Growth and development
Publisher Summary
This chapter describes the process of growth and development in a living organism. The processes by which a fertilized ovum develops finally into an adult human being are still not completely understood. The most obvious way to assess or express development is by age. However, chronological age is not necessarily related to either physical or mental development. Tissues may grow by an increase in the number of cells or an increase in the size of cells or both. In the nonregenerative tissues, growth occurs early in life. The best correlation between the various measures of development is that between bone age and the age of the menarche in girls, but bone age does not correlate well with the age at which puberty begins in either sex. The changes that take place as a result of the growth spurt are in some instances similar in the two sexes, in others different. The critical factor is usually the presence or absence of testosterone. The main change that can definitively be described is an increase in the cross-linking and rigidity of collagen fibers.
The processes by which a fertilized ovum develops finally into an adult human being are still not completely understood. Although measurements of hormone concentrations in blood and tissues are yielding more information about the stimuli for various developmental changes, there is virtually no information on how the time scale and sequence of development and growth are programmed and realised. The factors which govern organ size and shape are still poorly identified. Molecules known as organisers probably diffuse between cells to control differential growth and cell membranes probably interact with each other in delineating tissue boundaries – but no organiser molecules have yet been isolated and the mechanisms of membrane interaction are obscure.
Measures of growth and development
Size, or height, is not a particularly reliable indicator of development but is used. As mentioned above, it is one of the principal ways of determining the age, or developmental age, of the fetus. The crown-rump length or the crown-sole length can be measured on X-ray pictures, and ultrasonic scanning can be used to measure head size, crown-rump length and abdominal circumference.
Other measures of development are available. The growth of the legs and feet is used as an indicator between birth and 18 months; after that the hand and wrist may be X-rayed to reveal the development of the individual bones which calcify in a specific sequence (Fig. 23.1). The mean ages at which the different stages are observed are termed bone ages. Growth in the female is always some 25% ahead of that in the male during the first ten years of life, and so female bone age is ahead of male bone age at the same chronological age.
Tissue growth
In the non-regenerative tissues growth occurs early in life. There are three phases: one of cell division, one of cell growth associated with a slower rate of cell division, and a final stage of cell growth only. Nerve cells stop dividing at about 18 weeks i.u. life and no new cell nuclei appear thereafter. The axons and dendrites can grow, and damage to one of these cell extensions is repaired by outward growth from the cell body. The supporting cells of the nervous system, the glial cells, appear to be capable of division throughout life. The growth and maintenance of sympathetic nerves is dependent upon nerve growth factor, a peptide produced in many tissues including the salivary glands, which is taken up by the axons and transported back to the soma, or body of the cell. Muscle cell numbers are also established at an early age, probably soon after 30 weeks i.u. life. Developing skeletal muscle is a tissue in which cells fuse together during embryonic development so that the final cells have many nuclei. Some cells remain as reserve, or satellite, cells: these are mononuclear with very little cytoplasm around the nucleus. Growth of muscle cells occurs by the synthesis of new protein to increase the contractile protein content of each cell. Muscle growth in response to the stimulus of increased usage involves incorporation of some satellite cell nuclei into the existing fibres in addition to the increase in protein content. Since growth occurs by increased protein synthesis, it is dependent upon the anabolic hormones, testosterone, adrenal androgens, and growth hormone. Insulin is also anabolic in its effects. The third tissue in which the number of cells is determined before birth is adipose tissue. Like the cells of skeletal muscle, fat cells increase the size of the tissue by increasing the volume of their cell contents: in this case by storing fat. Loss of stored fat from within the cells decreases the tissue size, just as decrease in the actin and myosin content of disused skeletal muscle leads to wasting of the muscle. It is probable that in adipose tissue, again as in muscle, there are less well-differentiated cells which will not develop into mature adipocytes unless excessive fat deposition occurs. Some writers dispute whether fat cells are non-regenerative and believe that new cells can be produced in these conditions. The adipose tissue of the fetus includes a relatively large proportion of brown adipose tissue, whose forming cells resemble those of the more usual white adipose tissue but whose mature cells differ from them both in appearance and function. All the non-regenerative tissues increase in size by hypertrophy.
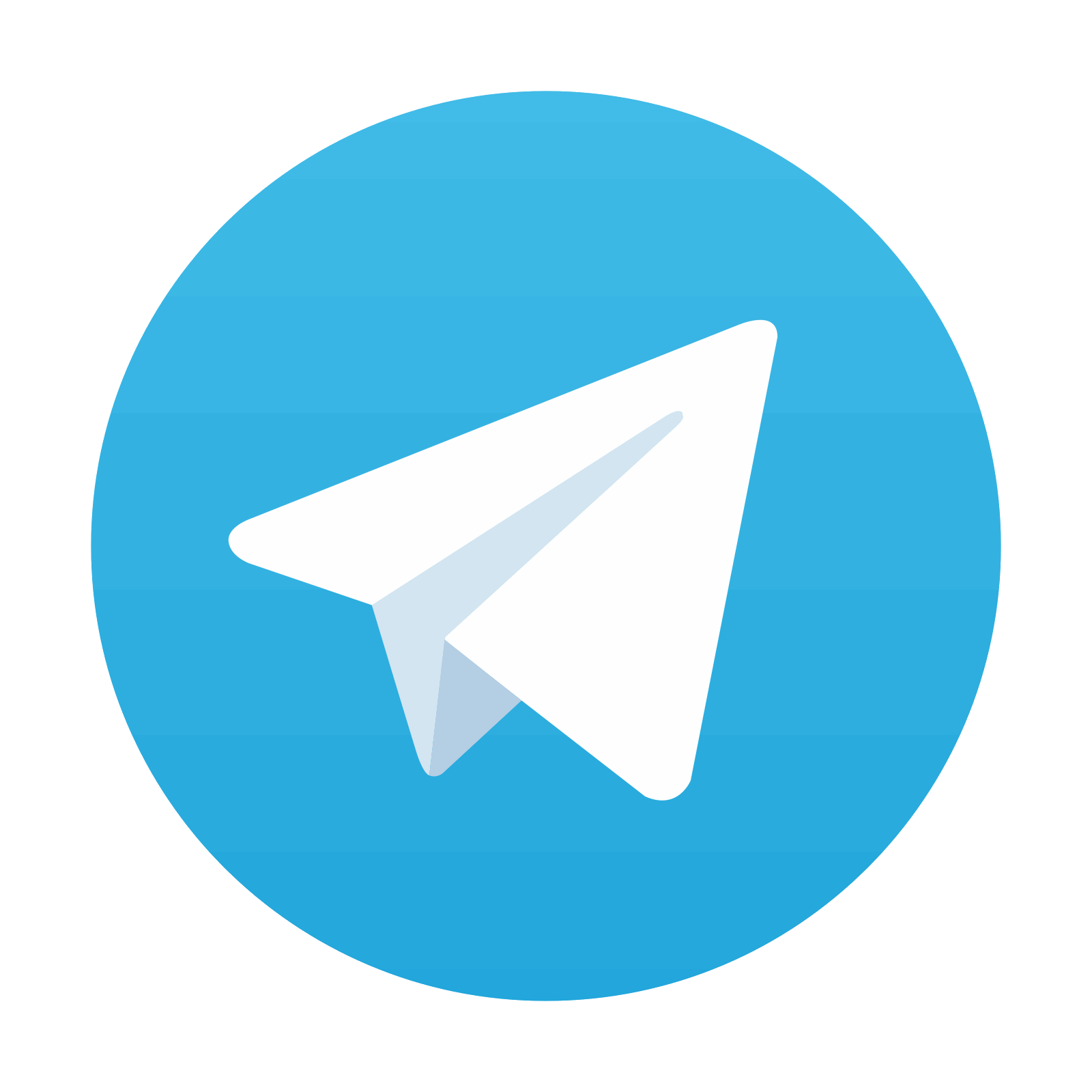
Stay updated, free dental videos. Join our Telegram channel

VIDEdental - Online dental courses
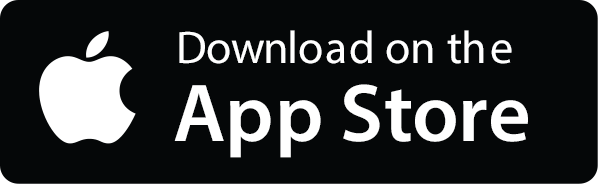
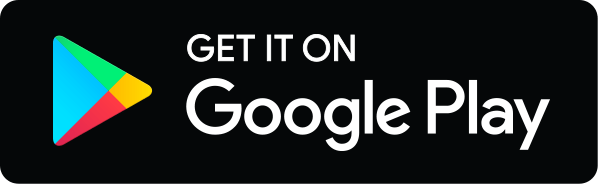