9
Clinical Applications of Digital Dental Technology in Implant Surgery : Computer‐Aided Implant Surgery
Hans-Peter Weber, Mariam Margvelashvili-Malament, and Andre Barbisan De Souza
9.1 Introduction
Technological advancements and engineering breakthroughs have transformed the protocols of medical sciences. Digital technologies, artificial intelligence, and tele‐medicine have become inseparable parts of various medical fields (Seyhan and Carini 2019). Dentistry is not an exception, and increased computerization can be seen in clinical dentistry and dental research (Chen et al. 2020). Perhaps one of the pioneer dental fields to have adopted widespread digital workflows is implant dentistry. In fact, computer‐aided implant surgery (CAIS) is becoming the standard in both academic and private dental environments.
Cornerstone for CAIS was the development of cone beam computed tomography (CBCT), which allowed three‐dimensional (3D) and accurate visualization of bony structures at reduced radiation dosage (Guerrero et al. 2006). The total radiation of CBCT devices is approximately 20% less than that of a helical computed tomography (CT) and corresponds to the exposure of a full‐mouth periapical series (Mah et al. 2003).
At the same time, the leaders in implant dentistry pointed out the importance of converting information from planned slices to the tangible structures of the patient. The article was pioneering the discussion of 3D implant planning and ways to transfer the planned data to the surgical area to achieve anatomically safe implant placement (Verstreken et al. 1996). As implant dentistry evolved, it became clearer that prosthetically driven implant placement is equally important for esthetic results and long‐term success of implant‐retained restorations (Cooper 2008). With that in mind, earlier protocols included using radiopaque guides, which provided information regarding the future restoration (e.g. incisal edge position, cervical margin) in relation to the existing alveolar bone. These imaging guides were then converted into surgical templates to direct implant positioning (conventional guides).
Computer‐aided implant surgery has evolved and become more popular since then and entails proven advantages, such as reduced time for surgery, improved postoperative healing, anatomically safe and prosthetically driven implant placement, and reduced number of appointments. To a certain extent, it could even compensate for limited surgical experience (Vercruyssen et al. 2015).
Technological advancements and their integration in implant dentistry allowed the development of various CAIS protocols. Based on the type of workflow or technology that is chosen, CAIS can be categorized into two major types: computer‐guided (static) surgery and computer‐navigated (dynamic) surgery (Hammerle et al. 2009).
This chapter will review current technologies and protocols for CAIS. It will mostly focus on static CAIS, which has been adopted by clinicians globally. It describes workflows for various clinical scenarios, current protocols, and clinical applications as well as limitations and future directions. It also briefly summarizes workflows for dynamic computer‐aided and robot‐assisted implant surgeries for comparison purposes.
9.2 Prosthetically Driven 3D Implant Positioning
Success of dental implants have largely been described based on the evidence of osseointegration and implant survival (Albrektsson et al. 1986). However, implant success has been redefined, and some newer evaluation parameters have been introduced. Soft tissue health status, natural appearance, esthetics, and patient satisfaction were among the new criteria. Perhaps, one of the most important assessments is to evaluate the implant–prosthetic complex as a whole (Papaspyridakos et al. 2012).
The evaluation criteria of anterior implants have also been updated. The goal is to achieve implant‐retained restorations that are unnoticeable and provide esthetics equal to or better than the contralateral and adjacent teeth (Cooper 2008, Testori et al. 2018). This was called the “fine‐tuning phase” in implant dentistry (Buser et al. 2017).
Recent studies have pointed out the association between prosthetic features like restoration emergence contour and emergence angle with peri‐implant disease (Katafuchi et al. 2018, Yi et al. 2020). Specifically, overcontoured, convex prosthetic profiles had a significantly higher risk for developing peri‐implantitis. Not only peri‐implant disease, but also a higher incidence of caries was linked with implants that have more than 4 mm implant‐to‐tooth horizontal distance as measured at the alveolar crest. It is conceivable that large gingival embrasures and overcontoured restorations caused a 17% incidence of caries on adjacent teeth (Smith et al. 2020).
Thus, to avoid aforementioned comorbidities and achieve an implant‐retained prostheses with predictable long‐term success and favorable esthetic outcomes, optimal, prosthetically driven positioning of an implant in mesio‐distal, apico‐coronal, and bucco‐lingual dimensions is crucial. For missing single teeth, multiple references are present that can guide an experienced surgeon to ideal implant positioning. However, with multiple missing adjacent teeth, where the landmarks from adjacent and opposing teeth are absent, prosthetically correct free‐hand implant placement is rather challenging. In these instances, CAIS can provide both anatomically safe and prosthetically driven implant placement. Planning of a future restoration can be achieved with the help of radiopaque templates, temporary restorations, and/or computer‐aided design (CAD) software. This allows clinicians to plan the best possible implant position from a restorative point of view while taking into consideration the anatomy and the volume of the alveolar bone.
9.3 Computer‐Aided Implant Planning
As mentioned above, CBCT provides accurate 3D bone volume information at lower and safe radiation dose levels. Bone volumetric data is displayed in cross‐sectional images in relation to prospective implant position. Also, implant‐planning software can display 3D surface models. Due to different tissue characteristics, the volumetric data has various radiation attenuations. This can enable the selective display of anatomical structures (e.g. teeth, bone), referred to as segmentation. Nevertheless, digital‐imaging‐and‐communication‐in‐medicine (DICOM) files do not display sufficient detail of tooth surfaces for the digital design of prostheses and/or planning osteotomy guides. For this reason, optical scan Standard Tessellation Language (STL) files obtained through an intraoral or extraoral scan are incorporated with a DICOM file to merge the information of bone volume with tooth and soft tissue surface patterns. The process of superimposition of different imaging datasets is called registration. Multiple references can be used to achieve accurate registration/alignment. The most common one is probably the tooth surface. In the absence of teeth or the presence of highly radio‐opaque restorations (silver amalgam fillings, metal restorations, porcelain‐fused‐to‐metal restorations), custom reference markers (fiducial markers) are used, either attached to non‐mobile soft tissue surfaces or to radiographic templates (Kernen et al. 2020).
Most of the available software options have built‐in artificial intelligence algorithms to align corresponding anatomical surface references. Some of the software requires marking of certain corresponding areas to facilitate (semi‐automatic) registration. Accurate superimposition of various datasets is crucial for reliable implant planning. For this reason, clinicians often correct automated alignment and account for any possible inaccuracies (Margvelashvili‐Malament et al. 2019).
Following alignment of datasets, the next step is to acquire information about the future restoration (prosthetic plan). Most of the implant‐planning software options have built‐in CAD elements that allow virtual prosthetic set‐ups. Older software versions required a double‐scan technique, where two separate model scans were obtained: one with the missing teeth and the other with a wax‐up to incorporate the position and design of the future prosthesis. Alternative workflows with double scans were also proposed, where two intraoral scans are obtained: one with prepared teeth and one with the provisional restoration in place. A clinical example of this workflow is discussed below. This workflow is best suited for full‐mouth reconstructions, which entail provisionalization as a prior phase to implant surgery (Margvelashvili‐Malament et al. 2019).
Whether a double scan, double intraoral scan or the CAD features are used, the goal remains the same: prosthetically driven implant‐planning and placement. Clinicians should be able to visualize implant positions in the 3D bony environment in relation to a future restoration, adjacent teeth, emergence profile, incisal edge, cervical area, etc. (Kernen et al. 2020, Margvelashvili‐Malament et al. 2019).
9.4 Computer‐Aided Implant Surgery
Based on the type of workflow or technology chosen, CAIS can be categorized as two major types, which are defined as follows (Hammerle et al. 2009):
- Computer‐guided (static) surgery. The use of a static surgical template that reproduces the virtual implant position directly from CT data and does not allow for intra‐operative modification of the implant position.
- Computer‐navigated (dynamic) surgery. The use of a surgical navigation system that reproduces the virtual implant position directly from CT data and allows for intra‐operative changes in implant position.
The current protocol for computer‐aided static surgery incorporates a CBCT scan produced DICOM dataset with optical scan STL files allowing 3D planning in both virtual and a 3D bony environment. Based on the plan, a guide is then produced that copies the locked plan of the implant position to the surgical site (Jung et al. 2009).
Conversely, dynamic navigation does not require a surgical guide and allows visualization of osteotomy and implant placement in real time on a monitor (Block and Emery 2016, Edwards et al. 1999, Gargallo‐Albiol et al. 2019). Initially, the DICOM data is integrated into the navigation software to plan a 3D implant position. The surgeon then places implant and guides through visualizing on the screen (Block and Emery 2016, Edwards et al. 1999, Gargallo‐Albiol et al. 2019). Apart from the human‐controlled dynamic guidance, a relatively new system is the robot‐assisted implant surgery, which incorporates haptic guidance with dynamic computer‐assisted implant surgery. It physically guides and controls the surgeon’s hand to replicate planned implant angulation and positioning. Robot‐guided implant placement has the capability to track patient motion and adjust the parameters in real time (real‐time tracking). A surgeon can visually confirm the implant position on the monitor.
9.5 Static Computer‐Aided Implant Surgery and Guides
Once implant planning is completed, the osteotomy guide that will transfer the plan to the surgical site is designed. There are various types of static guides. Some allow a guided osteotomy followed by free‐hand implant placement, others offer guided osteotomy and guided implant placement, described as fully guided surgery (D’Haese et al. 2017). Fully guided surgery may use several guides in sequence of increasing drill diameters or a single guide with adjustable drill handles and appropriate sleeve diameters (Hammerle et al. 2009). The classification of static guides is summarized in Figure 9.1.

Figure 9.1 Classification of computer‐aided implant surgery.
9.5.1 Surgical Template Fixation Methods
The surgical guides may also be different in terms of the support and can be classified as follows (D’Haese et al. 2017):
- Tooth supported: a guide is supported by the remaining teeth of partially edentulous patients.
- Mucosa supported: a guide is placed on the soft tissues in completely edentulous patients.
- Tooth and mucosa supported: a guide is placed on remaining teeth and soft tissues. Combined support is used when remaining teeth do not provide sufficient stability. Primary stress bearing areas like retromolar pads, maxillary tuberosities, and horizontal palate are mostly chosen to minimize tissue resilience.
- Mucosa supported with anchor (trans‐mucosal) pins: a guide is placed on the alveolar ridge mucosa. Additional support and template stabilization are provided through anchor pins inserted into the underlying bone.
- Bone supported: a mucoperiosteal flap is raised, and the guide is placed on the alveolar bone in patients requiring more extensive osteoplasties.
- Mini‐implant supported: a guide is placed on mini implants, which are placed in the alveolar bone crest prior to or during surgery.
9.5.2 Fabrication Methods
After a surgical guide is designed, the information is further processed for its fabrication. Static surgical guides can be manufactured either in‐house or outsourced and fabricated using computer‐aided design and computer‐aided manufacturing (CAD/CAM) technology via additive (i.e. printing) or subtractive (i.e. milling) techniques (Jabero and Sarment 2006).
9.6 CAD/CAM Fabrication of Surgical Guides
In this technique, the planning data are transferred to the production center, which fabricates the surgical guides according to the given information, either via stereolithography or additive (3D printing) techniques.
9.6.1 Stereolithographic Surgical Guides
The stereolithographic technology involves the reproduction of the surgical guide via a laser beam that selectively solidifies an ultraviolet‐sensitive liquid resin. This technique can be associated with higher cost and longer manufacturing time. Fabrication cost of centrally fabricated surgical guides is generally higher. Also, since the data is sent to the production center and then the guide is shipped to the dental office, it takes longer.
As an example of this technique, the protocol of the NobelGuide™ system (Nobel Biocare AB, Zurich, Switzerland) is reviewed (Figure 9.2).
Firstly, a CBCT scan of the patient with a radiographic guide inserted is taken. Then, a second scan of only the radiographic template is performed using the same CT settings. The data is then incorporated in the software where the two scans are merged (registered) using fiducial markers of the imaging appliance. Afterwards, implant planning can be started. Once the clinician is satisfied with the digital treatment plan, the data are sent to the Nobel Biocare® workstation (Nobel Biocare AB, Zurich, Switzerland) for the fabrication of a stereolithographic model. The surgical stereolithographic template includes metallic sleeves. During the surgery, the clinician uses a sequence of drill guides that match with the diameter of each surgical drill. Finally, the implant is placed via the same stereolithographic surgical template.
Stereolithographic materials are sensitive to light and display a lack of thermal stability. However, neither of these features affected the accuracy of the guides (D’Haese et al. 2012). Nevertheless, direct sunlight, autoclaving, or chemical sterilization is not recommended.
9.6.2 Additive Manufacturing (3D Printing) of Guides
The principle of 3D printing is to slice a digital object in cross sections that are printed in layers (additive technique). Those layers are made of liquid, powder, or any sheet material and can be fused together to create objects with any shapes, including surgical guides. The accuracy requirements for anatomical models lie within a range of a tenth of a millimeter (Stopp and Luth 2007).

Figure 9.2 Static computer‐aided implant placement in completely edentulous jaw using dual‐scan technique. (a) Patient CBCT; (b) radiographic template CBCT; (c) registration of two CBCTs; (d) implant planning; (e) planned surgical guide.
Treatment times and labor resource can be reduced when using 3D printing technology. The templates can be produced in a production center (centralized) or even in a local laboratory (decentralized). For the production in the local laboratory, a 3D printer capable of producing surgical guides is required. Of course, local fabrication requires special skills and experience in planning, designing, and manufacturing surgical guides. With proper knowledge, devices, and software, surgical guide manufacturing via 3D printing can be straightforward and fast.
9.6.3 Workflows for Static Computer‐Aided Implant Placement
Depending on the number of remaining teeth, the workflow for CAIS may vary. The workflows, types of guides, and predictability for partial or complete edentulism are characterized by significant differences. Therefore, they are discussed separately below.
9.6.4 Partially Edentulous Arches (Single and Multiple Missing Teeth)
Like in any dental intervention, a thorough examination and diagnosis of the patient is required for implementing CAIS. Regardless of the workflow, data registration of a tomographic image (DICOM file) with an STL file of an optical scan obtained directly using an intraoral scanner or indirectly by scanning a conventional impression or dental cast using a desktop scanner is needed.
Surgical guides for a single missing tooth and for most multiple missing teeth scenarios are tooth supported. However, a combination of teeth and mucosa or bone‐supported guides can be fabricated in some long‐span distal extension edentulous areas for providing additional support. Maxillary tuberosities or retromolar pads are the anatomical locations used for this purpose (Figure 9.3).
Clinical examples of implants placed in single tooth and extended edentulous spaces using static computer‐aided implant placement are presented in figures (Figures 9.3–9.9 and Videos 9.1 and 9.2).
The workflows for partial edentulism are summarized in Figure 9.10.
9.6.5 Completely Edentulous Arches
Computer‐aided implant placement for completely edentulous patients relies on the same basic principles of completing a comprehensive examination and obtaining 3D data of bone, soft tissue surfaces, and future prosthesis.
Data acquisition can be accomplished through conventional or digital methods or by a combination of the two. The first step in the digital workflow is to obtain optical data of edentulous jaws. It can be done by making conventional impressions or fabricating casts, which are scanned with a desktop scanner, or fully digitally, using an intraoral scanner. The next step is to visualize the future restoration. This can be achieved by duplicating existing conventional dentures or a lab‐based tooth set‐up. The duplication of a pre‐existing denture often requires an intraoral reline of the duplicate to ensure adequate fit. In the event of a lab‐based tooth set‐up, intraoral confirmation and appropriate adjustments are required based on classic denture prosthodontic principles. When the CBCT is taken with the resin‐based denture, it is characterized by similar density and radiation attenuation of the soft tissues. Therefore, it is hard to differentiate either of the latter during segmentation (Vercruyssen et al. 2015). For this reason, an imaging appliance is fabricated based on the denture replica or tooth set‐up using a radio‐opaque material. The CBCT is taken with the imaging appliance. Ideal implant positioning, taking into consideration remaining alveolar bone volume and future prosthesis, is then planned to produce the surgical guide. A clinical case is presented in Figures 9.11–9.14 and Video 9.2.

Figure 9.3 Clinical case demonstrating an unbounded edentulous space using a tooth‐mucosa supported surgical guide during planning: (a) and (b) lateral and occlusal views and during surgery; (c) and (d) lateral and occlusal views.

Figure 9.4 Patient with edentulous left central incisor extracted six weeks ago, planned for fully guided early implant placement (a) and (b) frontal and occlusal views.

Figure 9.5 Superimposition/registration as part of the process for static computer‐aided implant surgery: (a) three‐dimensional image of CBCT; (b) edentulous arch surface scan superimposed to CBCT three‐dimensional image; (c) dentate arch scan surface before extraction to be used as reference for implant planning.

Figure 9.6 Digital implant planning for fully guided implant placement of a 3.3×12 mm Straumann bone level tapered implant: (a) three‐dimensional image of digital implant planning of edentulous site for a screw‐retained restoration; (b) tooth‐supported surgical guide design; (c) cross‐sectional view of the CBCT demonstrating the relationship between implant planning, alveolar bone, soft tissue profile and future prosthetic restoration; (d) tangential view; (e) a series of axial view demonstrating the relationship between implant planning and the anatomical structures (in red) nasopalatine canal and adjacent root.

Figure 9.7 (a) Surgical guide in position after full‐thickness flap elevations; (b) implant placement fully guided; (c) and (d) facial deficiency “v‐shape” expected for Type 2 implant placement modality.

Figure 9.8 (a) Guided bone regeneration using autogenous bone with deproteinized bovine bone mineral and covered with double‐layer collagen membrane; (b) immediate post‐surgical photograph; (c) facial; (d) occlusal view of the surgical site 15 days after surgery.

Figure 9.9 (a) Facial view; (b) occlusal view of the surgical site three months after surgery; (c) facial view; (d) occlusal views of final screw‐retained implant‐supported zirconia restoration.

Figure 9.10 Workflow for static computer‐aided implant placement in partial edentulism cases.

Figure 9.11 Digital implant planning of fully edentulous patient using double‐scan technique: (a) three‐dimensional CBCT image superimposed with digital denture; (b) virtual implant planning for eight implants as part of a plan for three pieces segmented prosthesis; (c) planning of four horizontal anchor pins to stabilize the surgical guide; (d) surgical guide design mucosa‐supported with horizontal anchor pins.
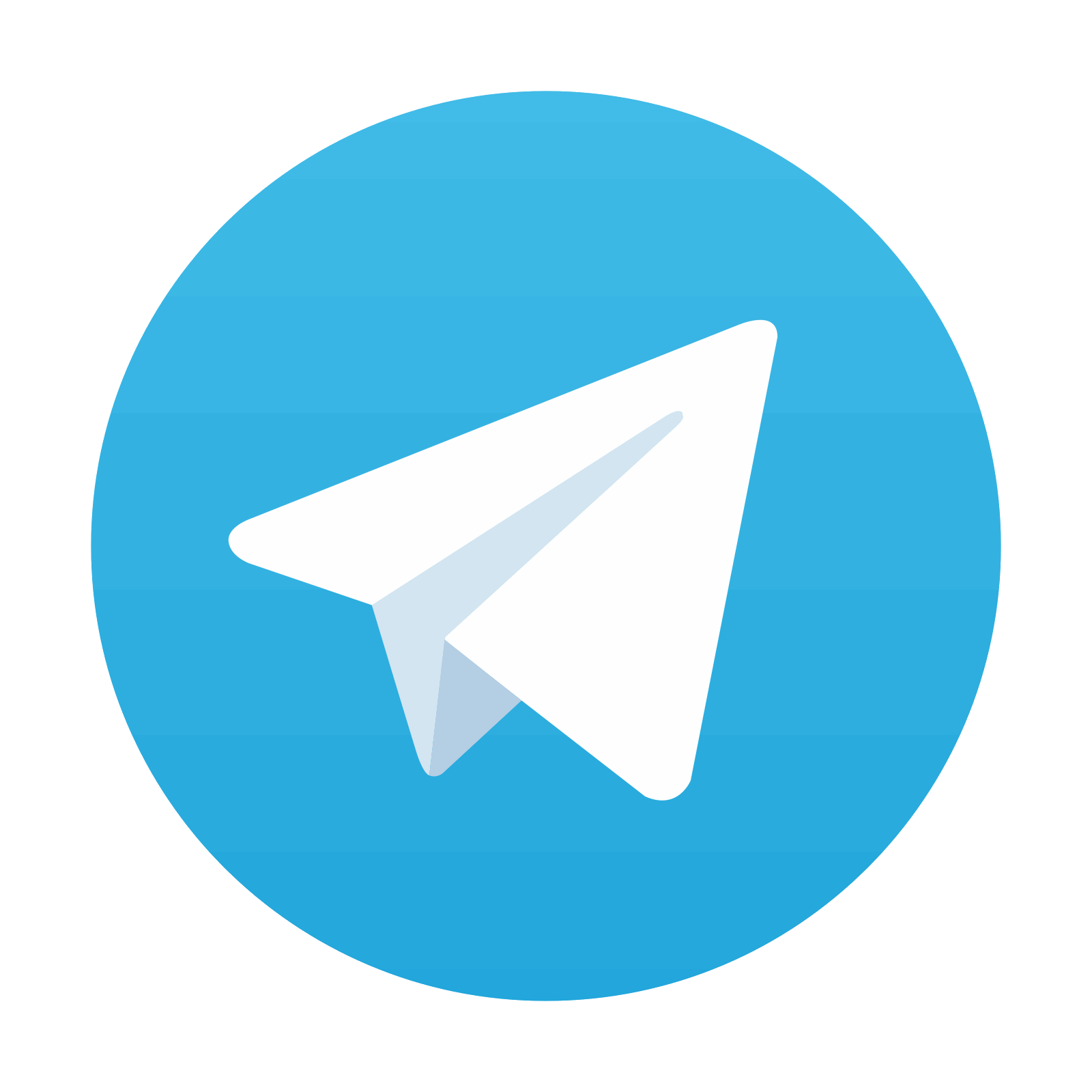
Stay updated, free dental videos. Join our Telegram channel

VIDEdental - Online dental courses
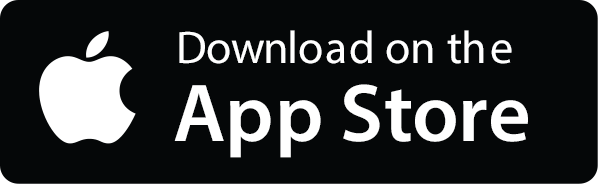
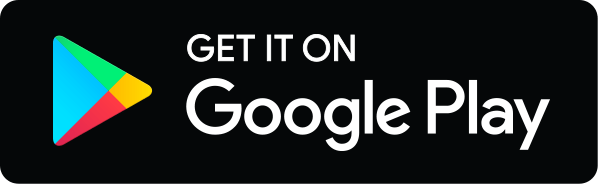