Fig. 1
Drawing of a section of the human eye. Image courtesy of the National Eye Institute, National Institutes of Health
This chapter reviews ongoing research involved in assessing the potential of dental stem cells for regenerative ocular therapy. Several populations of adult stem cells have be identified in dental tissues, and due to their neural crest developmental origins, maybe be advantageous for use in ocular cellular therapy. These populations of stem cells include those isolated from the dental pulp of adult of exfoliated deciduous teeth, or periodontal ligament. Portions of the cornea are derived from the cranial neural crest; therefore due to their similarity in developmental origins, dental stem cells may potentially possess a stronger affinity to differentiate towards corneal cell lineages. Additionally, the neural crest gives rise to the neurons of the peripheral nervous system, so dental stem cells may have the capacity to differentiate into neurons for replacing damaged cells of the retina. An additional benefit of dental stem cells is their accessibility. These cells can be easily collected from patients for autologous use. These attributes make dental stem cells a potential viable option for developing cellular ocular therapies.
Dental Stem Cells to Treat Corneal Blindness
The cornea is the anterior most tissue of the eye that serves multiple functions such as allowing the passage of light with minimal scatter, acting as a barrier to prevent penetration or infiltration into ocular tissues, and providing two-thirds of the refractive power of the eye to focus light onto the retina [1]. The complex structure of the cornea allows it to facilitate these multiple functions. The cornea comprises three cellular layers: the epithelium is the anterior-most, the stroma is central, and the posterior-most layer is the endothelium. Millions of individuals worldwide suffer from bilateral corneal blindness due to diseases associated with these cellular layers or trauma to the cornea [2]. Stem cell research shows promising results for developing cellular therapies to restore vision to patients suffering from corneal disorders.
Corneal Epithelium
The anterior surface of the cornea comprises a stratified squamous epithelium that acts as a barrier to the eye. The corneal epithelium is a self-renewing structure where the superficial terminal cells are naturally shed and repopulated by a population of stem cells found in the limbus, the transition zone between the cornea and the sclera, termed limbal epithelial stem cells [3]. In addition to functioning in the natural process of epithelial self-renewal, these stem cells are capable of regenerating the entire corneal epithelium following injury.
Disease or injury leading to total limbal stem cell deficiency (LSCD) results in the inability of the corneal epithelium to renew and regenerate itself and therefore leads to corneal opacity due to corneal inflammation and vascular infiltration. Unilateral LSCD is currently treated by transplanting limbal epithelial cells from the contralateral eye [4]. However, treating bilaterial LSCD requires allogeneic tissue transplantation, which is subject to failure due to immunological rejection [5]. Therefore the development of autologous stem cell therapies utilizing cells from an alternative tissue source to repopulate the limbal epithelial stem cells would bypass limitations of current treatments.
Stem cells from exfoliated deciduous teeth (SHED) are a highly investigated population of stem cells since they can be easily isolated from autologous tissues and have the capacity to differentiate into multiple cell types [6]. Recent studies have shown promising results on the ability of SHED to regenerate corneal epithelial cells. Epithelial and stem cells gene expression profiles of SHED were shown to be more similar to that of limbal epithelial stem cells than differentiated corneal epithelial cells for genes such as ABCG2, K12, and K3 [7]. SHED were able to restore corneal epithelial tissue in an LCSD animal model in vivo. LSCD was induced in New Zealand white rabbits through chemical burning. One month following injury, keratectomy was performed to remove pannus formed from the chemical burn, and cell sheets comprising SHED were transplanted onto the exposed rabbit stroma. To prevent damage to the SHED sheet, acellular human amniotic membrane was placed over the cell sheet and sutured to the episclera. Without intervention, the chemical assault to rabbit corneas resulted in corneal vascularization, conjunctivalization, and opacification. Histological analysis of untreated eyes lacked the presence of a stratified epithelium and confirmed the presence of a disorganized, neovascularized conjunctival tissue [7, 8]. Alternatively, the introduction of sheets of SHED to chemically injured rabbit eyes led to clearer corneas with less neovascularization than the control eyes. Furthermore, histological analysis showed that injured eyes receiving SHED had a regenerated corneal epithelium containing cuboidal basal cells, intermediate flattened cells, and polygonal surface cells, similar to nature corneal epithelial tissue [3, 7, 8]. These studies provide promising results for the potential use of SHED in cellular or tissue engineering therapies to regenerate corneal epithelial tissue .
Corneal Stroma
The corneal stroma is the central-most tissue of the cornea and makes up the bulk of the cornea comprising about 90 % of its structure [9]. The stroma has a complex, lamellar structure that facilitates its mechanical integrity and transparency. Each stromal lamella contains long fibrils of collagen that are organized in parallel, have approximately uniform diameter, and are uniformly spaced. The direction of collagen fibril orientation rotates orthogonally between adjacent lamellae [10]. The lattice structure formed by these tightly packed collagen fibrils minimizes light scatter facilitating the transparency of the cornea [11]. Keratocytes are the cells that maintain the stroma. These are quiescent cells serve multiple functions including generating specific molecules that regulate collagen fiber spacing to maintain corneal transparency.
Trauma or disease can cause disruptions to the collagen organization within the corneal stroma diminishing its transparency. Keratocytes respond to stromal trauma by differentiating into fibroblasts and depositing an unorganized scar tissue . This scar tissue does not have the highly organized collagen structure characteristic of healthy stromal tissue and is consequently opaque [12]. Millions of individuals suffer from bilateral corneal blindness resulting from the formation of corneal scars [2]. The current method of treatment is a keratoplasty , which involves using cadaveric grafts to replace the damaged tissues. However, there is a worldwide shortage of donor tissue and this treatment has a failure rate of 38 % after 10 years due in part to immune rejection [13].
The development of a cellular therapy or engineered tissues using autologous stem cells to treat stromal scarring could bypass the limitations associated with current allogeneic grafting treatment. Researchers have started investigating adult stem cell populations such as those derived from adipose tissue or corneal stromal tissue [14–16]. Dental pulp isolated from adult teeth contains a population of multipotent stem cells that are easily accessible and may have a higher affinity for corneal stromal differentiation since, similar to the corneal stroma, they are neural crest-derived.
Human dental pulp stem cells (DPSCs) isolated from adult third molar cells were shown to be able to differentiate into keratocytes in vitro and maintain this phenotype in vivo [17]. The DPSCs were cultured as pellets in a keratocyte differentiation medium, in a similar manner as the culture treatment used to induce keratocyte differentiation from corneal stromal stem cells [18]. After in vitro differentiation, DPSCs had increased expression of molecules characteristic of keratocytes at both the gene and protein levels [17]. Of particular significance was the upregulation in the expression of the proteoglycan keratocan. This molecule is critical in maintaining proper collagen fibril spacing required for corneal transparency. Importantly, keratocan is only found in the corneal stroma and is therefore considered a keratocyte specific marker [19]. The expression of keratocan at the gene and protein levels verifies that DPSCs differentiated into keratocytes in vitro.
DPSCs differentiated into keratocytes in vitro were injected into mouse corneal stroma to assess the behavior of the cells in the in vivo environment. The DPSCs remained in the mouse stroma for up to 5 weeks and produced corneal stromal matrix molecules such as type I collagen and keratocan in vivo. Furthermore, the mouse stroma remained transparent with the injected DPSCs indicating that the DPSCs were not inducing any adverse reaction vivo [17].
The ability of DPSCs to generate tissue engineered corneal stromal constructs with similar structure as native tissues was assessed. DPSCs were cultured on aligned nanofibers to direct cell and matrix organization. In these engineered tissues, DPSCs aligned in parallel and produced a parallel-aligned collagenous matrix, similar to native corneal stromal tissue. Furthermore, the direction of collagen fibrils rotated at different depths within engineered tissue indicating the formation of multiple lamellae. The collagen fibrils were approximately uniform in diameter similar to engineered tissues generated by CSSC [17]. This study showed that DPSCs can differentiation in keratocytes in vitro, maintain this phenotype in vivo, and be used to generate engineered corneal stromal tissue. This shows promise for the development of DPSC-based cellular or tissue engineering therapies to treat corneal stromal scarring .
Corneal Endothelium
The corneal endothelium is the posterior most cellular layer of the cornea. It is a single layer of neural crest derived cells that function to regulate hydration of the stroma through barrier and pump functions [9]. Correct water content is critical in controlling the stromal collagen fibril spacing needed to maintain corneal transparency. Therefore, hydration defects such as edema cause increased light scatter resulting in corneal haze [1]. Corneal endothelial cells have limited proliferative capacity and severe decreases in cellularity due to trauma or diseases such as Fuch’s dystrophy results in an edematous cornea with reduced transparency [20]. More than half of corneal transplants occur due to damage or disease to the corneal endothelium [21, 22] indicating a need for the development of cellular therapies . A recent study showed that neural crest derived stem cells isolated from the corneal stroma maintain the capacity to differentiate into corneal endothelial cells [23]. However, few, if any, additional studies have identified alternative adult stem cell populations with this ability. Currently, there are no studies reporting the differentiation competence of dental stem cells into corneal endothelial cells. The capacity of dental stem cells to differentiate into corneal endothelial cells seems plausible since both tissues share similar developmental origins. Potentially, the methods developed to induce corneal endothelial cells from corneal stromal stem cells could be adapted to dental cells. This is still an open scientific question that needs to be answered since, if possible, the use of dental stem cells to treat disorders of the corneal endothelium would greatly impact the field of corneal regeneration.
Dental Stem Cells to Treat Retinal Degeneration
The retina is a complex tissue in the posterior region of the eye involved in converting light into visual signal and transmitting it to the brain. This process is orchestrated by multiple, specialized neuronal cells organized into nuclear layers. The photoreceptor cells, the rods and cones, are located in the outer nuclear layer and are the light-sensitive cells that first detect light and initiate the process of translating it into visual signal. The signal is then processed by neurons in the inner nuclear layer, including horizontal cells, bipolar cells, and amacrine cells. Finally, the signal is received by the retinal ganglion cells (RGCs) , which transmit the signal to the brain. The RGC somas are positioned in the ganglion cell layer and their axons stretch towards to posterior of the eye, along the surface of the retina in the retinal nerve fiber layer. The RGC axons collect at the optic nerve head, and assemble to form the optic nerve [24, 25].
Retinal degeneration due to genetic disease or age-related disorders can lead to retinal cell damage or death resulting in vision impairment or loss. Common genetic disorders affecting retinal function result from mutations of the gene for rhodopsin, the light-sensitive protein expressed by the photoreceptor cells [24]. Additional types of retinopathies include glaucoma , a group of diseases resulting from optic nerve degeneration due to RGC loss or damage. Over 60 million individuals worldwide suffer from glaucoma, and this disease has caused bilateral blindness in approximately eight million of these people [26]. Glaucoma is the second leading cause of blindness [27], and currently a cure for this disease is not available. The development of therapies to enhance cell viability and restore cell function in these types of retinopathies could be used to treat these blinding conditions.
Dental Stem Cells for Retinal Cell Regeneration
Multiple groups have shown that dental pulp stem cells have an affinity to differentiate into mature neuronal cells both in vitro and in vivo [28–30]. This characteristic is attributed to the neural crest origins of these cells, since the neural crest gives rise to the nerves of the peripheral nervous system [28]. Under appropriate in vitro conditions, DPSC develop the characteristic stellate neuronal morphology, express neuronal markers at both the gene and protein levels, and produce voltage-dependent current similar to mature neurons [28, 30]. Furthermore, in vivo, neuronally- differentiated DPSC maintained their neuronal phenotype and integrate into brain tissue after transplantation [28, 29]. Because of these capabilities, researchers are investigating the capacity of DPSC to differentiate into retinal neurons.
A recent study has shown that human DPSC cultured with conditioned media from rat retinal explants differentiated into retinal neurons [31]. Conditioned medium was collected from cultures of normal rat retinal explants or explants treated with N-methyl-N-nitrosourea (MNU), which chemically damages the retina by inducing apoptosis of the photoreceptor cells. Researchers found that DPSC adopt a neuronal cell morphology when cultured with either normal or damaged retinal explant conditioned medium [31]. However, DPSC only expressed rhodopsin after being cultured with conditioned medium from damaged retinal explants [31]. This shows promising data that DPSC have the capacity to differentiate into retinal neuron-like cells, and damaged retinal cells secrete signals to induce this differentiation. Elucidating the specific signals necessary to induce retinal cell differentiation from DPSCs would be additionally powerful and allow for the development of an efficient mechanism to develop a cellular therapy to treat retinal disorders.
Similar to DPSC, periodontal ligament stem cells (PDLSC) are able to differentiate down neuronal lineages [32], and this also is attributed to the neural crest origins of this tissue. Research is now also emerging on the use of PDLSC for retinal cell differentiation [33]. To induce differentiation, PDLSCs were first cultured as neuorospheres and then plated on Matrigel-coated dishes in differentiation medium containing Noggin and Dkk-1, inhibitors of the bone morphogenic protein and Wnt/β-catenin pathways, respectively [33]. Antagonizing these pathways mimicked developmental processes necessary for anterior neural plate formation and retinogenesis. Differentiated PDLSCs expressed genes and proteins characteristic of retinal fate induction such as Pax6 and Rx, and markers of photoreceptors such as rhodopsin and Nrl [33]. This study shows promising data on the potential use of PDLSCs for retinal cell regeneration. Additional animal studies showing the in vivo behavior of these differentiated PDLSCs such as cell integration into retinal structures and cell functionality would provide further support on the potential use of PDLSCs for regeneration therapies to treat retinopathies.
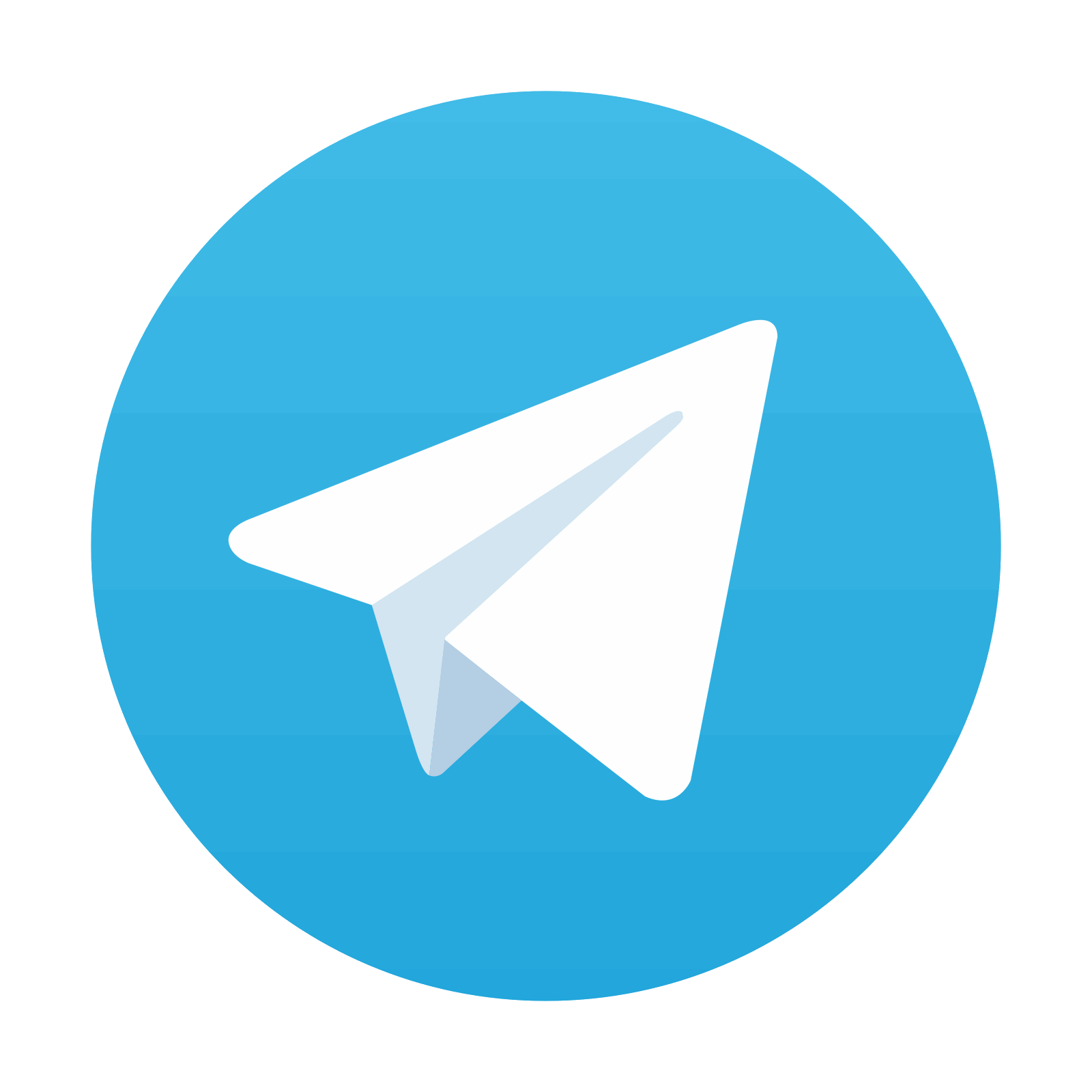
Stay updated, free dental videos. Join our Telegram channel

VIDEdental - Online dental courses
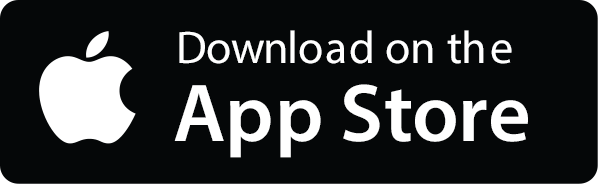
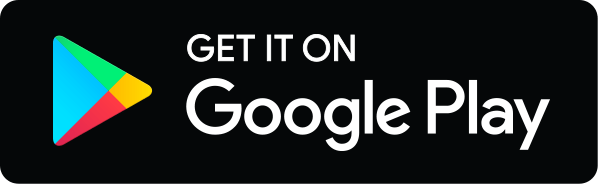