3
Embryology, Physiology, and Biochemistry of the Salivary Glands
Embryology
Salivary glands are present in amphibians upward. Parotid glands are first present in mammals. The major salivary glands start to develop as outpouchings of oral ectoderm into the surrounding mesoderm. The connective tissue in the glands is derived from neural crest cells. The salivary glands originate from solid epithelial buds.
The primordial parotid gland starts to develop in the seventh embryonic week at the site of the eventual duct orifice near the angle of the stomodeum. Three stages are described in the development of the salivary glands.1 The first stage includes the anlage and the development of branched duct buds that are dichotomous. Two cell types are present: ciliary epithelial cells that line the lumen, and myoepithelial cells covering the external surface. The early presence of myoepithelial cells is evidence that these cells are of ectodermal origin. Branching morphogenesis of the developing salivary glands is initiated by the formation of shallow clefts in a globular bud that deepen to generate new buds. Similar to lung and kidney, salivary gland branching results in repetitive epithelial cleft and bud formation, leading to complex three-dimensional branching structures. Epithelial basement membrane components such as laminin and proteoglycans are required for salivary branching and epithelial functions in general.2,3 Fibronectin, an extracellular matrix protein, is essential for cleft formation during the initiation of epithelial branching.4 A second stage includes early formation of lobules and canalization of the ducts. Myoepithelial cells are found around primitive acini and the distal segment of the duct. These functional units, which include endoplasmic reticulum and supranuclear Golgi zones, are completed at the seventh embryonic month. A dysfunction during this second stage may lead to congenital polycystic parotid. The third stage leads to further differentiation of the acinar cells and intercalated ducts with a reduction of the interstitial connective tissue. A study of 22 normal human fetus salivary glands of varying maturities removed at autopsy revealed that the functional maturity of the fetal gland occurs at 21 weeks into gestational maturity. The acini and ducts undergo distinct alterations in antigen expression with growth and maturation until 33 to 40 weeks of gestation, when they resemble adult salivary gland.5
Whereas the parotid anlage grows posteriorly, forming tubuloacinar systems, the facial nerve grows anteriorly. The gland eventually envelops the proximal extratemporal facial nerve and its major branches. An examination of human embryos and fetuses revealed no evidence of the parotid gland becoming a bilobate structure as a result of the course of the facial nerve.6 The interstitium that the parotid moves into is rich in lymphocytes. Mesenchymal tissue surrounds and penetrates the gland, entrapping lymph nodes in the process. A true capsule is not formed around the parotid gland. The parotid gland reaches its final position at the end of the third embryonal month, being the salivary gland farthest removed from its place of origin in the oral cavity. During embryonic maturation, the number of myoepithelial cells decreases, but subsequently increases after birth. The striated ducts are not recognized until birth. The first salivary secretion does not occur until after birth on the stimulation of feeding.
The medial paralingual groove constitutes the anlage of the submandibular glands that appears in the sixth embryonal week. It develops from endodermal buds in the floor of the stomodeum and grows posteriorly and lateral to the developing tongue. During the early stages of development, ducts are surrounded by a one-or two-layer thickness of cells. Acini form at 12 weeks. Myoepithelial cells lie on the external surface of the ducts and acini. Electron microscopy demonstrates desmosomes with microvilli, glycogen granules in the cytoplasm, and secretory granules in the primitive acini.1 A complete capsule is formed, and its final position, like all salivary glands, is achieved in the third embryonic month. Intraglandular lymph nodes are a rare finding.
The sublingual gland anlage forms at the ninth gestational month. It develops from multiple endodermal epithelial buds in the paralingual sulcus. The glands are penetrated by sublingual connective tissue, and a capsule is therefore absent. Intraglandular lymph nodes are not generally observed. The sublingual glands do not form major ducts. They are aggregations of minor salivary glands, which empty either directly into the oral cavity individually or through openings into the Wharton’s duct.
Minor salivary glands form directly as individual units from upper respiratory ectoderm as simple tubuloacinar systems.7,8 They appear at about the 12th week of intrauterine life. Staining for mucin suggests secretion begins in intrauterine life.9
Physiology and Biochemistry
Autonomic Nervous System
The flow of saliva is regulated predominantly by the autonomic nervous system. Control of most other gastrointestinal secretions is hormonal. There is a minimal impact from endogenous factors such as bradykinin, aldosterone, estrogen Y1, and antidiuretic hormone on saliva. The salivary glands appear to have roughly equal innervations by sympathetic and parasympathetic fibers. Although both sympathetic and parasympathetic stimulation produces saliva, the parasympathetic system is dominant.
Parasympathetic denervation leads to atrophy of the glandular tissue and decreased production of saliva, whereas sympathetic denervation yields no significantly observable effect on salivary flow.10,11
Autonomic innervation extends to vascular, acinar, and myoepithelial cells, as well as intercalated ducts. Neurotransmission by acetylcholine and vasoactive intestinal polypeptide (parasympathetic) and norepinephrine (sympathetic) is nonsynaptic. The receptors reside directly on the cell membranes, and the postganglionic axons from both types of fibers may terminate on or near the same cells. These axons can be either hypolemmal (penetrate the basement membrane) or epilemmal (do not penetrate the basement membrane). The majority of hypolemmal axons are cholinergic, although adrenergic ones also exist.11,12 Because there is no synaptic transmission, there can be a spillover effect of neurotransmitter from one cell to another.
Although parasympathetic stimulation is dominant and longer lasting, normal salivary physiology depends on the interplay between both sides of the autonomic13,14 nervous system. This autonomic duality is a common theme in animal neurophysiology; however, in the case of the salivary complex, the effects of the two sides of the autonomic nervous system are more complementary than antagonistic. The parasympathetic system is the primary stimulus for fluid formation, stimulation of glandular metabolism and growth, transport activities of acinar and ductal epithelium, and vasodilation.14 Parasympathetic stimulation has a limited role in regard to protein secretion and exocytosis and produces a high volume of saliva with low protein content. The sympathetic system is the primary stimulation of exocytosis, modulating protein composition in saliva and constriction of blood vessels, resulting in a decreased salivary gland blood flow. It has a small role in fluid secretion, possibly additive to the parasympathetic system. Both systems induce contraction of myoepithelial cells and stimulate salivary flow (Table 3-1). The sympathetic system is synergistic to the dominant parasympathetic system.
Receptors for neurotransmitters for exocrine glands are on the basolateral membranes (cells distal from the lumen).15 After the interaction of the neurotransmitter with the receptor, activation of an effector process to create a cellular response either occurs directly or via a second messenger. Predominant cholinergic, but also α1-adrenergic, stimulations are responsible for fluid and electrolyte secretion and, to a lesser extent, protein secretion. The cholinergic stimulation is mediated through the neurotransmitter, acetylcholine, binding to M3 muscarinic cholinergic receptors. Heterotrimeric guanine nucleotide–binding proteins (G proteins) are subsequently activated in the acinar cell and bind to the receptor. G proteins have α, β, and γ components (heterotrimeric). G protein binding to the receptor leads to a dissociation of the αcomponent of the G protein, leading in turn to activation of phospholipase C. This results in the formation of inositol triphosphate and the subsequent release of Ca2+ as a second messenger (Fig. 3–1). The second messenger Ca2+ is instrumental in fluid and ion transport across the apical membrane and into the ductal lumen. The neurotransmitter, norepinephine, acting on αreceptors, is an additional mediator that releases Ca2+. Atropine binds to this same M3 muscarinic receptor and can block acetylcholine. Substance P, vasoactive intestinal peptide, neuropeptide Y, and galanin are additional mediators of the parasympathetic system that are atropine resistant. When G proteins activate phospholipase C, they also form diacylglycerol that activates protein kinase C, resulting in protein exocytosis, a minor pathway for protein secretion.
Table 3-1 Functions of Autonomic Nervous System
Parasympathetic Fluid formation Glandular metabolism Glandular growth Transport activity in acinar and ductal cells Vasodilation |
Sympathetic Exocytosis Protein metabolism modulation Constriction of blood vessels |
Parasympathetic and sympathetic Stimulation of salivary flow Constriction of myoepithelial cells |
FIGURE 3-1 Autonomic nervous system.
The sympathetic stimulation produces saliva of low volume and is the major modulator of salivary protein composition and exocytosis.14 Beta-adrenergic stimulation via the sympathetic system is mediated through the binding of norepinephrine to β-adrenergic receptors (αand βreceptor categories are further subdivided into α1 and α2 and β1 and β2) and the subsequent activation of another G protein. This G protein activates adenylate cyclase, converting adenosine triphosphate (ATP) to cyclic adenosine monophosphate (cAMP). The second messenger, cAMP, activates cAMP-dependent protein kinase A, leading to protein exocytosis. Vasoactive intestinal polypeptide also serves to increase the cAMP in acinar cells.16 Alpha-2, β1, and β2 receptors affect cAMP either by stimulation (β1 and β2) or inhibition (α2). In summary, calcium is the predominant second messenger for voluminous fluid secretion and cAMP the predominant second messenger for a secretion rich in amylase, mucin, and other proteins. The opposite is true for the pancreas.
The activation of the effector process can be a direct effect of the neurotransmitter/receptor binding or via second messengers, including the release of intracellular calcium and/or activation of adenylate cyclase, leading to a buildup of cAMP. Both of these second messengers in turn activate kinases that phosphorylate a multiplicity of effector process proteins, such as myosin, plasma membrane ion pump proteins, and granule membrane associated proteins. In the case of excretory glands such as the salivary apparatus, the cellular response of this complicated process is exostosis of zymogen granules (resulting in the release of amylase and other salivary proteins), ion and fluid transport across the acinar cells and ductal cells, absorption of sodium chloride by the ductal epithelium, vasodilatation and myoepithelial contraction, as well as resetting of the surface and transmembrane potentials (Table 3-2). Other substances such as muscarinic agonist medications, hormones, and kinins can also activate these11,17–19
Acinar and Ductal Protein Secretion
Most protein secretion occurs in the acinar cells. Amino acids cross the basolateral membrane via an active transport mechanism and enter acinar cells from the interstitium. Proteins and other macromolecules (principally amylase and glycoproteins) found in saliva are produced intracellularly in the rough endoplasmic reticulum (RER) (see Chapter 4, Fig. 4–1). They are segregated in the cisternal space of the RER. Intracellular transport via an ATP mechanism carries secretory products to the Golgi complex in condensing vacuoles. Terminal glycosylation occurs in the Golgi complex. The Golgi complex has a high-permeable membrane. Concentration to secretory (zymogen) granules (a low-permeable membrane) is followed by temporary storage and subsequent release of their contents by fusion of secretory granules with the plasma membrane (exocytosis) after activation of granule membrane proteins by cAMP and calcium activated kinases.20 The principal from the pathway, responsible for the secretion of newly synthesized parotid proteins, is through storage of newly synthesized secretory proteins in the secretory (zymogen) granules, followed by regulated extracellular release in response to β-adrenergic stimulation. Ductal cells secrete a small percentage of salivary protein in the ductal lumen.
Table 3-2 Structures of Autonomic Nervous System
Parasympathetic | Sympathetic |
Cholinergic stimulation | β-adrenergic stimulation |
Acetocholine neurotransmitter | Norepinephrine neurotransmitter |
M3 muscarinic receptor | β–adrenergic receptor |
Ca2+ (2nd messenger) | cAMP (2nd messenger) |
↘ | ↙ |
Kinase phosphorylation of effector process proteins and cellular response |
cAMP, cyclic adenosine monophosphate
Acinar Electrolyte Secretion
Most of what is understood regarding salivary gland electrolyte secretion is derived from rat and rabbit animal models. The acinar cells are the site of all fluid generation. The liquid component of saliva is derived from the surrounding vasculature. Associated capillaries are extremely permeable, allowing rapid transit of water across the basement membrane. Recently discovered water-specific channels, or aquaporins (aquaporin-5 in salivary glands), may play an important role in the molecular basis of water movement across membranes (transcellular water transport pathway).21
Na+K+ adenosinetriphosphatase (ATPase) and Na+K+2Cl− are postulated pump and cotransporter, respectively, located in the basolateral membrane (cells distal from the acinar lumen) of the acinar cell that power intracellular Na+ down its electrochemical gradient, while K+ (via the Na+K+ ATPase pump) and Cl− (via the Na+K+2Cl− cotransporter) accumulate against their gradient22 (Fig. 3–2).
Therefore, in the resting unstimulated state, K+ and Cl− are concentrated in the acinar cell above the electrochemical equilibrium. With autonomic stimulation, Ca2+ (the second messenger) sensitive ion channels permeable to K+ (at the basolateral membrane) and Cl− (at the apical membrane) open.17,18,23 Cl− leaves the acinar cell into the lumen via the apical membrane. This negative charge in the acinar lumen draws Na+ from the interstitium to pass through tight junctions between the acinar cells into the lumen. Water is carried from the interstitium to the acinar lumen via an osmotic gradient produced by the NaCl in the lumen.
In the preceding model, mass and charge balance in ion transfer must be in a steady state in the acinar cell (Fig. 3–2). At the basolateral membrane, the Na+K−2Cl− (via cotransporter) goes through three cycles of entry into the acinar cell from the interstitium. This allows 3Na+3K+ and 6Cl+ to enter the acinar cell. The Na+K+ ATPase pump at the basolateral membrane results in the extrusion of 3Na+ from the acinar cell to the interstitium and the entry of 2K+ into the acinar cell from the interstitium at the energy cost of 1 ATP. The 3Na+ extruded from the acinar cell by the Na+K+ ATPase pump is balanced with the entry of 3Na+ into the acinar cell from the Na+K+2Cl− cotransporter. Na+ remains in charge and mass balance. In the resting state, there is 5K+ in the acinar cell (3K+ from the cotransporter and 2K+ from the ATPase pump). With autonomic stimulation, Ca2+ (the second messenger) sensitive ion channels permeable to K+ at the basolateral membrane allow the release of 5K+ from the acinar cell to the interstitium, maintaining a neutral charge balance of K+. When the autonomic stimulation is complete, the resting state in the acinar cell returns to 5K. Meanwhile, in the resting state, there is 6Cl− in the acinar cell (from three cycles of the Na+K+2Cl− cotransporter). With autonomic stimulation a Cl− channel opens at the apical membrane, resulting in the release of 6Cl− into the acinar lumen from the acinar cell, maintaining mass and charge balance for Cl. The 6Cl− in the acinar lumen draws 6Na+ from the interstitium to the lumen via tight junctions between the acinar cells. The 6NaCl draws water via transepithelial movement from the interstitium into the lumen from the osmotic gradient. With cessation of autonomic stimulation (Ca2+ mediated), the Cl− channel at the apical membrane and the K+ channel at the basolateral membrane close, and 6Cl− and 5K+ are reaccumulated in the acinar cell.
FIGURE 3-2 Acinar cell electrolyte secretion.
A second proposed mechanism of Cl− secretion (not diagrammed in Fig. 3–2) is an acinar cell Cl HCO3 exchanger in parallel with the Na+H+ exchanger, both at the basolateral membrane, resulting in the secretion of 3Cl− across the apical membrane into the acinar lumen. Another Cl− channel (not diagrammed in Fig. 3–2) is a volume-sensitive Cl− channel activated by cell swelling and inhibited by cell shrinkage. Lastly, in the resting state, voltage-activated Cl− channels in the apical membrane may also exist.
Acinar bicarbonate secretion (HCO3−) into the acinar lumen results from the following postulated mechanism (Fig. 3–2). CO2 diffuses across the basolateral membrane. CO2 and H2O are converted to HCO3− and H+ by carbonic anhydrase. The HCO3− crosses the apical membrane via an anion channel (possibly the same anion channel used by Cl), while H+ is extruded across the basolateral membrane via the Na+/H+ exchanger.
Mass and charge balance in ion transfer must again be in a steady state in the acinar cell (Fig. 3–2). The Na+K+ ATPase pump drives the extrusion of 3Na+ from the acinar cell to the interstitium in exchange for 2K+ entering the acinar cell from the interstitium at a cost of 1 ATP. The parasympathetic-stimulated Ca2+ mediated channel allows the exit of 2K+ from the acinar cell back out to the interstitium for K+ balance. The Na+H+ exchanger brings the 3Na+ back into the acinar cell from the interstitium for Na+ balance. Three cycles of CO2 and H2O are converted to HCO3− and H+ by carbonic anhydrase. The 3H+ produced in the acinar cell is balanced by a 3H+ extrusion from the acinar cell into the interstitium via the Na+H+ exchanger. The ultimate result is the release of 3HCO3− from the acinar cell to the lumen (at an energy cost of 1 ATP).
Ductal Electrolyte Secretion
At the time that saliva is secreted by the acini, it is just slightly less than isotonic with plasma, but this is actively modified as it moves through the intercalated and probably the striated ducts (composition of the primary secretion is altered, but not the volume). The Na+K+ ATPase located in the basolateral membrane of the ductal epithelial cell maintains the electrochemical potential gradient of Na+ and K+ that powers most of the other ionic transfer processes in the cell (Fig. 3–3). Ductal cells do not express detectable levels of Na+K+2Cl− cotransporter activity. Therefore, low concentrations of Cl− exist in the ductal cell, providing a more favorable condition for Cl− entry into the ductal cell when the Cl− channel opens.
As the primary secretion moves through these ducts, it becomes higher in potassium and bicarbonate concentration (potassium and bicarbonate are secreted) and lower in sodium and chloride concentration (sodium and chloride are reabsorbed), but always hypotonic to plasma. Na+ and Cl− in saliva are less than measured in serum, but K+ is always much greater in saliva compared with serum. When salivary flow rates are very low, the secreted salivary K+ levels are high. The more slowly flowing basal secretions are considerably hypotonic to plasma, but stimulated salivary secretions flow rapidly and are thus less modified by the ductal system and only slightly hypotonic. At maximal flow rates the tonicity of saliva is 70% of plasma.
FIGURE 3-3 Ductal cell electrolyte secretion.
Mass and charge balance in ion transfer must be in a steady state in the ductal cell as well. The Na+K+ ATPase pump again at the basolateral membrane draws 3Na+ out of the ductal cell into the interstitium in exchange for 2K+ to enter the ductal cell at an energy cost of 1 ATP. Some K+ leaves the ductal cell back to the interstitium at the basolateral membrane via a K+ channel. K+ that enters the ductal cell from the interstitium via the Na+K+ pump can exit the ductal cell by the above-mentioned K+ channel at the basolateral membrane or an apical K+H+ exchanger. When K+ is secreted from the ductal cell into the ductal lumen by the apical K+H+ exchanger, H+ enters the ductal cell. This H+ can leave the ductal cell into the interstitium via a basolateral Na+H+ exchanger. The overall result is a low intracellular concentration of Na. Na+ can now be absorbed into the ductal cell from the ductal lumen from either the Na+ channel (primary mechanism of uptake) or an apical membrane Na+H+ exchanger. Cl− to maintain charge balance in the ductal cell is absorbed into the ductal cell from the ductal lumen from a Cl− channel (primary mechanism of uptake) or the Cl−HCO3− exchanger. When Cl− is absorbed from the ductal lumen to the ductal cell via a Cl−HCO3− exchanger, HCO3− is secreted into the ductal lumen. Cl− subsequently leaves the ductal cell at the basolateral membrane via a Cl− channel drawn out by K+ through the K+ channel mentioned above. The end result is that NaCl is absorbed into the ductal cell from the ductal lumen, and KHCO3 is secreted from the ductal cell into the ductal lumen.
In addition to parasympathetic cholinergic (second messenger Ca2+) activated Cl− channels in ductal (and acinar) cells, there is a sympathetic β-adrenergic stimulation activated second messenger, cAMP, that activates a duct Cl− channel. cAMP may be required for efficent NaCl absorption in many ductal cells. cAMP activates the cystic fibrosis transmembrane conductance regulator located in the apical membrane, resulting in the opening of a Cl− channel and absorption of Cl− into the ductal cell. Mutations of this gene result in cystic fibrosis, characterized by reduced flow rate and elevated Na+ and Cl− and subsequent mucous plugs.
Sialochemistry
Proteins
Whole saliva is composed of ~99.5% water and therefore has a much lower protein concentration than plasma. The remaining 0.5% is composed of large organic molecules, including proteins, glycoproteins, and lipids; small organic molecules, including glucose and urea; and the electrolytes, chiefly potassium, bicarbonate, sodium, chloride, calcium, and phosphates. Saliva has a specific gravity, depending on flow rate, of 1.002 to 1.012.
Humans secrete between 0.5 and 1.5 L of saliva daily. The parotid and submandibular glands together produce ~90 to 95% of the basal (unstimulated) total salivary flow (Table 3-3). The submandibular gland saliva is more viscous due to its higher mucin content. It has a higher basal flow rate than the parotid. However, this relationship is reversed upon stimulation. During eating, the parotid gland produces about two thirds of the salivary flow, a proteinaceous, watery secretion.24,25 Sublingual gland isolates are more viscous than submandibular gland secretions, with a higher mucous content. The minor salivary glands are purely mucous glands and produce ~5% of the total saliva.
Proteins, which are actively transported, make up the vast majority of the organic components of saliva. Most salivary organic molecules are produced in the acinar cells, with a smaller quantity produced in the ducts or transported into the saliva from blood. The protein content of the parotid gland saliva is higher than that of the submandibular gland even though the viscosity of saliva from the latter is greater.10,25
Alpha-amylase is the most important and abundant protein found in saliva, comprising, 10% of salivary protein. Electrophoresis demonstrates that α-amylase is composed of several isoenzymes. Total amylase production for humans is estimated to be 1.5 g a day, with the pancreas secreting 60% and the salivary glands 40%.10 The parotid glands secrete 70% of the salivary amylase.
There are many salivary glycoproteins produced by the acinar cells. Glycoproteins are involved in tissue coating, lubrication, buffering, digestion, and microbial clearance.26 Salivary glycoproteins have a polypeptide core and oligosaccharide side chains. These vary between the serous parotid (N-linked oligosaccharides such as α-amylase, salivary peroxidase, and proline-rich glycoproteins) and mucous submandibular, sublingual, and minor salivary glands (O-linked oligosaccharides), producing cells that are the main determinant of viscosity of saliva. The mucins (mucous glycoproteins) are subdivided into mucin 1 (gene designation MUC5B) and mucin 2 (gene designation MUC7). Mucin 1 is a high molecular weight multisubunit molecule that is highly glycosylated (a larger carbohydrate component). Mucin 2 is a low molecular weight single-polypeptide molecule that is glycosylated.26
Table 3-3 Salivary Gland Secretion
Parotid gland secretion Proteinaceous, watery, serous secretion Two thirds of salivary flow during gustatory and olfactory stimulation Organic (proteins including enzymes) and inorganic materials are higher |
Submandibular gland secretion High mucin content, viscous/serous secretion Higher basal flow rate Calcium is higher |
Sublingual gland secretion Higher mucin content than submandibular gland 5% of salivary flow |
Minor salivary gland secretion Purely mucous glands 5% of salivary flow |
Blood group reactive substances are glycoproteins found on the cell membranes and in low concentrations in saliva, predominantly in labial minor salivary glands.10 The ability to secrete blood group reactive substances (A, B, AB, and O) is inherited as a dominant factor independent of the ABO blood classification system and is absent in recessive homozygotes, information important in the forensic sciences.
Other enzymes (proteins) secreted in saliva include muramidase, phosphatases, hydrolases, dehydrogenases, arginase, and esterases.27 Many other proteins have been isolated from saliva,28 including albumin, amino acids, polypeptides and proline-rich, histatin-rich, and tyrosine-rich proteins. The histatin-rich proteins have antifungal properties, whereas another salivary protein, secretory leukocyte protease inhibitor (SLPI), has antiviral capabilities. Albumin passively diffuses into the saliva from plasma and functions as a carrier protein. The concentration of organic (and inorganic) substances is highly variable and depends on which gland is being measured and the flow rate. Smaller organic elements such as urea, glucose, amino acids, and lipids are passively diffused from the plasma and are therefore directly correlated to serum concentrations.
Several polypeptides have been isolated from mammalian submandibular glands. These include nerve growth factor, epidermal growth factor, renin, kallikrein, and peptidases.27,29 Most of these are androgen dependent, and their secretion is mediated by α-adrenergic control.27 They are found in or considered to be secreted by the striated ductal cells. These salivary polypeptides have also been found in blood. This raises the issue of a possible endocrine or paracrine function of the salivary glands.29 The functions of salivary kallekrein, nerve growth factor, and renin are unknown. Salivary epidermal growth factor may have a role in oral wound healing and head and neck cancer.
Electrolytes
The sodium, chloride, and calcium of the salivary secretion rise during stimulation (they are not reabsorbed by the ductal system), whereas phosphate, urea, ammonia, uric acid, and magnesium fall.25,30 Increased salivary flow correlates with an increase in osmolality.19,31 In the unstimulated parotid gland, the secretion of potassium ion concentration is higher than the sodium ion concentration; with stimulation, the ratio is reversed because of decreased Na+ reabsorption in the salivary ducts at higher rates.31 Salivary electrolyte concentrations are different than those found in serum because of the active transport of ions across the acinar and ductal cells. The large number of variables that influence electrolyte concentration reduces the accuracy of reliable laboratory ranges compared with serum electrolyte concentrations.
In general, the concentration of both organic (proteins including enzymes) and inorganic materials is higher in the parotid than in the submandibular gland, with the exception of calcium.25 The concentration of calcium in the unstimulated submandibular gland is 2 to 8 mmol/L, compared with 0.2 to 2.5 mmol/L in the parotid gland.31 Calcium is flow dependent only at very high rates.22 Calcium in the parotid gland exists in an ionized and protein-bound form in a 1:1 ratio. Calculi (also referred to as sialoliths) are more common in the submandibular gland than in the parotid gland. Magnesium concentrations are equivalent in all salivary glands and are two thirds of the plasma concentration.26
The concentration of bicarbonate (HCO3−) in saliva is greater than plasma concentration except at the lowest flow rates. The hydrogen ion concentration (pH) hovers around neutral, with the unstimulated parotid and submandibular glands having slightly acidic values.31 With increasing flow rate, HCO3− increases, dihydrogen phosphate (H2PO4−) decreases, and the pH increases to an alkaline state.31 The pH rises from a low of 5.75 (unstimulated, slow flow) to nearly 8.0 (stimulated, rapid flow).10 Bicarbonate (H2CO3/HCO3−) and, to a lesser extent, phosphate (H2PO4/HPO4−) are two buffer systems that, coupled with proteins, provide saliva with a buffer capacity.
Salivary fluoride is similar to plasma but is increased in individuals who drink fluorinated water or use fluorinated toothpaste. There is a slow, prolonged release of fluoride into the oral cavity from soluble deposits on the teeth that prevent dental caries.26
Sialometry
Sialometry measures the flow rate of saliva. Unstimulated saliva should be collected 2 hours after eating or after an overnight fast. Whole saliva (a total product of all salivary glands) can be measured by a variety of volumetric and gravimetric techniques, including drooling, spitting, suction, and swab. Isolated parotid secretion can be obtained by placement of a suction cup over the Stensen’s duct, usually referred to as a Carlson-Crittenden cup or Lashley cup. Collection of unstimulated saliva requires a person to drool quietly into a preweighed collection vial, expectorating each minute, for up to a total of 5 minutes.32 Stimulated saliva can use a masticatory stimulus (flavorless gum base or paraffin wax chewed 60 times/min) or a gustatory stimulus (citric acid) with a similar collection period.
Dynamic radionuclide scintigraphy measures uptake, concentration, and secretion of salivary glands using intravenously injected technetium 99m pertechnetate. It provides an assessment of the functional capacity of the major salivary glands; sequential scanning demonstrates technetium 99m pertechnetate uptake from the vascular system and secretion in saliva. In diseased states there is decreased and delayed uptake, and in the severely atrophic gland there is diminished output from the glands into the mouth. Sialography, computed tomography, and magnetic resonance imaging (MRI) have largely replaced scintigraphy. In vivo nuclear MRI has been used to analyze salivary production in the unstimulated and stimulated parotid gland.33
Normal values for salivary flow rates are difficult to establish because of circadian rhythms and other factors that vary the flow rate. There is a nearly 50% normal variability in salivary flow rates in healthy individuals over time.34 Salivary production rates drop to their lowest levels during sleep. Emotional states are asso ciated with expected higher flow rates35,36. Climate, light, age, gender, physical activity, hydration, and personality potentially impact salivary flow. Empty mouth clenching does not appear to be a stimulus of salivary flow.35 There are also enormous variations of flow rates, both stimulated and unstimulated, between study subjects selected free of subjective complaints or signs of salivary gland dysfunction.37
The average daily saliva secretion as stated above is 0.5 to 1.5 L daily. Averages for resting flow rates for whole saliva are 0.3 to 0.4 mL/min, rising to 1 to 2 mL/min in paraffin-stimulated saliva.38 Unstimulated whole salivary flow rates of less than 0.1 mL/min and stimulated whole flow rates less than 0.4 mL/min are considered to be one criterion for severe salivary hypofunction.39 Oral dryness is typically noticed when the stimulated rate drops below 0.2 mL/min/gland.25 Change in an individual’s salivary flow rate is a more reliable sign of dysfunction than comparison to normative data, and it has been suggested that individual decrements of more than 45% of normal values are indicative of salivary hypofunction.34 In experiments designed to reduce salivary production with anticholinergic drugs, dryness was noted when the flow rates were reduced by 50% of the individual’s pretreatment flow rate.40
The metabolic rate of salivary tissue, as in other secretory organs, is quite high. Spontaneous activity from the salivatory nuclei in the brainstem results in continuous secretion of saliva (unstimulated) without gustatory and masticatory stimuli and serves as a protective secretion. Saliva can increase to a maximum of ~1 mL/min/g of tissue in response to the marked release of neurotransmitters. At this rate the glands are producing their own weight in saliva each minute. This assists in food bolus formation and swallowing. The increase in salivary production is paralleled by an increase in blood flow, which can approach 10 times the rate of blood flow in actively contracting skeletal muscle.27 Maximal β-adrenergic stimulation of the parotid gland can result in the discharge of 70 to 80% of total stored secretory proteins within 1 hour. This process involves rapid fusion of granule membranes with the apical membrane (exostosis) and with membranes of other organelles.
Eighty to 90% of salivary production occurs with stimulation. The highest flow rates are achieved with stimulation during eating. Gustatory receptors in the oral cavity will stimulate the salivary nucleus in the medulla, resulting in autonomic stimulation of the salivary glands. Acid tastes create the highest salivary flow, and sweet tastes the least. The mechanical act of chewing stimulates saliva production. Olfaction will also increase the flow rate, but to a lesser degree.
Partial surgical removal of one parotid gland does decrease flow in that gland but is not associated with complaints of xerostomia and may even cause compensatory increase in secretion from other glands.41 Cunning et al24 reported a decrease in unstimulated salivary flow in patients undergoing unilateral submandibular gland excision with increased subjective complaints of xerostomia. Stimulated flow was not significantly different. In general, subjective postoperative complaints of dryness after unilateral submandibular gland resection are not common.
Salivary Gland Function
There are three major functions of the salivary glands: nutritional intake, communication, and host protection (Table 3-4). Saliva production is critical for efficient mastication and deglutition and thus is a significant component of the digestive process and overall nutrition. Alpha-amylase, secreted in high quantities by the human salivary glands, begins the enzymatic process of digestion of starch while the food is still in the oral cavity. Amylase reduces starch to oligosaccharide molecules by cleaving α-1, 4-glycosidic bonds. More then half of the starch may be reduced to oligosaccharides by the action of salivary amylase in a well-chewed meal. In the absence of salivary amylase, digestion of starch is still possible because of pancreatic amylase. The optimal pH for amylase is 7, but starch digestion can occur between a pH of 4 and 11. Its activity is terminated below a pH of 4 in the stomach antrum. Alpha-amylase initially continues to digest starch in the gastrointestinal tract because the food bolus protects it from gastric acid. The main end products of amylase digestion are maltose, oligosaccharides, and free glucose.
Table 3-4 Function of Saliva
Digestion |
Lubrication |
Buffer |
Transport to taste receptors |
Antimicrobial |
Dental protective |
Excretory |
Lingual lipase produced by the minor salivary glands of the tongue initiates the digestion of triglycerides. It stays active in the stomach because of its acid pH. Proteolytic function is not demonstrated to a significant degree in saliva in contrast to pancreatic secretions.
Saliva lubricates the crushed food, allowing for bolus formation, enzymatic digestion, and swallowing. The lubrication function of saliva is dependent primarily on mucous glycoproteins (and to a lesser extent on proline-rich polypeptides) that bind to water and keep the oral cavity hydrated. Mucins are present in higher concentration in the submandibular, sublingual, and minor salivary glands. Mucins form long end-to-end oligomers to manifest their lubricating properties.42 Salivary mucins coat the oral mucosa, preventing desiccation. Dry mouth from diminished saliva production is a common complaint. Desiccated mucosa is uncomfortable and more prone to trauma and infection. Some patients with stomatodynia (burning mouth) complain of xerostomia,43–45 which could be due to changes in salivary composition and flow,46 especially in the minor salivary glands.44 The lubrication process is a prerequisite for speech, mastication, and swallowing.
The lubrication function of saliva is also essential to the sense of taste. Saliva transports the bolus to the taste receptor and protects the receptor. Saliva helps dissolve and carry tastants to the taste buds, and is therefore a critical component of the gustatory system.47 Subjective taste disturbances and oral pain from dry mouth lead to decreased appetite and therefore potentially to malnutrition.
Saliva buffers acids (saliva has a slightly alkaline pH) and dissipates heat. Saliva buffers and dilutes noxious substances that can be expectorated by copious salivation. The chief buffer is bicarbonate (HCO3−) and, to a lesser extent, urea, phosphate, and histidine-rich proteins. The bicarbonate concentration in saliva is higher at stimulated (higher) flow rates. Hot solutions are diluted and their temperature lowered. During emesis saliva buffers and dilutes gastric acids.
Table 3-5 The Role of Salivary Proteins in Antimicrobial Clearance
Secretory immunoglobulin A (sIgA), IgG, IgMdash;antibacterial and antiviral properties |
Mucin 2—prevents the adhesion and promotes clearance of microorganisms to the oral cavity |
Amylase when coupled with mucin 1 clears bacteria from saliva |
Peroxidase—antimicrobial function |
Lysozyme—hydrolyzes a major component of bacterial outer membranes and activates autolysins |
Histatine-rich proteins—antifungal properties |
Lactoferrin—chelates iron, reducing the ability of microorganisms requiring iron |
Secretory leukocyte protease inhibitor (SLPI)—antiviral activities |
Proline-rich peptides inhibit herpes simplex virus l |
SLPI antiviral activities |
High- and low-weight glycoproteins—inhibit HIV infection |
HIV, human immunodeficiency virus
Immune-mediated antimicrobial proteins (Table 3-5) associated with the major salivary glands include secretory immunoglobulin A (sIgA) and, to a much lesser extent, immunoglobulins G and M.28 Dimeric IgA (two IgA macromolecules covalently linked by a J chain) is formed by the plasma cells in the interstitium of the salivary gland. It is transported into the acinar cell by an active transport mechanism. The “secretory component” of salivary IgA, both bound to dimers of serum type IgA and “free,” differs from serum IgA and is synthesized on the basolateral surface of acinar cells.10,28 It helps in the transport of IgA across the apical membrane of the acinar cell into the duct. sIgA is stimulated by bacteria and viruses reaching the mucosa. Mucins and enzymes are non-immunemediated agents that protect the oral cavity from microorganisms. Mucin 2 prevents the adhesion and promotes clearance of microorganisms to the oral cavity. Mucin 1 cradles other salivary molecules such as enzymes (amylase, histatins, proline-rich proteins, and statherin) and immunoglobulins and carries them to their eventual target sites. Amylase when coupled with mucin 1 helps clear bacteria from saliva when it is in solution. Amylase and salivary glycoproteins bind to a select group of Streptococci. Lysozyme hydrolyzes a major component of bacterial outer membranes and activates autolysins. Lactoferrin chelates iron, reducing the ability of microorganisms (and neoplasms) that require iron for growth. It is also bacteriocidal. Histatins exhibit antibacterial and candidal activity. Proline-rich peptides play an important role in inhibition of herpes simplex virus l,48 and SLPI purportedly has antiviral (especially human immunodeficiency virus [HIV]) activities. Peroxidase also has antimicrobial functions.49,50
The tooth surface has a protective film of mucin (produced by the submandibular gland and sublingual gland) and proline-rich glycoprotein (produced by the parotid gland) that protect against wear. Mucin 1 adsorbs to the tooth enamel, protecting the tooth from acid. Salivary mucins aggregate oral bacteria, assisting in the prevention of dental plaque formation, and thus help promote normal tooth development.51 Salivary amylase and mucin complexes can also help promote enamel breakdown, if they are absorbed to the tooth surface, by breaking down starches into simpler sugars that are used by bacteria to create enamel-destroying acids.26,27,52 Demineralization occurs when acids from attached bacteria erode through enamel. The basic pH of saliva helps prevent dental caries. The salivary bicarbonate (buffer) neutralizes acid. Demineralization is also retarded by proteins in concert with calcium and phosphate ions in saliva. Saliva has been shown to contribute to tooth enamel formation (remineralization) by supplying inorganic ions, including calcium, phosphates, fluoride, and magnesium.49 The salivary protein statherin, a small phosphoprotein rich in tyrosine and proline, functions to stabilize inorganic ions in solution, promoting remineralization. Statherin prevents precipitation of calcium phosphates from supersaturated solutions, which also plays a role in preventing salivary calculi.26 Salivary proteins (mucins, histatin, proline-rich proteins, and statherin) also bind to hydroxyapatite on the tooth surface, adding dental protection. Chewing sugar-free gum has become accepted as an adjunct of oral hygiene because of its stimulation of saliva. Decreased salivary flow rate is associated with accelerated tooth decay (dental caries), microbial infections, and subsequent loss of teeth.
Human salivary glands have an excretory function secreting exogenous elements, such as viruses (including rabies, polio, hepatitis B, and HIV), as well as antibiotics and other inorganic elements, such as mercury, lead, sulfur, and iodine. HIV may be recovered from whole saliva or individual glands. Saliva inhibits HIV infection by the action of high molecular weight components in combination with low molecular weight components from serous as well as mucous saliva affecting different stages of the infection cycle.53 The end result is the mouth is not a transmission route for HIV infection.
The preceding discussion on salivary function suggests that salivary proteins are multifunctional with overlapping roles. This redundancy allows deficiency of one protein to be compensated for by another. Salivary proteins can also cause contradictory outcomes; that is, amylase and mucin can clear bacteria from saliva when it is in solution and yet bind bacteria on tooth enamel, resulting in dental caries.
Pathophysiologic States
Systemic Diseases
Autoimmune diseases are a heterogeneous class of immunoregulatory disorders that usually affect multiple organ systems. The most common systemic disease associated with salivary disorders is Sjögren’s syndrome (SS), an autoimmune exocrinopathy that affects primarily postmenopausal females, with signs and symptoms of xerostomia and xerophthalmia (see Chapter 5).54,55 The American European Consensus Group recently revised the classification criteria for SS, which includes serological, oral, and ophthalmological evaluations and subjective complaints of dry eyes and xerostomia, and it can be used for diagnosing primary and secondary SS.56 Reported prevalence for primary SS varies from 0.05 to 4.8% of the population,57 with approximately one million people in the United States estimated to have the disease. The onset of disease is often insidious, and accordingly, diagnosis may be delayed for many years. The female to male ratio has been estimated to be 9:1, although reported ratios vary considerably. Collagen-vascular diseases associated with Sjögren’s syndrome (i. e., rheumatoid arthritis, systemic lupus erythematosus, and scleroderma) also cause decreased salivary flow, changes in salivary protein secretion, and alteration of ductal electrolyte absorption.54 A patient with a connective tissue disorder that manifests as an advanced autoimmune arthritis such as rheumatoid arthritis may not have salivary dysfunction, whereas another who has minimal joint disease may have fulminate SS.
Less commonly reported is autoimmune pancreatitis that is correlated with decreased salivary function that improves with steroid therapy.58,59 Hashimoto’s thyroiditis and hypothyroidism have decreased salivary flow.60,61 Patients with graft versus host disease from a bone marrow transplant present with sicca syndrome and lymphocytic infiltration of the salivary glands. The xerostomia resulting from an interaction between the bone marrow transplant and salivary gland tissue can be as profound as after head and neck radiation therapy. Sarcoidosis can involve the parotid gland in 10% of patients. It is characterized by bilateral glandular enlargement and xerostomia. HIV infection, which is not an autoimmune process, though it directly affects the immune system, can cause a diffuse infiltrative lymphocytosis syndrome (DILS) characterized by the persistence of CD 8 cells and lymphocytic infiltration of the salivary glands.62
Endocrine disorders, including diabetes, tend to cause protein alterations and glandular enlargement (sialosis). Individuals with diabetes have greater complaints of dry mouth,63 but not necessarily lower salivary flow rates. More likely, it is the poor glycemic control that is associated with a dry mouth.64 Hypertension leads to a decrease in salivary sodium levels but probably does not contribute to salivary hypofunction. Medication for hypertension rather than hypertension itself leads to reduced salivary flow rates.65 Chronic renal failure in patients receiving renal dialysis can lead to decreased excretory function.66 Patients with alcoholic cirrhosis have lower stimulated and unstimulated salivary flow rates and lower concentration of electrolytes and proteins.67 Protein and vitamin deficiencies can also cause salivary gland hypertrophy or swelling (sialosis).
Genetic Disorders
Ectodermal dysplasias are a heterogeneous group of conditions comprised of developmental defects of two or more ectodermal derivatives, including the hair, nails, teeth, and sweat glands. Patients with these dysplasias appear to have reduced salivary flow rates, especially of the submandibular glands.68
Cystic fibrosis (mucoviscoidosis), an autosomal recessive disorder, leads to abnormal chloride regulation in exocrine glands, including sweat glands and pulmonary and salivary mucous glands. Chloride channels can be found on apical and basolateral membranes of acinar cells and are critical for fluid secretion and NaCl resorption.69 The ultimate effect of these changes is increased protein, sodium, chloride, calcium, and urea concentrations27 in saliva, with a decrease in flow rate resulting in an inspissation of secretions and sludging. These changes can lead to stone formation, obstruction, and infection. Microscopic examination of glands shows acinar atrophy and ductal ectasia.22
Head and Neck Radiotherapy
Radiotherapy, a common treatment modality for head and neck cancers, causes severe and permanent salivary hypofunction with persistent complaints of xerostomia70 (see Chapter 13). Patients frequently experience the spectrum of oral health problems associated with salivary hypofunction, including new and recurrent dental caries, oral candidiasis, dysphagia, dysgeusia, difficulty with mastication, impaired use of removable prostheses, and an altered quality of life.71
The serous acini that produce saliva are considered to be highly radiosensitive and undergo interphase cell death by apoptosis when exposed to external beam radiotherapy. Within 1 week of the start of irradiation (after 10 Gy has been delivered), salivary output declines by 60 to 90%, with later recovery only if the total dose to salivary tissue is <25 Gy.72,73 Most patients receive therapeutic dosages that exceed 60 Gy, and their salivary glands undergo atrophy and become fibrotic.
Therapeutic doses of iodine 131 for thyroid cancer result in occasional long-term salivary damage because of the uptake of iodine 131 by the salivary glands. Fortunately, most cases are transient and can be treated with sialagogues.
Medications and Toxins
The most common cause of salivary disorders is prescription and nonprescription medications (Table 3-6). For example, 80% of the most commonly prescribed medications have been reported to cause xerostomia,74 with over 400 medications causing a side effect of salivary gland dysfunction.75 Polypharmacy, regardless of the actual medications taken, is associated with subjective complaints of xerostomia as well.76
All drugs acting on the autonomic nervous system impact the salivary glands. Although certain cholinergic agonists are known to cause increased salivation, a far greater number of pharmacologic agents cause decreased salivary output due to their anticholinergic and/or antiadrenergic effects.
The anticholinergic effects of the plant Atropa belladonna have been known throughout history. Belladonna was a poison used in classical times and has also been used medicinally for centuries. Jimson weed and stink apple are also known for their toxic effects. Both of these plants contain the alkaloid atropine. Hyoscyamus niger contains the closely related alkaloid scopolamine. These compounds cause decreased salivation as well as other anticholinergic effects.
The most common types of medications causing salivary dysfunction have anticholinergic effects, which will inhibit the movement of fluid from serum, through salivary acinar cells, into the ductal system and ultimately into the mouth. Several drugs are used specifically for their anticholinergic effects and will cause profound salivary inhibition, including atropine, scopolamine, methscopolamine, tolterodine glycopyrrolate, and ipitrobium bromide. Any drugs that inhibit neurotransmitter binding to acinar membrane receptors, or that perturb ion transport pathways, may also adversely affect the quality and quantity of salivary output. These medications include tricyclic antidepressants, sedatives and tranquilizers, antihistamines, antihypertensives (α-and β-blockers, diuretics, calcium channel blockers, angiotensin converting enzyme inhibitors), cytotoxic agents, and antiparkinsonian and antiseizure drugs.75–83 Antidepressant drugs are among the strongest inhibitors of salivation due to their anticholinergic side effects. These include phenothiazines such as chlorpromazine, tricyclic antidepressants, and newer selective serotonin reuptake inhibitor antidepressants that have reduced peripheral anticholinergic effects yet are still associated with dry mouth symptoms. Venlafaxine, another antidepressant, is both a serotonin and a norepinephrine reuptake inhibitor that causes clinical xerostomia in some patients. Bupropion, an antidepressant, whose mechanism of action is not well understood, also causes dry mouth.
Table 3-6 Medications and Xerostomia
Anticholinergic drugs: atropine, scopolamine, methscopolamine, tolterodine glycopyrrolate, and ipitrobium bromide |
Antidepressant drugs: phenothiazines, selective serotonin reuptake inhibitors |
Antihistamines (first-generation): ethanolamines, ethylenediamines, alkylamines, piperazines, and phenothiazines |
Antihypertensives: b-blockers, clonidine (inhibits central a-adrenergic receptors), guanethidine and methyldopa (inhibit the postganglionic sympathetic stimulation), diuretics (intravascular volume depletion) |
Seizure medication: carbamazepine |
ADHD: atomoxitine (norepinehrine uptake inhibitors) |
Chemotherapy: recombinant interleukin-2 (rIL-2) |
ADHD, attention deficit/hyperactivity disorder
Virtually all of the first-generation antihistamines, including the ethanolamines, ethylenediamines, alkylamines, piperazines, and phenothiazines, cause significant dry mouth due to their anticholinergic effects. The newer “second-generation” antihistamines, such as astemazole, terfenadine, fexofenadine, cetyrizine, and loratadine, have markedly less central nervous system and anticholinergic effects. A notable exception is cetyrizine, which is derived from hydroxyzine, an anxiolytic and antihistamine, and keeps some of its anticholinergic properties. Antihypertensives, especially β-blockers, are associated with xerostomia. Their use results in decreased protein secretion as well as decreased salivary flow.76 Clonidine inhibits parasympathetic innervation by stimulation of central α-adrenergic receptors. Guanethidine and methyldopa inhibit the postganglionic sympathetic stimulation. Diuretics are a common cause of xerostomia, although this effect is usually indirect through intravascular volume depletion. Pain in the parotid gland with gustatory stimulation can be caused by antihypertensive drugs.
Carbamazepine, which is used to treat seizures, commonly causes dry mouth. Amphetamines and norepinephrine uptake inhibitors, such as atomoxitine, which are commonly used to treat attention deficit/ hyperactivity disorder (ADHD), are known as a class to cause salivary dysfunction.
Chemotherapy for cancer treatment has also been associated with salivary disorders.84 Fortunately, these changes appear to occur only during and immediately after treatment, and salivary function returns to pre-chemotherapy levels in most patients after completion of therapy. Recombinant interleukin-2 (rIL-2) utilized as a chemotherapeutic agent for cancer is known to cause xerostomia. Recent studies have demonstrated that salivary flow rates are reduced during rIL-2 treatment. Salivary composition is markedly altered on measurement of basal flow, but not stimulated flow.85
There are cholinergic agonists that can increase salivary output. South American natives have long known that chewing the leaves of the Pilocarpus plants caused salivation and sweating. Pilocarpine is used to promote salivary flow and reduce symptoms of xerostomia in patients with Sjögren’s syndrome86,87 and in patients who have received head and neck radiotherapy for cancer.88 Another muscarinic agonist, cevimeline hydrochloride (an M1-and M3-specific receptor agonist), has demonstrated effectiveness for patients with Sjögren’s syndrome89,90 and is used in patients undergoing head and neck radiotherapy (see Chapter 13)
Metochlopramide and urecholine are found to promote gastrointestinal and bladder motility but are known to cause salivation as a side effect. Bethanachol and carbachol are choline esters that stimulate muscarinic receptors. Guaifenesin has been said to improve mucosal hydration through increased salivary production by an unknown mechanism, although its clinical effects appear to be limited.
Sialorrhea
Drooling can be caused by excessive salivary secretion or loss of oral competence. Medication effects and toxicity such as from household pesticides should be considered. In younger patients it can be from birth or trauma-related neurological disorders and/or dysautonomias that lead to poor oral motor competence. Treatment is often pursued because of the difficulties of maintaining proper hygiene and due to impaired social interactions (see Chapter 8). Anticholinergic medications can be tried if side effects such as hypersomnolence and gastroparesis are tolerated.
Elderly patients are also subject to this problem. Drooling can be caused by aberrant autonomic control (diabetic autonomic neuropathy, Parkinson’s disease) and/or by poor oral competence from neuromuscular diseases (cerebral palsy). Surgery for oral or oropharyngeal disorders, gastroesphogeal reflux disease, or simply loss of teeth that leads to a reduction of mandibular mass and volume are causes of sialorrhea. Antisialagogues are generally contraindicated in this group of patients, typically due to potential complications with concomitant medications and to the potential for significant side effects in the elderly. Low-dose radiation therapy to the parotid and/or submandibular glands has uncommonly been recommended in this population, although its practice in a younger population would be strictly contraindicated due to the risk of delayed neoplasms.91
Salivary Physiology with Aging
The aging salivary glands are known to undergo structural changes. The lobule structure becomes less ordered, acini vary more in size and eventually atrophy, interlobular ducts become more prominent, and the percentage of fibroadipose tissue increases.27 These changes noted in the submandibular gland and, to a lesser degree, in the parotid92 include shrinkage of cells, dilation of ducts, oncocytic transformation, increased adiposity, fibrosis, focal microcalcifications with obstruction, and chronic inflammation. Similar changes occur in the minor salivary glands with aging.
Approximately 20 to 25% of the elderly population complains of dry mouth.93 Many authors conclude the frequent complaint of dry mouth in the elderly is secondary to systemic disease and medications and not a by-product of aging itself.94–98 The lack of increased age-related variability in parotid salivary flow rates over repeated measures in one report suggests that parotid glands have a large secretory reserve.99 A recent study using isoproteronol, cAMP analogues, and carbachol to study protein fluid secretion showed a decrease in response to all of these compounds in cell lines from patients over 70 years old compared with younger patients. This suggests that there is a decrease in secretory reserve with age.100 This diminished secretory reserve has clinical sequelae: The older person is more vulnerable to the deleterious effects of medications and systemic diseases associated with salivary disorders.34
In contrast, an uncontrolled English population study showed age, female gender, and medication to be independent risk factors for xerostomia.101 Similar findings have been reported elsewhere.83,102–104 A decreased flow in the intensively stimulated submandibular gland of the aged has been reported, but this same study did not show a significant change in stimulated flow within the parotid gland.105 Hydration status may play a role in age-related changes, as many older adults have insufficient fluid intake and are more susceptible to the effects of diminished fluid intake on salivary output.106 Several scientific reports show decreases in the number and salivary flow of minor salivary glands with age.107–109 Aging salivary glands are more adversely affected by antisialagogues.110,111 Rodents show an age-dependent decrease in flow as well as a decrease in the production of mucins and amylase.112 There are contradictory studies in the literature regarding the effects of age on the rate of stimulated and unstimulated salivary flow in the major salivary glands.97,105,113 One of the expected major problems in studying this issue is that salivary flow rates vary by as much as 45% in the same individual at different times.114
Human parotid saliva has been shown to have less electrolyte content and therefore decreased osmolality with age.115 IgA increases with age.105,116,117 There also appears to be a decrease in histatins with age, which may leave patients more susceptible to fungal infections.118 Mucin content increases as amylase decreases, creating more viscous saliva that can be difficult to clear from the throat.119
Finally, there has been speculation that estrogen deficits may produce symptoms of xerostomia in older adults, but replacement therapies including estrogen have not been shown to have any significant effect.116,120 In summary, there are structural changes in salivary glands that occur over the course of the human life span, but they may not be sufficiently profound to significantly affect salivary output. However, these histomorphological changes predispose salivary glands to clinically significant qualitative and quantitative decrements when older adults experience multiple medical disorders, are exposed to head and neck radiotherapy, or take multiple prescription and nonprescription medications. Accordingly, the older adult must take precautions to the potentially deleterious oropharyngeal effects of salivary dysfunction.
Future Physiologic Research
Pharmacological research into medications that selectively promote salivary secretion may depend more on regulating ion channels and second messengers rather than simply stimulating the parasympathetic nervous system, as is currently done with pilocarpine and its derivatives.121
The use of gene transfer technology in vivo has demonstrated salivary endocrine pathways. Salivary glands can be used as target tissues for systemic applications of gene therapeutics. Salivary glands are advantageous for this application because intraoral delivery of a vector is possible through the excretory duct, and salivary gland encapsulation prevents vector dissemination.122
Saliva meets the demand for a noninvasive, accessible, and efficient diagnostic medium. Salivary transcriptome diagnostics relies on the detection of ribonucleic acid (RNA) in saliva. Distinct cancer-associated molecules in saliva have been accurately measured to distinguish between healthy people and those diagnosed with oral squamous cell carcinoma. One report documents that distinct patterns of cancer-linked messenger RNA can be measured in saliva and indicate the presence of a developing tumor.123
REFERENCES
1. Gibson, M. The prenatal human submandibular gland: a histological, histochemical and ultrastructural study. Anat Anz 1983; 153 (1): 91–105
2. Bernfield, M Banerjee, S Cohn, R. Dependence of salivary
epithelial morphology and branching morphogenesis upon acid mucopolysaccharide-protein (proteoglycan) at the epithelial surface. J Cell Biol 1972; 52: 674–689
3. Kadoya, Y. Antibodies against domain E3 of laminin-1 and integrin alpha 6 subunit perturb branching epithelial morphogeneisis of submandibular gland, but by different modes. J Cell Biol 1995; 129: 521–534
4. Sakai, T Larsen, M Yamada, K. Fibronectin requirement in branching morphogenesis. Nature 2003; 423: 876–881
5. Lee, S Lim, C Chi, J, et al. Immunohistochemical distribution of MAM-3 and MAM-6 antigens in developing salivary glands of the human fetus. Histochem J 1991; 23: 355–360
6. Espin-Ferra, J Merida-Velasco, J Garcia-Garcia, JD Sanchez- Montesinos, I Barranco-Zafra, RJ. Relationships between the parotid gland and the facial nerve during human development. J Dent Res 1991; 70: 1035–1040
7. Johns, ME. The salivary glands: anatomy and embryology. Otolaryngol Clin North Am 1977; 10 (2): 261–271
8. Kontis, TC Johns, ME. Salivary gland anatomy and physiology. In: Meyerhoff, WL Rice, DH eds. Otolaryngology: Head and Neck Surgery. Philadelphia: WB Saunders; 1992: 553–569
9. Ferraris, ME Samar, ME Busso, C Avila, R Ferraris, R de Fabro, S. Prenatal development of human palatine glands: a structural and cytochemical study. Acta Odontol Latinoam 1993; 7: 23–29
10. Arglebe, C. Biochemistry of human saliva. Adv Otorhinolaryngol 1981; 26: 97–234
11. Garrett, JR. Recent advances in physiology of salivary glands. Br Med Bull 1975; 31 (2): 152–155
12. Chilla, R. Sialadenosis of the salivary glands of the head: studies on the physiology and pathophysiology of parotid secretion. Adv Otorhinolaryngol 1981; 26: 1–38
13. Emmelin, N. Nerve interactions in salivary glands. J Dent Res 1987; 66 (2): 509–517
14. Garrett, JR. Innervation of salivary glands: neurohistological and functional aspects. In: Sreebny, LM, ed. The Salivary System. Boca Raton, FL: CRC Press; 1987: 69–93
15. Stumpf, WE. Histochemical characteristics and significance of cell receptors in biology and pathology. Acta Histochem Suppl 1984; 29: 23–33
16. Garrett, JR. The proper role of nerves in salivary secretion. J Dent Res 1987; 66 (2): 387–397
17. Williams, JA. Regulatory mechanisms in pancreas and salivary acini. Annu Rev Physiol 1984; 46: 361–375
18. Peterson, OH. The Electrophysiology of Gland Cells. New York: Academic Press; 1980
19. Shannon, IL Suddick, RP Dowd, FJ Jr. Saliva: composition and secretion. Monogr Oral Sci 1974; 2: 1–103
20. Palade, G. Intracellular aspects of the process of protein synthesis. Science 1975; 189: 347–358
21. Izutsu, KT. Salivary electrolytes and fluid production in health and disease. In: Sreebny, LM, ed. The Salivary System. Boca Raton, FL: CRC Press; 1987: 95–122
22. Findlay, I. Patch-clamp study of potassium channels and whole-cell currents in acinar cells of the mouse lacrimal gland. J Physiol 1984; 350: 179–195
23. Ishikawa, Y Ishida, H. Aquaporin water channel in salivary glands. Jpn J Pharmacol 2000; 83: 95–101
24. Cunning, DM Lipke, N Wax, MK. Significance of unilateral submandibular gland excision on salivary flow in noncancer patients. Laryngoscope 1998; 108 (6): 812–815
25. Mandel, ID. Sialochemistry in diseases and clinical situations af-fecting salivary glands. Crit Rev Clin Lab Sci. 1980; 12 (4): 321–366
26. Humphrey, SP Williamson, RT. A review of saliva: normal composition, flow and function. J Prosthet Dent 2001; 85 (2): 162–169
27. Batsakis, JG. Salivary gland physiology. In: Otolaryngology/Head and Neck Surgery. St Louis: CV Mosby; 2000: 1210–1222
28. Moro, I Umemura, S Crago, S Mestecky, J. Immunohistochemical distribution immunoglobulins, lactoferrin and lysozyme in human minor salivary glands. J Oral Pathol 1984; 13 (2): 97–104
29. Barka, T. Biologically active polypeptides in submandibular glands. J Histochem Cytochem 1980; 28: 836–859
30. Amsterdam, A Ohad, I Schramm, M. Dynamic changes in the ultrastructure of the aciner cell of the rat parotid gland during the secretory cycle. J Cell Biol 1969; 41: 753–773
31. Dawes, C. The effects of flow rate and duration of stimulation on the concentrations of protein and the main electrolytes in human parotid saliva. Arch Oral Biol 1969; 14 (3): 277–294
32. Navazesh, M Christensen, C Brightman, V. Clinical criteria for the diagnosis of salivary gland hypofunction. J Dent Res. 1992; 71 (7): 1363–1369
33. Saito, M Umeda, M Fujiwara, K, et al. Functional analysis of human parotid gland in vivo using the (1) H MRS MT effect. NMR Biomed 2002; 15 (6): 416–421
34. Ghezzi, EM Lange, LA Ship, JA. Determination of variation of stimulated salivary flow rates. J Dent Res 2000; 79 (11): 1874–1878
35. Anderson, DJ Hector, MP Linden, RW. The effects of unilateral and bilateral chewing, empty clenching and simulated bruxism on the masticatory-parotid salivary reflex in man. Exp Physiol 1996; 81 (2): 305–312
36. Gemba, H Teranaka, A Takemura, K. The influences of emotion upon parotid secretion in humans. Neurosci Lett. 1996; 211 (3): 159–162
37. Ship, J Fox, P Baum, B. Normal salivary gland function: how much saliva is enough? J Am Dent Assoc 1991; 122: 63–69
38. Edgar, W. Saliva: its secretion, composition, and functions. Br Dent J 1992; 172: 305–312
39. Navazesh, M Christensen, C Brightman, V. Clinical criteria for the diagnosis of salivary gland hypofunction. J Dent Res. 1992; 71 (7): 1363–1369
40. Dawes, C. Physiological factors affecting salivary flow rate, oral sugar clearance and the sensation of dry mouth in man. J Dent Res 1987; 66: 648–653
41. Chaushu, G Dori, S Taichler, S Kronenberg, J Talmi, Y. Salivary flow dynamics after parotid surgery: a preliminary report. Otolaryngol Head Neck Surg 2001; 124 (3): 270–273
42. Castle, D. Cell biology of salivary protein secretion. In: Dobrosielski- Vergona, K, ed. Biology of Salivary Glands. Boca Raton, FL: CRC Press; 1993: 81–104
43. Ship, J Grushka, M Lipton, J Mott, A Sessle, B Dionne, R. An update on burning mouth syndrome. J Am Dent Assoc 1995; 126 (7): 842–853
44. Niedermeier, W Huber, M Fischer, D, et al. Significance of saliva for the denture-wearing population. Gerodontology. 2000; 17 (2): 104–118
45. Grushka, M Epstein, J Gorsky, M. Burning mouth syndrome. Am Fam Physician 2002; 65 (4): 615–620
46. Chimenos-Kustner, E Marques-Soares, M. Burning mouth and saliva. Med Oral 2002; 7: 244–253
47. Spielman, A. Interaction of saliva and taste. J Dent Res 1990; 69: 838–843
48. Haraszthy, G Collins, A Bergey, E. Identification of salivary proteins inhibiting herpes simplex virus 1 replication. Oral Microbiol Immunol 1995; 10: 54–59
49. Mandel, ID. The functions of saliva. J Dent Res 1987; 66: 623–627
50. Pollock, JJ Lotardo, S Gavai, R Grossbard, BL. Lysosyme-proteaseinorganic monovalent anion lysis of oral bacterial strains in buffers and stimulate whole saliva. J Dent Res. 1987; 66 (2): 467–474
51. Carlen, A Olsson, J Ramberg, P. Saliva mediated adherence, aggregation and prevalence in dental plaque of Streptococcus mutans, Steptococcus sanquis, and Actinomyces spp, in young and elderly humans. Arch Oral Biol 1996; 41 (12): 1133–1140
52. Levine, MJ. Salivary macromolecules: a structure/function synopsis. Ann NY Acad Sci 1993; 694: 11–16
53. Bolscher, J Nazmi, K Ran, L, et al. Inhibition of HIV-1 IIIB and clinical isolates by human parotid, submandibular, sublingual and palatine saliva. Eur J Oral Sci 2002; 110: 149–156
54. Atkinson, J Travis, W Pillemer, S Bermudez, D Wolff, A Fox, P. Major salivary gland function in primary Sjögren’s syndrome and its relationship to clinical features. J Rheumatol 1990; 17: 318–322
55. Fox, R Stern, M Micholson, P. Update in Sjögren syndrome. Curr Opin Rheumatol 2000; 12 (5): 391–398
56. Vitali, C Bombardieri, S Moutsopoulos, H, et al. Classification criteria for Sjögren’s syndrome: a revised version of the European criteria proposed by the American-European Consensus Group. Ann Rheum Dis 2002; 61 (6): 554–558
57. Pillemer, S Matteson, E Jacobbson, L, et al. Incidence of physiciandiagnosed primary Sjögren syndrome in residents of Olmsted County, Minnesota. Mayo Clin Proc 2001; 76 (6): 593–599
58. Kamisawa, T Egawa, N Inokuma, S, et al. Pancreatic endocrine and exocrine function and salivary gland function in autoimmune pancreatitis before and after steroid therapy. Pancreas 2003; 27 (3): 235–238
59. Kamisawa, T Egawa, N Sakaki, N, et al. Salivary gland involvement in pancreatitis of various etiologies. Am J Gastroenterol 2003; 98 (2): 323–326
60. Changlai, S Chen, W Chung, C Chiou, S. Objective evidence of decreased salivary function in patients with autoimmune thyroiditis. Nucl Med Commun 2002; 23 (1): 1029–1033
61. Persson, RE Persson, GR Kiyak, HA Powell, LV. Oral health and medical status in dentate low-income older persons. Spec Care Dentist 1998; 18 (2): 70–77
62. Rivera, H Nikitakis, NG Castillo, S Siavash, H Papadimitriou, J Sauk, J. Histopathological analysis and demonstration of EBV and HIV p-24 antigen but not CMV expression in labial minor salivary glands of HIV patients affected by diffuse infiltrative lymphocytosis syndrome. J Oral Pathol Med 2003; 32 (7): 431–437
63. Moore, P Guggenheimer, J Etzel, K Weyant, R Orhard, T. Type 1 diabetes mellitus, xerostomia, and salivary flow rates. Oral Surg Oral Med Oral Pathol Oral Radiol Endod 2001; 92 (3): 281–291
64. Chavez, E Taylor, G Borrell, L Ship, J. Salivary function and glycemic control in older persons with diabetes. Oral Surg Oral Med Oral Pathol Oral Radiol Endod 2000; 89: 305–311
65. Streckfus, C Wu, A Ship, J Brown, L. Stimulated parotid gland salivary flow rates in normotensive, hypertensive, and hydrochlorothiazide- medicated African Americans. J Oral Pathol Med 1994; 23: 280–283
66. Kaya, M Cermik, TF Uston, F Sen, S Berkarda, S. Salivary function in patients with chronic renal failure undergoing hemodialysis. Ann Nucl Med 2002; 16 (2): 117–120
67. Dutta, SK Dukehart, M Narang, A Latham, PS. Functional and structural changes in parotid glands of alcoholic cirrhotic patients. Gastroenterology 1989; 96: 510–518
68. Nordgarden, H Storhaug, K Lyngstadaas, SP Jensen, JL. Salivary gland function in persons with ectodermal dysplasias. Eur J Oral Sci 2003; 111 (5): 371–376
69. Melvin, JE. Chloride channels and salivary gland function. Crit Rev Oral Biol Med 1999; 10 (2): 199–209
70. Henson, BS Inglehart, MR Eisbruch, A Ship, J. Preserved salivary output and xerostomia-related quality of life in head and neck cancer patients receiving parotid-sparing radiotherapy. Oral Oncol 2001; 37 (1): 84–93
71. Epstein, J Emerton, S Kolbinson, DA, et al. Quality of life and oral function following radiotherapy for head and neck cancer. Head Neck 1999; 21 (1): 1–11
72. Eisbruch, A Ten Haken, R Kim, H Marsh, L Ship, J. Dose, volume, and function relationships in parotid salivary glands following conformal and intensity modulated irradiation of head and neck cancer. Int J Radiat Oncol Biol Phys 1999; 45 (3): 577–587
73. Eisbruch, A Ship, J Dawson, L, et al. Salivary gland sparing and improved target irradiation by conformal and intensity modulated irradiation of head and neck cancer. World J Surg 2003; 27 (7): 832–837
74. Smith, RG Burtner, AP. Oral side-effects of the most frequently prescribed drugs. Spec Care Dentist 1994; 14 (3): 96–102
75. Sreebny, L Schwartz, S. A Reference Guide to Drugs and Dry Mouth, 2nd ed. Gerontol 1997; 14 (1): 33–47
76. Nederfors, T. Xerostomia: prevalence and pharmacotherapy: with special reference to beta-adrenoceptor antagonists. Swed Dent J Suppl 1996; 116: 1–70
77. Nederfors, T Twetman, S Dahlof, C. Effects of the thiazide diuretic bendroflumethiazide on salivary flow rate and composition. Scand J Dent Res 1989; 97 (6): 520–527
78. Streckfus, C Wu, A Ship, J Brown, L. Comparison of stimulated parotid salivary gland flow rates in normotensive and hypertensive persons. Oral Surg Oral Med Oral Pathol 1994; 77: 615–619
79. Loesche, W Bromberg, J Terpenning, M, et al. Xerostomia, xerogenic medications and food avoidances in selected geriatric groups. J Am Geriatr Soc 1995; 43 (4): 401–407
80. Nederfors, T Dahlof, C Ericsson, T Twetman, S. Effects of the antihypertensive drug captopril on human salivary secretion rate and composition. Eur J Oral Sci 1995; 103 (6): 351–354
81. Field, E Longman, L Bucknall, R Kaye, S Higham, S Edgar, W. The establishment of a xerostomia clinic: a prospective study. Br J Oral Maxillofac Surg 1997; 35 (2): 96–103
82. Pajukoski, H Meurman, H Snellman-Grohn, S Keinanan, S Sulkava, R. Salivary flow and composition in elderly patients referred to an acute care geriatric ward. Oral Surg Oral Med Oral Pathol Oral Radiol Endod 1997; 84 (3): 265–271
83. Bergdahl, M Bergdahl, J. Low unstimulated salivary flow and subjective oral dryness: association with medication, anxiety, depression, and stress. J Dent Res 2000; 79 (9): 1652–1658
84. Meurman, JH Laine, P Keinanan, S Pyrhonen, S Teerenhovi, L Linquist, C. Five-year follow-up of saliva in patients treated for lymphomas. Oral Surg Oral Med Oral Pathol Oral Radiol Endod 1997; 83 (4): 447–452
85. Nagler, RM Gez, E Rubinov, R. The effect of low-dose interleukin- 2-based immunotherapy on salivary function and composition in patients with metastatic renal cell carcinoma. Arch Oral Biol 2001; 46 (6): 487–493
86. Nelson, JD Friedlaender, M Yeatts, R, et al. Oral pilocarpine for symptomatic relief of keratoconjunctivitis sicca in patients with Sjögren’s syndrome: the MGI PHARMA Sjögren’s Syndrome Study Group. Adv Exp Med Biol 1998; 438: 979–983
87. Vivino, F Al-Hashimi, I Khan, Z, et al. Pilocarpine tablets for the treatment of dry mouth and dry eye symptoms in patients with Sjögren syndrome: a randomized, placebo-controlled, fixed-dose, multicenter trial. P92–01 Study Group. Arch Intern Med 1999; 159 (2): 174–181
88. Johnson, J Ferretti, G Nethery, W, et al. Oral pilocarpine for postirradiation xerostomia in patients with head and neck cancer. N Engl J Med 1993; 329 (6): 390–395
89. Fife, R Chase, W Dore, R, et al. Cevimeline for the treatment of xerostomia in patients with Sjögren syndrome: a randomized trial. Arch Intern Med 2002; 162 (11): 1293–1300
90. Petrone, D Condemi, J Fife, R Gluck, O Cohen, S Dalgin, P. A double-blind, randomized, placebo-controlled study of cevimeline in Sjögren’s syndrome patients with xerostomia and keratoconjunctivitis sicca. Arthritis Rheum. 2002; 46 (3): 748–754
91. Borg, M Hirst, F. The role of radiation therapy in the management of sialorrhea. Int J Radiat Oncol Biol Phys. 1998; 41 (5): 1113–1119
92. Scott, J. Structure and function in aging human salivary glands. Gerodontology 1986; 5: 149–158
93. Billings, RJ Proskin, HM Moss, ME. Xerostomia and associated factors in a community-dwelling adult population. Community Dent Oral Epidemiol 1996; 24 (5): 312–316
94. Jones, RE Ship, JA. Major salivary gland flow rates in young and old, generally healthy African Americans and whites. J Natl Med Assoc 1995; 87 (2): 131–135
95. Kim, SK Allen, ED. Structural and functional changes in salivary glands during aging. Microsc Res Tech 1994; 28: 243–253
96. Ship, JA Pillemer, SR Baum, BJ. Xerostomia and the geriatric patient. J Am Geriatr Soc 2002; 50 (3): 535–543
97. Ship, JA Nolan, NE Puckett, SA. Longitudinal analysis of parotid and submandibular salivary flow rates in healthy, different aged adults. J Gerontol A Biol Sci Med Sci 1995; 50 (5): M285–M289
98. Wu, AJ Ship, JA. A characterization of major salivary gland flow rates in the presence of medications and systemic disease. Oral Surg Oral Med Oral Pathol 1993; 76: 301–306
99. Fischer, D Ship, J. Effect of age on variability of parotid salivary gland flow rates over time. Age Ageing 1999; 28 (6): 557–561
100. Segawa, A Loffredo, F Puxedda, R Yamashina, S Testa, R Riva, A. Cell biology of human salivary secretion. Eur J Morphol 2000; 38 (4): 237–241
101. Field, EA Fear, S Higham, S, et al. Age and medication are significant risk factors for xerostomia in an English population, attending general dental practice. Gerodontology. 2001; 18 (1): 21–24
102. Hochberg, MC Tielsch, J Munoz, B Bandeen-Roche, K West, SK Schein, OD. Prevalence of symptoms of dry mouth and their relationship to saliva production in community dwelling elderly: the SEE project. J Rheumatol 1998; 25 (3): 486–491
103. Nederfors, T Isaksson, R Mornstad, H. Dahlof C. Prevalence of perceived symptoms of dry mouth in an adult Swedish population—relation to age, sex and pharmacotherapy. Community Dent Oral Epidemiol 1997; 25 (3): 211–216
104. Yeh, CK Johnson, DA. Dodds MW. Impact of aging on human salivary gland function: a community based study. Aging (Milano) 1998; 10 (5): 421–428
105. Wu, AJ Ship, JA Baum, BJ. Extended stimulated parotid and submandibular secretion in a healthy young and old population. J Gerontol A Biol Sci Med Sci 1995; 50A (1): M45–M48
106. Ship, JA Fischer, DJ. The relationship between dehydration and parotid salivary gland function in young and older healthy adults. J Gerontol A Biol Sci Med Sci 1997; 52 (5): M310–M319
107. Bretz, WA Loesche, WJ Chen, YM Schork, MA Dominquez, B Grossman, N. Minor salivary gland secretion in the elderly. Oral Surg Oral Med Oral Pathol Oral Radiol Endod. 2000; 89 (6): 696–701
108. Dayan, D Vered, M. Age related changes in proliferative markers in labial salivary glands: a study of argyrophilic nucleolar organizer regions and Ki-67. Exp Gerontol 2002; 37 (6): 841–850
109. Vered, M Buchner, A Haimovici, E Hiss, Y Dayan, D. Focal lymphocytic infiltration in aging human palatal salivary glands: a comparative study with labial salivary glands. J Oral Pathol Med 2001; 30 (1): 7–11
110. Ghezzi, EM Ship, JA. Aging and secretory reserve capacity of major salivary glands. J Dent Res 2003; 82 (10): 844–848
111. Patel, PS Ghezzi, E Ship, JA. Xerostomia complaints induced by anti-sialogogues in healthy young vs. older adults. Spec Care Dentist 2001; 21 (5): 176–181
112. Dobrosielki-Bergona, K. Age-related changes in the function of salivary. In: Dobrosielski-Vergona, ed. Biology of the Salivary Glands. Boca Raton, FL: CRC Press; 1993: 431–439
113. Ghezzi, EM Wagner-Lange, LA Shork, M, et al. Longitudinal influence of age, menopause, hormone replacement therapy, and other medications on parotid flow rates in healthy women. J Gerontol A Biol Sci Med Sci 2000; 55 (1): M34–M42
114. Ghezzi, EM Lange, LA Ship, JA. Determination of variation of stimulated salivary flow rates. J Dent Res 2000; 79 (11): 1874–1878
115. Chauncey, HH Feller, RP Kapur, KK. Longitudinal age-related changes in human parotid saliva composition. J Dent Res 1987; 66 (2): 599–602
116. Childers, NK Greenleaf, C. Effect of age on immunoglobulin subclass distribution in human parotid saliva. Oral Microbiol Immunol 2003; 18 (5): 298–301
117. Percival, RS Marsh, PD Challacombe, S. Age-related changes in salivary antibodies to commensal oral and gut biota. Oral Microbiol Immunol 1997; 12 (1): 57–63
118. Johnson, DA Yeh, CK Dodds, MW. Effect of donor age on the concentrations of histatins in human parotid and submandibular/ sublingual saliva. Arch Oral Biol 2000; 45 (9): 731–740
119. Astor, FC Hanft, KL Ciocon, JO. Xerostomia: a prevalent condition in the elderly. Ear Nose Throat J 1999; 78 (7): 476–479
120. Streckfus, CF Baur, U Brown, L, et al. Effects of estrogen status and ageing on salivary flow rates in healthy Caucasian women. Gerontology 1998; 44 (1): 32–39
121. Turner, JT Landon, LA Gibbons, S Talamo, B. Salivary gland P2 nucleotide receptors. Crit Rev Oral Biol Med 1999; 10 (2): 210–224
122. Voutetakis, A Wang, J Baum, B. Utilizing endocrine secretory pathways in salivary glands for systemic gene therapeutics. J Cell Physiol 2004; 199 (1): 1–7
123. Li, Y St. John, M Zhou, X, et al. Salivary transcriptome diagnostics for oral cancer detection. Clin Cancer Res 2004; 10: 8442–8450
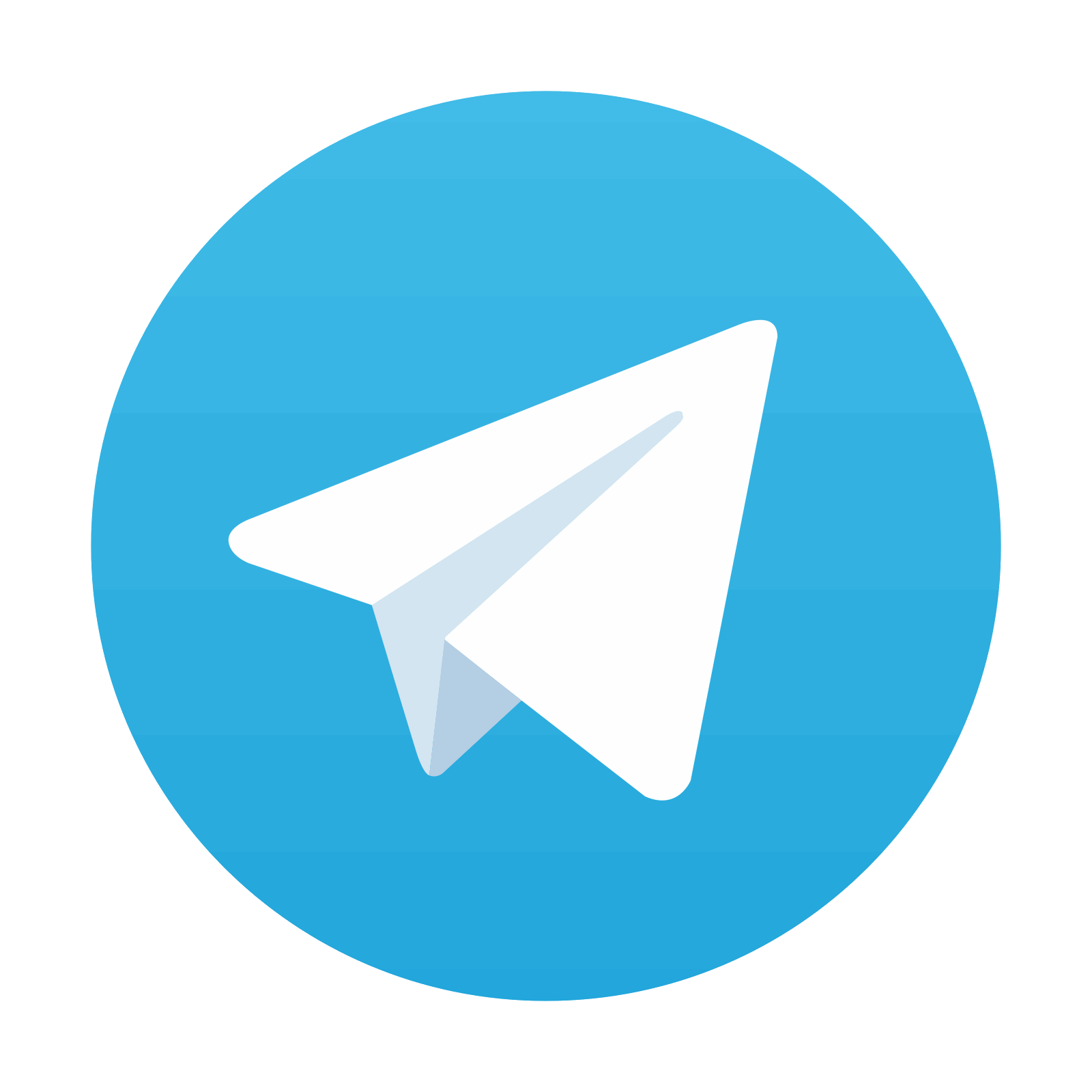
Stay updated, free dental videos. Join our Telegram channel

VIDEdental - Online dental courses
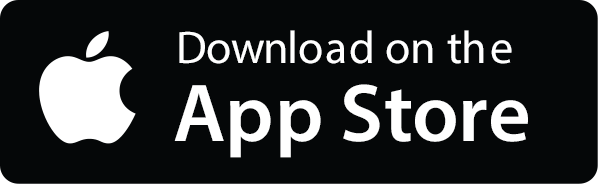
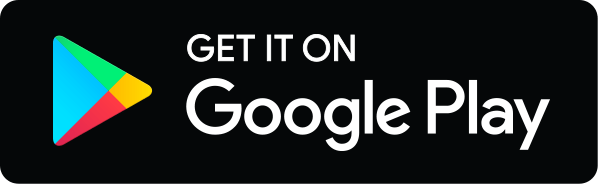