Introduction
The exact force systems as well as their progressions generated by removable thermoplastic appliances have not been investigated. Thus, the purposes of this experimental study were to quantify the forces and moments delivered by a single aligner and a series of aligners (Invisalign; Align Technology, Santa Clara, Calif) and to investigate the influence of attachments and power ridges on the force transfer.
Methods
We studied 970 aligners of the Invisalign system (60 series of aligners). The aligners came from 30 consecutive patients, of which 3 tooth movements (incisor torque, premolar derotation, molar distalization) with 20 movements each were analyzed. The 3 movement groups were subdivided so that 10 movements were supported with an attachment and 10 were not. The patients’ ClinCheck (Align Technology, Santa Clara, Calif) was planned so that the movements to be investigated were performed in isolation in the respective quadrant. Resin replicas of the patients’ intraoral situation before the start of the investigated movement were taken and mounted in a biomechanical measurement system. An aligner was put on the model, the force systems were measured, and the calculated movements were experimentally performed until no further forces or moments were generated. Subsequently, the next aligners were installed, and the measurements were repeated.
Results
The initial mean moments were about 7.3 N·mm for maxillary incisor torque and about 1.0 N for distalization. Significant differences in the generated moments were measured in the premolar derotation group, whether they were supported with an attachment (8.8 N·mm) or not (1.2 N·mm). All measurements showed an exponential force change.
Conclusions
Apart from a few maximal initial force systems, the forces and moments generated by aligners of the Invisalign system are within the range of orthodontic forces. The force change is exponential while a patient is wearing removable thermoplastic appliances.
The Invisalign system, introduced by Align Technology (Santa Clara, Calif) in 1999, combines the basic principles of Kesling’s, Ponitz’s, McNamara’s, and Sheridan’s orthodontic treatment with removable thermoplastic appliances (RTAs; appliances made from transparent plastic material such as polyurethane) with modern CAD-CAM stereolithography and tooth movement simulation software. Since it is a relatively new method, some aspects are still insufficiently investigated. Most previous studies on RTAs are case reports and system descriptions. Furthermore, material science studies have evaluated the changes in force delivery properties of RTAs after thermocycling, as well as the chemical and morphologic changes after usage.
Forces and moments generated by RTAs have barely been investigated.
In 3 follow-up in-vitro studies, Hahn et al quantified the forces for 3 tooth movements (rotation, tipping, and torque of a maxillary central incisor) generated by 3 thermoplastic materials (Ideal Clear [Dentsply GAC, Gräfelfing, Germany], Erkodur [Erkodent Erich Kopp GmbH, Pfalzgrafenweiler, Germany], and Biolon [Dreve Dentamid GmbH, Unna, Germany]). They all had a thickness of 1.0 mm but differed in the thermoforming process. Regarding their results, it seems that pressure-formed appliances exert greater force systems at higher rates of activation compared with thermoplastic vacuum-formed appliances. However, the results were statistically significant only in some cases.
In an in-vivo study, Barbagallo et al investigated the force transmitted by aligners (synonym for RTAs) of the ClearSmile system (Clear Smile appliance, Woollongong, Australia) using Pressurex films (Fuji Photo Film Co, Ltd, Tokyo, Japan). The films, placed under the aligners, recorded the overall mean forces. The measurements were performed on the first day and after 2 weeks—the last day of wearing the aligner. They found a high initial mean force on the first day and a low final force, and concluded that the force curve is not linear but, rather, exponential. Their results agree with the findings of Vardimon et al, who indirectly evaluated the generated forces of aligners of the Invisalign system. Assuming that forces generated by aligners behave like the von Mises strains developed in an aligner, they bonded strain gauge rosettes on aligner surfaces and measured in-vivo strains during Invisalign treatment. Their results showed a peak strain on the first day followed by a plateau phase at a lower force level between days 2 and 15.
The main disadvantages of these in-vivo studies are that the data only show an overall mean force on a specific position on the crown at a certain time. The exact force systems in all 3 planes of space, as well as the exact distribution of the forces and moments, have not been detected so far, but they are important to know when planning orthodontic treatment with an aligner system.
Thus, the aims of this experimental study were (1) to quantify the exact initial force systems that are delivered by an individual aligner, (2) to measure the force systems generated by a series of aligners, and (3) to investigate the influence of auxiliaries (attachments, power ridges) on the force transfer.
These aims were achieved by aligners of the Invisalign system for 3 predefined tooth movements, since these are the movements described as impossible or difficult to perform with RTAs: incisor torque, premolar derotation, and molar distalization.
Material and methods
In this study, we investigated the initial force systems delivered by 970 individual aligners and 60 series of aligners (mean aligners per series, 16.7; SD, 5.0). These aligners came from 30 consecutive patients (11 male, 19 female; ages, 13-72 years; mean age, 32.9 years; SD, 16.3 years) who underwent orthodontic treatment in 2011 and 2012 in a private orthodontic practice in Cologne, Germany. Our inclusion criteria for the patients were orthodontic treatment with Invisalign aligners and a need for 1 of these 3 movements: incisor torque, premolar derotation, or molar distalization. Exclusion criteria were cleft lip and palate or any other syndrome-associated orofacial malformation.
According to the investigated tooth movement, the 30 patients were divided into 3 main movement categories with 10 patients in each group. In each movement category, 20 tooth movements (2 per patient) were determined. To investigate the influence of auxiliaries such as attachments (temporarily bonded composite buttons) and power ridges (pressure lines close to the gingival margin), the 3 main movement categories were split into 2 subgroups using a split-mouth design ( Fig 1 ).
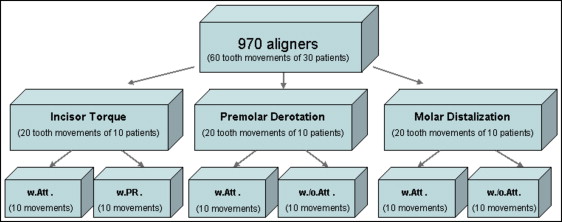
In group 1, maxillary central incisor torque was greater than 10°, supported by a “horizontal ellipsoid attachment” or power ridges.
In group 2, premolar derotation was greater than 10°, supported by an “optimized rotation attachment” or with no auxiliary.
In group 3, molar distalization was greater than 1.5 mm, supported by a 4-mm-long “horizontal beveled gingival attachment” or with no auxiliary.
The force systems generated by the series of 60 aligners for 60 tooth movements from 30 patients (2 tooth movements per patient, 20 tooth movements in each main group, and 10 tooth movements in each subgroup) were determined.
All the attachments we used were engineered by Align Technology to achieve predictable tooth movements. The vertical and transverse positions of the attachments on the tooth surfaces were done according to Align Technology’s treatment protocol (for incisor torque and molar distalization) or automatically by the software (for premolar derotation). The allocations of the teeth to the subgroups with or without an auxiliary were randomized.
Using ClinCheck (Align Technology, Santa Clara, Calif), the treatment was planned so that the tooth movement to be investigated was performed in isolation at the beginning of the treatment to make it clearly distinguishable from any other tooth movement.
The experimental investigation of the force systems generated by the aligners was performed at the University of Bonn in Germany. Alginate impressions (Tetrachrom Alginat; Kaniedenta, Herford, Germany) of each patient’s intraoral situation before tooth movement were taken, and plaster cast models were produced (Snow White Plaster; Kerr, Karlsruhe, Germany). Thereafter, resin replicas (Palavit G; Heraeus Kulzer, Hanau, Germany) of the pretreatment plaster cast models were produced, and the tooth to be analyzed was removed from the replica. Both the resin replica of the whole arch and the tooth to be moved were mounted into the orthodontic measurement and simulation system ( Fig 2 ). This is a custom-made system developed for orthodontic biomechanical investigations. More details of the technical specifications and the software running the experiments can be found in several articles. The measuring system consists of 2 force and moment sensors that can measure force and moment vectors in all 3 dimensions (x, y, and z). Sensors are mounted on a motor-driven 6-axis positioning table that can perform full 3-dimensional movements. The removed tooth was connected to 1 sensor, and the dental arch was mounted in the orthodontic measurement and simulation system. Adjustments were made with a passive aligner of the initial stage so that the tooth under investigation was in its initial position in the dental arch, and no active force system was transferred to the tooth. Subsequently, a duplicate of the clinically used aligner series was placed on the model, the removed tooth was deflected, and the forces and moments generated by the aligner were measured by the orthodontic measurement and simulation system sensor.
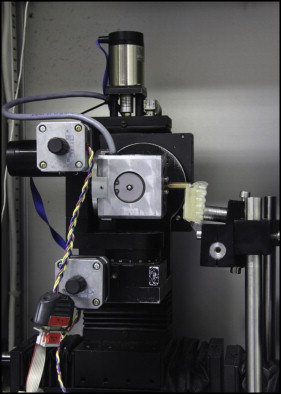
Based on the force system, the control program of the orthodontic measurement and simulation system calculated the developed tooth movement using a mathematical model, taking into consideration the center of resistance of the tooth. According to the literature, this is defined as one-third of the root length from the alveolar crest to the apex and at the furcation for the maxillary molars.
Tooth movement was implemented in increments and transformed by the stepping motors of the positioning tables. Depending on the vector of movement, the absolute value of each movement increment was below 0.1 mm. Single components were typically even close to zero (<0.01 mm). By means of continuous measurements of the force systems and simulation of the resulting tooth movement, the force progression generated by an aligner was measured, and the experimentally resulting tooth movement was depicted. For all 3 types of tooth movements, the measurements were terminated when forces dropped below 0.2 N (1 N = 1/9.81 kg = 102 g). Then the next aligner of the series was used. On average, 17 aligners were used to perform each tooth movement. Sixty tooth movements from 30 patients were investigated, so that 970 aligners were investigated in this study. To compensate for interlaboratory measuring inaccuracies, the measurements were repeated at least 3 times; overall, 2910 measurements were performed.
To describe the tooth movements in all 3 spatial dimensions, a coordinate system was set up: the positive x-axis describes extrusive and the negative x-axis describes intrusive forces and movements, parallel to the long axis of the tooth. Horizontal forces and movements are described in the y-axis and the z-axis. In respect to the position of the tooth in the dental arch, the y-axis and the z-axis describe different movements for a molar and an incisor. For a molar, the positive y-axis describes buccal movements, the negative y-axis describes palatinal and lingual forces and movements, the positive z-axis describes mesial movements, and the negative z-axis describes distal forces and movements. For a maxillary incisor, the positive y-axis describes distal movements, the negative y-axis describes mesial forces and movements, the positive z-axis describes buccal movements, and the negative z-axis describes lingual forces and movements ( Fig 3 ). Thus, the biomechanical measured force systems could be transferred exactly to the generated tooth movement.
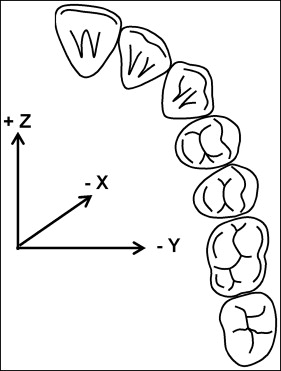
Statistical analysis
In this study, we investigated the force systems of 970 Invisalign aligners from 30 consecutively recruited patients. The aligners were produced to perform 3 predefined tooth movements: incisor torque, premolar derotation, and molar distalization. In all, 60 tooth movements (2 movements in each patient, 20 movements in each group) were determined. A split-mouth design was used to investigate the influence of auxiliaries (attachments and power ridges) on the force transfer. Therefore, the 3 main groups were divided into 2 subgroups (with an attachment, and with no attachment or with a power ridge), to which the teeth were allocated randomly.
The analysis included a comparison of the initial force systems that were delivered by an individual aligner and by a series of aligners. The statistical evaluation included the analysis of the measured force systems as well as minimums, maximums, means, and standard deviations of the means. Since the results were normally distributed according to a chi-square test, statistical significance was performed using the t test with a significance level of P ≤0.05.
Results
Figure 4 shows an example of a typical maxillary incisor torque measurement of 15 aligners with the measured forces ( Fig 4 , A ), the corresponding translational movements ( Fig 4 , B ), the measured moments ( Fig 4 , C ), and the resulting rotational movements ( Fig 4 , D ) each in all 3 axes. The data clearly demonstrate that each aligner creates high initial forces, followed by an exponential decrease to forces or moments just above 0 N/N·mm. Furthermore, the data demonstrate that the forces and moments between consecutive aligners differ, even though a continuous movement was planned in ClinCheck.
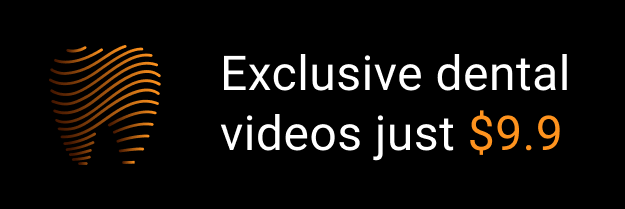