Highlights
- •
Pre-heating does not reduce polymerization stress of resin composite restorations.
- •
Thio-urethane addition increases resin composite degree of conversion.
- •
Thio-urethane addition reduces resin composite polymerization stress.
Abstract
Objective
The aim of this in vitro study was to test the effect of different composite modulation protocols (pre-heating, light-curing time and oligomer addition) for bulk filling techniques on resin polymerization stress, intra-pulpal temperature change and degree of conversion.
Methods
Class I cavities (4 mm depth × 5 mm diameter) were prepared in 48 extracted third molars and divided in 6 groups. Restorations were completed with a single increment, according to the following groups: (1) Filtek Z250XT (room temperature – activated for 20 s); (2) Filtek Z250XT (at room temperature – activated for 40 s); (3) Filtek Z250XT (pre-heated at 68 °C – activated for 20 s); (4) Filtek Z250XT (pre-heated at 68 °C – activated for 40 s); (5) Filtek BulkFill (at room temperature – activated for 20 s); (6) Filtek Z250XT (modified by the addition of a thio-urethane oligomer at room temperature – activated for 40 s). Acoustic emission test was used as a real-time polymerization stress (PS) assessment. The intra-pulpal temperature change was recorded with a thermocouple and bottom/top degree of conversion (DC) measured by Raman spectroscopy. Data were analyzed with one-way ANOVA/Tukey’s test ( α = 5%).
Results
Pre-heating the resin composite did not influence the intra-pulpal temperature ( p = 0.077). The thio-urethane-containing composite exhibited significantly less PS, due to a lower number of acoustic events. Groups with pre-heated composites did not result in significantly different PS. Filtek BulkFill and the thio-urethane experimental composite presented significantly higher DC.
Significance
Resin composite pre-heating was not able to reduce polymerization stress in direct restorations. However, thio-urethane addition to a resin composite could reduce the polymerization stress while improving the DC.
1
Introduction
Dental resin composites are widely used for adhesive restorative procedures, more so nowadays due to the significant improvement in their physical and mechanical properties compared to the early materials . However, volumetric shrinkage is still an inherent drawback of the polymerization of the resin matrix . The decrease in free volume around monomers during the polymerization reaction causes macroscopic shrinkage of the material . In this way, volumetric shrinkage induces stress at the surface to which the resin composite is being adapted . Although volumetric shrinkage is related to polymerization stress, other factors including elastic modulus, gelation point and final conversion can also influence polymerization stress . The effect of the polymerization stress generated during placement of direct restorations can lead to microleakage, cuspal deflection, cuspal fractures, and marginal defects . Therefore, reducing polymerization stress through multiple protocols and techniques during composite resin placement or new material development is a constant research aim.
Pre-heating of composite resins has been shown to modify some of the material properties. At higher temperatures resin viscosity is reduced and higher conversion can be achieved . In addition, monomer mobility is increased, which favors propagation and termination events, leading to higher polymerization rate . Some other practical strategies might include alternative curing protocols, such as increasing light exposure time . As a result of higher conversion or extended curing time, an unfavorable increase in polymerization stress or intrapulpar temperature could be expected . Despite this, significant stress relaxation was observed when a resin composite is heated to 60 °C . Stress relaxation during conversion is possible due to viscous flow or chain relaxation . Moreover, as conversion increases, better mechanical properties are also expected . Therefore, there is a possibility that pre-heated resin composites could be used in bulk placement with reduced polymerization stress.
The continuous search for a material that is simply and rapidly placed with reduced polymerization shrinkage has led to the development of bulk-fill composite resins: a range of materials with several changes in the chemistry and concentration of monomers, addition of new photoinitiators, modified inorganic content and increased translucency, which allowed those materials to be placed and photo-polymerized in a single layer of 4–5 mm thickness .
Another composite modulation protocol includes thio-urethane additives in dental resins, with the aim of reducing polymerization stress and increasing toughness in crosslinked materials. Thio-urethanes are oligomers with pending thiols from the backbone, through which they are able to establish covalent interactions with a methacrylate matrix . The multiple thiol functionalities pendant from the oligomer undergo chain-transfer events with the methacrylate, creating crosslinks while at the same time leading to delayed network formation. This delayed gelation/vitrification, in turn, leads to increased final conversion and viscous flow, ultimately leading to lower stress . Indeed, studies testing experimental and commercial composites modified with thio-urethanes have reported significant stress reduction combined with increased degree of conversion and mechanical properties . A recent study also suggested filler functionalization with thio-urethane, which could be an alternative for improving mechanical properties, conversion and reducing polymerization stress . Although several studies reported important properties improvement with these new additives, no studies have tested them as bulk-fill materials against commercially available materials. If thio-urethane modified composites present similar or higher stress reduction than commercial bulk-fill composites, a new class of low-stress materials with even higher mechanical properties could be achieved. Testing a thio-urethane modified composite against a commercial bulk-fill composite in high C-factor (Class I and II) cavities should help clarify if this mechanism of stress reduction works better than the ones available on the market.
Therefore, the aim of this in vitro study was to evaluate the effect of different modulation protocols (i.e. using a pre-heated resin and a thio-urethane modified version of this material) for bulk techniques on resin polymerization stress, intra-pulpal temperature change and degree of conversion. A conventional resin composite was used at room temperature and pre-heated at 68 °C and compared to a high viscosity bulk-fill resin composite. The tested hypotheses were: (1) pre-heating would reduce the polymerization stress and increase the degree of conversion; (2) there is no difference on the polymerization stress reduction between a thio-urethane modified resin composite and a bulk-fill resin composite.
2
Materials and methods
2.1
Thio-urethane synthesis and filler silanization
A thio-urethane was synthetized for this study by combining 4,4-methylene bis(cyclohexyl isocyanate), trimethylol-tris-3-mercaptopropionate and 3-(triethoxysilyl) propyl isocyanate (2:1:1 mol ratio) in 60 ml of methylene chloride at room temperature. Triethylamine was used to catalyze the reaction and oligomers were purified by precipitation in hexane. All chemicals were purchased from Sigma Aldrich (Madison, WI, USA). A rotary evaporator (R-100, Buchi Corporation, New Castle, DE, USA) was used for solvent removal. Solvent evaporation was done at 32 °C for 2:30 h. Formation of thio-urethane bonds and absence of starting materials was verified by H-NMR and mid-IR spectroscopy as previously described .
A glass filler was silanized with the synthetized thio-urethane. The silanization process was performed in an ethanol/distilled water solution (80%/20%). Glacial acetic acid was added to adjust the pH to 4.5. After this, 2 wt% of the oligomer was added to the ethanol/water silanizing solution together with 0.7 μm unsilanized barium-aluminum-borosilicate-glass filler (GM27884 – Schott AG, Landshut – Germany). The solution was kept under mechanical agitation for 24 h, filtered and dried for 4 days in an oven at 37 °C. A thio-urethane-silanized filler was obtained for the experimental resin composite.
2.2
Thio-urethane-modified composite
One commercial resin composite (Z250XT, 3M ESPE, St. Paul, MN, USA) was used as the base for thio-urethane addition. Considering that this composite has an organic content of 18 wt%, the oligomer was added in relation to the mass of resin matrix. Previous studies have reported good properties improvement with the addition of 20 wt% of thio-urethane in relation to the resin matrix weight , so that concentration was selected for this study. Since adding the thio-urethane leads to an overall decrease of the filler loading, additional filler silanized with the thio-urethane was incorporated so that the final material presented 82 wt% filler loading, to achieve similar loading to the original commercial material.
2.3
Cavity preparation
Forty-eight freshly extracted human third molars were used in this study. Teeth were stored refrigerated in 0.5% chloramine-T solution before use. All teeth were extracted due to therapeutic reasons and the protocols used were approved by the local ethics committee review board (46562215.6.0000.5347).
Roots were removed using a low-speed diamond saw by performing a cut 3 mm below the cement-enamel junction. Pulpal tissue was gently removed with a dentin excavator to clear the pulpal chamber. After this, a small hole was made with a round diamond bur next to the cement-enamel junction to create a lateral access to the pulpal chamber. Occlusal 4-mm deep class I cavities (C-factor = 5) were prepared with a large round end taper diamond bur (#3131 – KG Sorensen, Cotia, SP, Brazil). A 5-mm diameter round template was placed over the center of the occlusal surfaces to establish the conformation of the cavity preparation. Cavities were manually prepared with a high-speed handpiece using water spray refrigeration and the dimensions were checked with a probe during the preparation. Teeth were numbered from 1 to 48 and randomly assigned by drawing to one of the 6 experimental groups.
2.4
Polymerization stress (PS)
Before the restoration procedure samples were attached to a WDI differential acoustic emission sensor from Physical Acoustics Corporation (PAC) ( Fig. 1 ) in order to determine the polymerization stress of the composites. Root surfaces were attached to the sensors with cyanoacrylate adhesive. Three channels from a PAC Disp 16C acoustic emission system were used to record acoustic events generated by PS. Each channel was connected to a different acoustic sensor of the same model and pre-amplified with a 60 dB gain. Sensor 1 and 2 were used as guard sensors to detect false calls: the first from a sensor with a free surface and the second received the signal from a sensor with a non-testing tooth. Sensor 3 received the signal from the sensor where the testing tooth was attached. After the test, signals recorded from second and third sensors were used to compare and remove false signals from the environment. A threshold of 32 dB was used for all the channels. The number of acoustics events (AE) and their respective amplitudes were recorded from the start of light curing until 20 min after. The resulting signals were filtered and reported as total AE and AE higher than 60 dB (AE > 60 dB).

2.5
Intra-pulpal temperature
The remaining dentin thickness at the pulpal floor was measured with a caliper at 3 different spots. A mean remaining dentin thickness was calculated for each tooth and group to avoid different test conditions. The pulpal chambers were filled with thermal conducting paste (Implastec Eletrochemistry, Votorantim, SP, Brazil). A K-type thermocouple sensor connected to a CPM-45 thermometer (Contemp, São Caetano do Sul, SP, Brazil) was inserted inside the pulpal chamber through the lateral hole near the cement-enamel junction. Tests were conducted in a controlled temperature room with constant baseline temperatures. Temperature changes (Δ t ) were recorded between the start of light curing and the maximum temperature reached. Temperature changes during adhesive activation were also recorded.
2.6
Restorative procedures
After being attached to the AE sensor, teeth were restored according to the different experimental groups. Description of the materials used are detailed in Table 1 . A universal adhesive (SingleBond Universal, 3M ESPE, St Paul, MN, USA) was used in self-etch mode in dentin and in selective etch mode in enamel, for 30 s (Scotchbond Universal Etchant, 3M ESPE, St Paul, MN, USA). Enamel was washed with water spray for 30 s and cavities were dried with cotton pellets. The adhesive was applied for 20 s with active scrubbing. Solvent evaporation was performed with 10 s of gentle air. A LED curing light (Emitter, Schuster, RS, Brazil) was used for 10 s, at 1 mm from the cavosuperficial border. Light irradiance was checked with a radiometer (LM-1, Woodpecker, Guilin, China) throughout the study. Constant light irradiance at the tip of the instrument (1300 mW/cm 2 ) was observed during all the experiment. Two composite materials (Filtek Z250XT and Filtek BulkFill, 3M ESPE, St Paul, MN, USA) were divided into 6 experimental groups. Cavities were bulk filled according to the following groups:
- 1.
Filtek Z250XT at room temperature – activated for 20 s.
- 2.
Filtek Z250XT at room temperature – activated for 40 s.
- 3.
Filtek Z250XT at 68 °C – activated for 20 s.
- 4.
Filtek Z250XT at 68 °C – activated for 40 s.
- 5.
Filtek BulkFill at room temperature – activated for 20 s (according to manufacturer instructions).
- 6.
Filtek Z250XT w/thio-urethane at room temperature – activated for 40 s.
Product | Type | Manufacturer | Composition |
---|---|---|---|
Scotchbond Universal Etchant | Phosphoric acid etching gel | 3M ESPE | 32% by weight phosphoric acid, water, poly(vinyl alcohol) |
SingleBond Universal | Universal adhesive | 3M ESPE | HEMA; bis-GMA; dimethacrylate resins; ethanol; silane treated silica; water; 2-propenoic acid; 2-methyl-; reaction products with 1,10-decanediol and phosphorous oxide; copolymer of acrylic and itaconic acid; CQ; 2,6-di-tert-butyl-P-cresol |
Filtek Z250XT (also known as Filtek Supreme Ultra and Filtek Supreme XTE) |
Nano filled conventional composite resin | 3M ESPE | Bis-GMA, UDMA,TEGDMA, bis-EMA, silanated silica, silanated zirconia, photoinitiators |
Filtek BulkFill Restorative | High-viscosity nano filled bulk-fill composite resin | 3M ESPE | AFM, AUDMA, UDMA, DDDMA, Ytterbium trifluoride, nonaggregated silica, nonaggregated zirconia, zirconia/silica clusters |
For groups with pre-heated material, compules were loaded with resin composite and placed inside a composite heating device (Calset, AdDent, Danbury, CT, USA) set to 68 °C for at least 5 min to stabilize the compule temperature inside the device. A Centrix syringe was used to deliver the resin composite inside the cavity in a single increment. Time was controlled so that each restoration had to start the light activation at 1 min after removing the compule from the heating device. This was done to standardize the amount of heat loss for every group, which has been estimated to be around 10 °C/min .
2.7
Degree of conversion
After being restored, teeth were sectioned in 1 mm slices with a low-speed diamond saw under water irrigation. Slices were analyzed with micro-Raman spectroscopy (Senterra, Bruker Optik GmbH, Ettlingen, Germany). An initial spectrum was collected for each unpolymerized resin composite to identify and compare the reaction peaks by a 100-mW diode laser with 785-nm wavelength. Five samples from each group were analyzed to calculate the degree of conversion. Only central slices from restorations were used to standardize for light exposure. One reading 50 μm from the top surface and one from the bottom surface of the restoration were recorded. The aromatic peak, observed at 1610 cm −1 , was used as the reference peak and the vinyl peak, observed at 1640 cm −1 , as the reaction peak. The degree of conversion was calculated using the ratio between the reaction and internal reference peak areas as the ratio of polymerized to unpolymerized resin composite. The DC was calculated according to the formula:
DC(%)=100∗1−H2/H1(polymerized)H2/H1(non-polymerized)
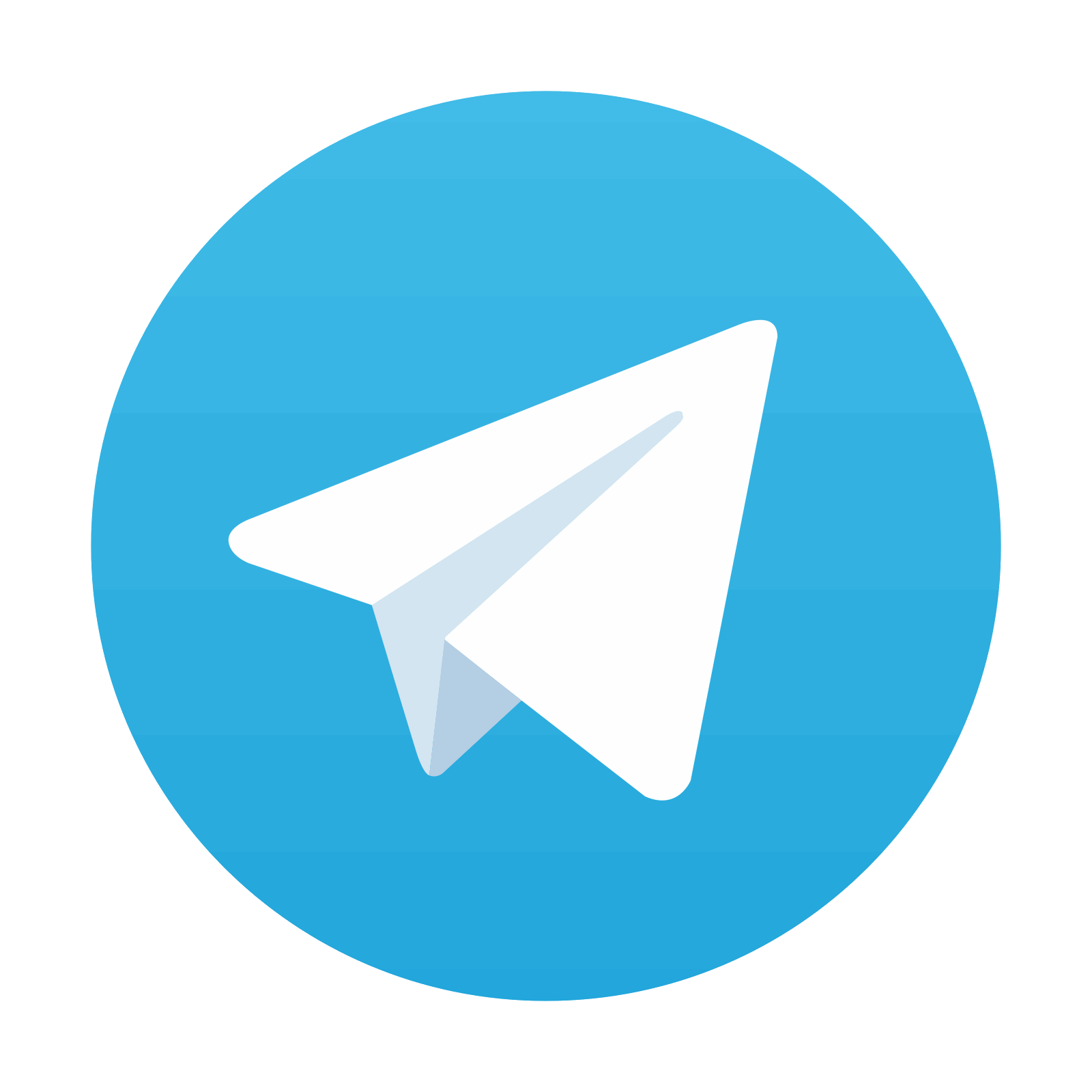
Stay updated, free dental videos. Join our Telegram channel

VIDEdental - Online dental courses
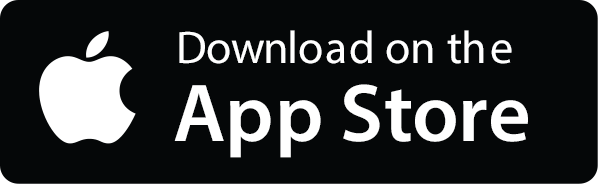
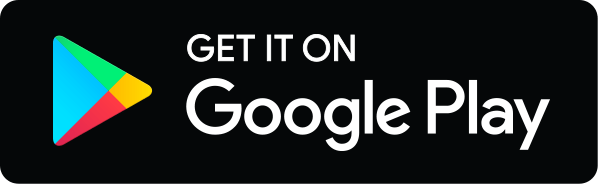
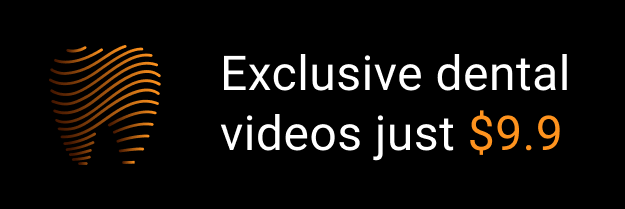