the drug into bone. That continued accumulation (loading) of the drug over a prolonged period of time burdens the normal bone remodeling process, and we have coined the term “bone burden” to describe that phenomenon. Once incorporated, the BP drug would be released slowly during physiologic bone turnover and to a greater degree during accelerated remodeling processes associated with orthodontic tooth movement. That will most likely have adverse outcomes on bone turnover during attempts to move the tooth roots into BP-laden bone because of the drug’s ability to interfere with osteoclastic activity.
Limited scientific evidence is currently available on the actual consequence of accomplishing orthodontic tooth movements in patients undergoing, or who have undergone, a regimen of BP drug therapy (a clinical scenario of a patient taking long-term BP drugs to treat osteoporosis and interested in orthodontic alignment of his or her dentition). This bone burden effect that can result from BP drugs from previous BP use remaining attached to the bone tissues and being released during the active remodeling process, such as in natural remodeling or orthodontic tooth movement, can have adverse outcomes and has not been investigated. Thus, particularly during bone healing or the linear phase of orthodontic tooth movement, when activated bone cells orchestrate resorption of mineralized bone, cells encounter BP drug molecules that have become trapped in the bone matrix because of their affinity for calcium ions and mineralized tissues. We hypothesized that when this bone burden of incorporated BP drug was released into the local microenvironment, it will interfere with bone cell activity, resulting in cell death and retardation of remodeling, orthodontic tooth movement, or bone healing. The objectives of this animal study were to evaluate and to quantify the inhibition of tooth movement associated with long-term alendronate use.
Material and methods
For the experimental protocol and the design of the orthodontic tooth movement appliance, 20 female Sprague Dawley rats (age, 12 weeks) were randomly assigned to 4 cohort groups (5 per group): (1) control vehicle (saline solution) concurrent dosed, (2) BP concurrent dosed (0.015 mg/kg subcutaneously dosed concurrently twice weekly during orthodontic tooth movement for 8 weeks), (3) control vehicle (saline solution) predosed for 12 weeks before and after orthodontic tooth movement for 8 weeks, and (4) BP predosed (0.015 mg/kg subcutaneously predosed for 12 weeks before and after orthodontic tooth movement for 8 weeks). This antiresorptive twice-weekly subcutaneous dosing schedule for the BP drug alendronate has previously been used successfully in rats. The 12-week predosing interval was chosen to mimic an effective dose of BP capable of blocking bone resorption over the 12-week period.
The control group had the same dosing schedule but with a saline-solution vehicle instead of the active drug. The experimental design and dosing schedule are shown in Figure 1 .
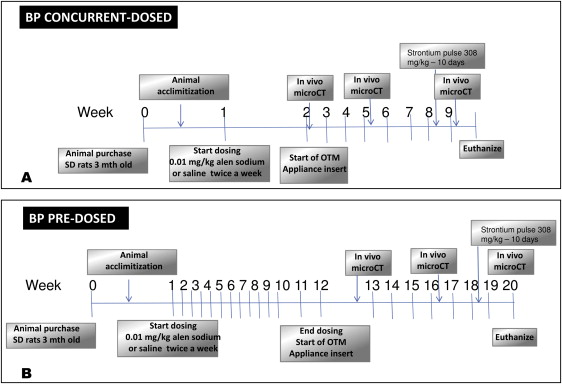
Ethics approval was obtained from the University of Alberta animal care and use committee. The animals were purchased from university biosciences and housed with 12-hour dark-and-light cycles. All animals were fed a standard laboratory soft diet ad libitum. To insert the temporary anchorage devices and the coil springs, the rats were anesthetized using 2% isoflurane/L oxygen (Forane; Baxter, Deerfield, Ill) and placed supinely in a custom-designed surgical jig that maintained the rat’s obligate nasal inhalation of the anesthetic, while permitting retraction of the mandible to expose the maxilla and the palate. A small 4-mm semilunar incision was made from the distopalatal gingival margin of the maxillary right incisor posteriorly with a number 15 surgical blade. After achieving adequate hemostasis, a pilot hole was drilled in the maxillary bone at a 45° angle using a .5-mm round bur attached to a slow-speed electric dental hand piece (NSK; Brassler, Savannah, Ga). A self-threading 1.2 × 3 mm titanium temporary anchorage device (Stryker-Leibinger, Hamilton, Ontario, Canada) was inserted to a depth of 1.5 mm into the alveolar bone about 12 to 14 mm from the mesial aspect of the right permanent first molar. The fit and stability of the temporary anchorage device into the alveolar bone were verified by finger pressure, with a side-to-side and in-and-out motions. A stainless steel ligature wire was placed around the neck of the maxillary right permanent first molar and secured in position by tightening. A 9-mm closed-coil nickel-titanium spring (GAC International, Bohemia, NY) was secured to the right permanent first molar and the implant neck anteriorly with a 0.010-in stainless steel ligature. The appliance was left in place for 8 weeks to achieve appreciable tooth movement, as measured by microcomputed tomography imaging (described below). The left side acted as an intra-animal control with no appliance. The surgical temporary anchorage device placement and appliance insertion are shown in Figure 2 .
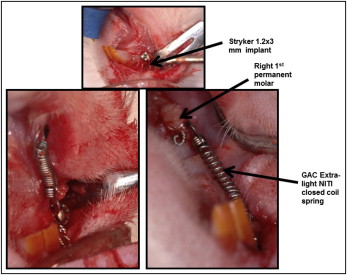
We measured the force displacement. Nickel-titanium closed-coil springs (stated by the manufacturer GAC International to exert 50 g of force) were tested for force levels at different displacements using a universal testing machine with a load cell capacity of ± 1 kN (model 4443; Instron, Norwood, Mass). Briefly, an acrylic resin attached to a hook was glued on the lower platen, and the GAC light nickel-titanium closed-coil spring was attached from the hook to the upper loading crosshead. Loading and unloading curves were generated by applying a constant displacement of 2.5 mm per minute up to 12 mm of displacement.
In-vivo microcomputed tomography imaging (SkyScan 1076; SkyScan NV, Kontich, Belgium) was performed on all animals at baseline (0 weeks), and after 4 and 8 weeks of orthodontic tooth movement. All scans were conducted at 100 kV and 100 μA through 180° with a rotation step of 0.5° to produce serial projectional images of isotropic 18-μm 3 voxels. All images were processed with bundled commercial software. The acquired data were Gaussian filtered and underwent global thresholding to extract the mineralized phase representing the 3-dimensional bone architecture. Visualization of the 3-dimensional architecture was generated to provide a qualitative assessment of bone structure. Measurements were made between the maxillary right first and second molars at all time points.
Briefly, microcomputed tomography-rendered 2-dimensional scans were reconstructed as .bmp files and viewed using Data Viewer software (SkyScan NV). The 2-dimensional slices displayed as 3 orthogonal sections in the x, y, and z planes of space were centered at the desired point inside the reconstructed space. Once the image was centered in all 3 planes, the linear distance from the most convex contact area between the maxillary right first and second molars was measured and recorded. Measurements were recorded at 0, 4, and 8 weeks, and the amounts of tooth movement were obtained by subtracting the distances at 4 and 8 weeks from the baseline reading. Measurements were made by the primary author (N.K.) in a blinded fashion and repeated for reliability (r = 0.93) ( Fig 3 ).
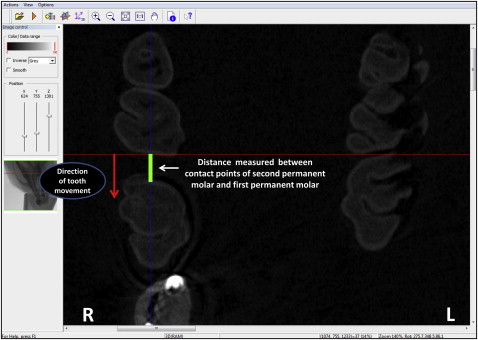
At the experimental end point (8 weeks), all animals were killed using isoflurane and carbon dioxide to effect, and the right and left maxillae were dissected, stored in 4% paraformaldehyde (Sigma-Aldrich Canada, Oakville, Ontario, Canada), and fixed for 1 week with frequent changes. After fixation, each hemi-maxilla was cut sagittally at the level of the permanent first molar. The palatal half was processed for the spatial mapping of bone turnover using electron-probe microanalysis, with the buccal half processed for routine histology. All samples processed for histology were rinsed and immersed in 4.13% EDTA (ethylenediaminetetraacetic acid; Sigma-Aldrich Canada) decalcifying solution for 3 weeks. Following decalcification, the samples were embedded in paraffin, and sagittal sections (6 μm) were cut and stained with hematoxylin and eosin for routine histologic examinations by light microscopy.
All animals were given a brief 10-day pulse of elemental strontium (strontium ranelate, PROTOS; Servier Laboratories, Hawthorn, Victoria, Australia; 308 mg/kg/day body weight) by gavage before they were killed. We have previously shown that subtherapeutic levels of elemental strontium will serve as an exquisite dynamic tracer for bone turnover capable of detection by electron probe microanalyzer (SX-100; CAMECA Instruments, Madison, Wis) at high resolution. Electron-probe microanalysis was performed on the palatal half of the right permanent first molar for spatially mapping the location and distribution of elemental strontium. Briefly, the first molar’s sagittal section was defatted in acetone, embedded in epoxy resin, progressively polished (about 0.5 μm), and scanned to analyze the calcium, strontium, and phosphorus content in both BP-dosed groups and the controls.
Statistical analysis
The pilot data (5 per group) were not assumed to be normally distributed because of the small sample size, and a nonparametric approach was used for statistical analysis. To compare the mean amounts of tooth movement at 4 and 8 weeks among the groups, a Kruskal-Wallis H nonparametric test was performed with the significance level set at 95% (α = 0.5). The hypotheses of interest were whether tooth movement was the same regardless of drug use; whether mean amounts of tooth movement are the same among the groups at 4 and 8 weeks; and whether mean amounts of tooth movement are different among the groups at 4 and 8 weeks.
SPSS statistical software (version 16.0; SPSS, Chicago, Ill) was used to analyze the data. Orthodontic tooth movement measurements, weight changes in the animals, and force level changes of the nickel-titanium closed-coil springs from the age-matched cohorts were used for statistical analyses. All quantitative data were expressed as means ± standard deviations. A value of P <0.05 was considered statistically significant. The Mann-Whitney U test was performed for comparisons between groups with the P value set at α = 0.016.
Results
None of the animals encountered postoperative complications or infections, although some food impaction was observed around the implant sites. All animals remained healthy and survived the experimental process without any statistically significant loss of weight in any group at 4 ( P = 0.262) and 8 ( P = 0.1) weeks. Four animals (2 in the BP concurrent dosed and 2 in the control concurrent dosed groups) exhibited spontaneous appliance loosening necessitating removal of the implant and removal of those animals from the study.
Figure 4 shows the loading and unloading curves of all the nickel-titanium springs used in the study. There was no difference in the mean force levels at 0 weeks between the controls (29.5 ± 7.4 g) and the BP-dosed rats (30.6 ± 3.1 g). In addition, the experimental animal model showed no statistically significant changes in the force levels among the groups at 4 ( P = 0.76) and 8 ( P = 0.918) weeks.
Descriptive statistics of tooth movements between the groups at the different time points are presented in the Table . The difference in tooth protraction between the control and the BP-dosed groups was evident qualitatively upon examination of the 3-dimensional microcomputed tomography renderings at 0, 4, and 8 weeks at the coronal level ( Fig 5 ). Nonparametric analysis of variance showed statistically significant differences among all 4 groups at 4 weeks ( P = 0.008) and 8 weeks ( P = 0.008). In terms of measured distances, the results showed statistically significant reductions in tooth movement of 0.51 ± 0.1 mm (77% reduction) and 1.7 ± 0.1 mm (86% reduction) at 4 ( P = 0.009) and 8 ( P = 0.009) weeks, respectively, between the BP predosed and the control animals. Because the significance level was set at α = 0.016, the BP concurrent dosed group showed a nonsignificant reduction in tooth movement compared with its respective control at 4 ( P = 0.05) and 8 ( P = 0.05) weeks, and the amounts of reduction were only 0.48 ± 0.1 mm (56%) and 1.2 ± 0.2 mm (65%) at 4 and 8 weeks, respectively, as shown in Figure 6 . There was no significant difference between the treatment groups (BP predosed group and BP concurrent dosed group) at 4 ( P = 0.053) and 8 ( P = 0.297) weeks.
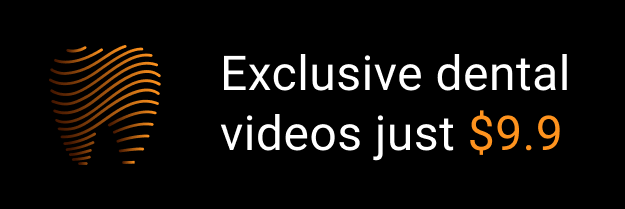