Fig 13.1 Bio-Oss is a natural, osteoconductive bone substitute that promotes bone growth in periodontal and maxillofacial osseous defects. It consists of the mineral portion of bovine bone. (A and B) Bio-Oss provides the body with a matrix for bone cell migration and is integrated into the natural physiologic remodelling process.
(Courtesy: Geistlich Biomaterials)
Bio-Oss: morphology like the human bone
Geistlich Bio-Oss® is a natural bone substitute material obtained from the mineral portion of bovine bone. The reason for the good bone regeneration seen with Geistlich Bio-Oss® is its close resemblance to human bone. Bio-Oss was developed as an ideal bone substitute that would replicate the structure of autogenous bone. Each step in the development of Bio-Oss was conducted with this goal in mind, resulting in a matrix that is very similar in physical and chemical composition to human bone (< ?xml:namespace prefix = "mbp" />
Process of natural bone regeneration with Bio-Oss
When Bio-Oss is grafted at the osseous defect, it provides a bioinert and bioactive scaffold, into which new blood vessels grow from the peripheral host bone and osteoblasts migrate into the graft and form a new bone at the graft site. The graft gets slowly replaced by new bone via resorption and substitution during remodelling phase of bone (
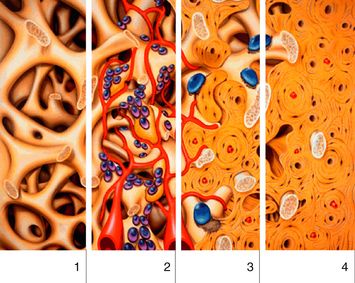
Fig 13.3 Clot stabilization facilitated by Bio-Oss interconnecting macro- and micropores (1). Revascularization (red), migration of osteoblasts (purple) and in-growth of woven bone (yellow) is enhanced by Bio-Oss scaffolding (2). Lamellar bone (dark yellow) and Bio-Oss (light yellow) are successfully integrated after approximately 6 months. Bio-Oss is included in the natural physiologic remodelling process (osteoclasts – blue) (3). Finally remodelled new bone formation at the site grafted with the Bio-Oss (4).
Advantages of Bio-Oss
1. Superior handling characteristics made possible by the large hydrophilic inner surface area similar to human bone.
2. Promotes revascularization and clot stabilization, due to its interconnecting macropores and micropores.
3. Facilitates bone formation by providing an exceptional osteoconductive scaffolding, which results from the retention of the natural porous architecture and trabeculation of human cancellous bone.
4. Effective space maintenance, and when integrated, provides mechanical strength and stiffness due to retention of the natural mineral content.
5. Optimal integration with the patient’s own bone aided by a chemical composition analogous to human bone with fewer hydroxyl and more carbonate groups than most synthetic materials.
6. Bio-Oss is integrated during the natural remodelling process of the human bone and slowly resorbed due to small crystallite size, which is comparable to human bone.
7. Effective bone regeneration that has been clinically and scientifically proven for more than 15 years.
8. Bio-Oss prevents newly formed bone from rapid resorption and leads to a long-term preservation of bone volume.
Disadvantages of Bio-Oss
The only disadvantage of Bio-Oss is its slow substitution rate, as the material may appear as pebbles or gel upon re-entry to the grafted site.
Synthetic bone graft/alloplastic graft
The bone grafts which are created from the synthetic source (ceramics) such as calcium phosphates (e.g. hydroxyapatite and tricalcium phosphate), bioglass, and calcium sulphate are called alloplastic or synthetic bone grafts.
Alloplastic grafts are often made of hydroxyapatite or other naturally occurring and biocompatible substances with mechanical properties similar to those of bone. Hydroxyapatite is a synthetic bone graft, which is the most commonly used among other synthetic grafts due to its osteoconduction property, hardness, and acceptability by bone. Hydroxyapatite (HA), if used in combination with tricalcium phosphate (TCP), gives both osteoconduction and resorbability, and thus is being widely used in bone regeneration procedures (OSTEON graft).
Graft quality for successful osteoconduction
1. The graft must provide a bioinert or bioactive scaffold at the ectopic site for new bone formation with the process of osteoconduction.
2. The material should be porous and hydrophilic to favour tissue growth and bony deposition.
3. It should be slowly replaced by new bone via resorption and substitution during the remodelling phase of bone.
4. The scaffold should have a microtopography similar to bone.
Mechanism of bone regeneration by various types of grafts
As described earlier in this chapter, bone formation at the grafted site occurs by three mechanisms – osteoconduction, osteoinduction, and osteogenesis. Various types of grafts play different roles in bone formation depending on their source. The alloplastic or synthetic grafts and xenografts show only osteoconductive properties and only provide a bioinert and bioactive scaffold onto which the blood vessels and osteoblasts migrate from the host bone, to form the new bone. The allograft shows the osteoconductive and also a variable degree of osteoinductive properties. Thus, besides providing a scaffold at the ectopic site, it may also induce the host bone into the procedure of bone formation at the grafted site. Besides showing more enhanced properties of osteoinductivity and osteoconductivity, the demineralization of allografts results in the graft becoming a little osteogenic. The autograft therefore forms bone by all three mechanisms – osteoconduction, osteoinduction, and osteogenesis. Besides providing a scaffold and inducing the host bone into bone formation like the allografts, the autograft also contains the vital cells to begin new bone formation at the graft site before bone formation occurs by the other two mechanisms (
Growth factors
Growth factor enhanced grafts are produced using recombinant DNA technology. They consist of either human growth factors or morphogens (BMPs in conjunction with a carrier medium, such as collagen). The implant surgeon can also extract the growth factors such as platelet-rich plasma (PRP), Plasma rich in growth factors (PRGF), or platelet rich-fibrin (PRF) from the patient’s venous blood just before surgery and mix in the graft to enhance its bone regeneration properties (
Composite graft
A composite graft consists of a mixture of autogenous cancellous marrow and a slow-substitution rate bone graft substitute, in a specified ratio.
Two-phase theory of osteogenesis
The new bone formation at the grafted site occurs in two phases when an osteogenic graft (autograft) is used.
Phase I
Volume yield from graft depends upon the concentration of viable cells transplanted from the donor through the process of osteogenesis. The autogenous cancellous marrow grafts are known to be the ‘Gold Standard’ for phase I osteogenesis. They are the most useful and predictable grafts because they transfer the viable osteoblast and osteoprogenitor cells to support phase I bone graft healing.
Phase II
Osteoinduction and osteoconduction of osteocompetent cells from the recipient site responsible for graft incorporation, remodelling and replacement according to local stress and strain factors mediated by site specific signalling.
The two-phase theory can be simplified as the autogenous graft transfers the viable osteoblasts and osteoprogenitor cells, which initiate new bone formation at the graft site (phase I osteogenesis), and later on the osteocompetent cells from the recipient site, which get incorporated into the graft, cause remodelling and replacement of the graft with new bone by the process of osteoinduction and osteoconduction (phase II osteogenesis).
Physical forms of grafts
Different types of grafts can be available or harvested in various physical forms and clinically used according to the requirements of the particular case of bone augmentation. The autogenous bone can be harvested and used in various forms like bone blocks (only cortical or corticocancellous), strips, and particulate graft (only cortical chips, corticocancellous, or only cancellous) (
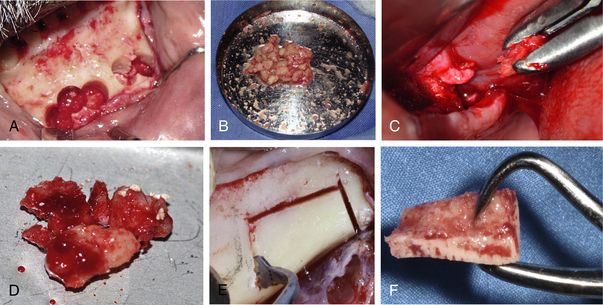
Fig 13.5 Figures showing autogenous bone in various physical forms – autogenous cortical bone harvested from the mandibular symphysis using trephines (A) and (B) crushed to be used as particulate cortical chips. (C and D) The cancellous bone harvested from the maxillary tuberosity and used as a particulate autograft. (E and F) The cortical block graft harvested from the mandibular buccal shelf.
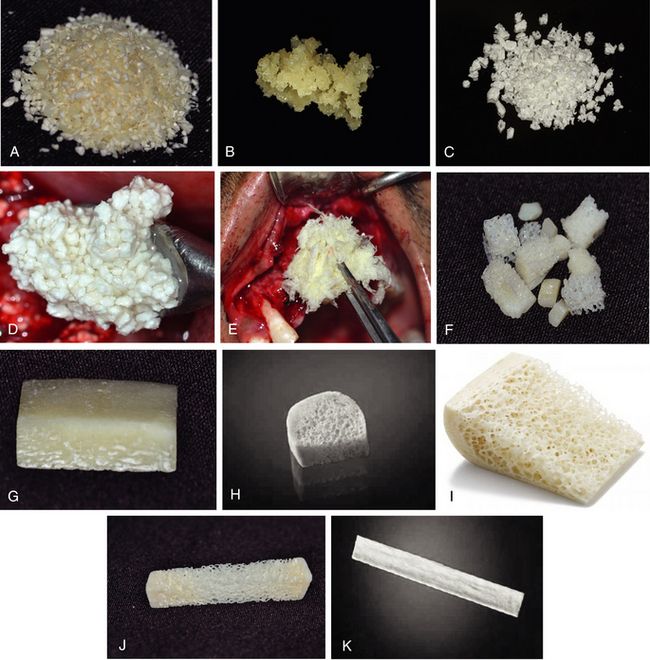
Fig 13.6 (A) Figures showing bone substitutes in various physical forms – particulate form of mineralized and (B) demineralized bone allograft. (C) Particulated xenograft, (D) particulated form of HA + β-Tcp mixture, (E) demineralized allograft in putty form, (F) allograft in the form of corticocancellous chips, (G) cortical block allograft, (H) cancellous block allograft, (I) corticocancellous block allograft (Courtesy: Zimmer Dental), (J) cancellous strip allograft, (K) cortical strip allograft.
Keys for successful bone grafting
Surgical asepsis/absence of infection
Infected sites should never be selected for bone grafting until all the infection has been removed and the site has healed with no sign of any active infection like pain, swelling, and purulent discharge. Bacterial infection reduces the pH level at the infected site, which causes solution-mediated rapid resorption of grafted material. There should be no clinical or radiographic findings of any active infection at the site chosen to graft (recipient site) or harvest (donor site) bone graft material. The grafted site should also be prevented from receiving any infection after the procedure because of poor oral hygiene, suture line opening, or membrane or graft exposure to the oral environment, etc. Antibiotics can be added to alloplastic material and autograft. Although tetracycline is often used in periodontal bone grafting to improve collagen formation, it chelates calcium and arrests the bone formation process. Instead, parenteral penicillin, cephalosporin, or clindamycin should be mixed into the graft material, because these antibiotics do not affect the process of bone regeneration (
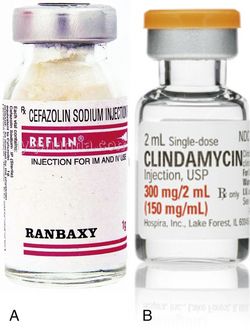
Fig 13.7 (A) A parenteral form of cefazolin sodium (Reflin) or (B) clindamycin can be mixed with graft material at the time of grafting procedure. These antibiotics can also be added to the graft material when the site has been contaminated and has become infected in the early phase of the healing process. This provides increased level of antibiotic concentration at the site, even though vascularization is incomplete.
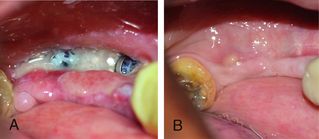
Fig 13.8 Suture line opening can result in loss of graft, soft tissue ingression, and infection to the graft. To prevent the exposed graft getting contaminated/infected, the site can be irrigated with parenteral antibiotics (e.g. cefazolin or clindamycin) two to three times a day till the site gets healed with secondary intension (A and B). This provides increased level of antibiotic concentration at the site, even though vascularization is incomplete.
Space maintenance
Space maintenance at the bone graft site is paramount to the bone formation process. The space of the graft site refers to the anatomical site and contour of the desired augmentation, and maintenance refers to the fact that the space must exist long enough for bone to fill the desired region. The barrier membrane which is used to cover the graft often collapses in the grafted space, which leads to the loss/resorption of a partial volume of the graft and results in under contoured new bone formation. The barrier membrane can be prevented from collapsing by using membrane fixation/stabilization screws (‘membrane tacks’) but better contour can be achieved by using a fixation screw which is elevated above the host bone level to the height/width of the desired bone volume to support the barrier membrane from underneath; this screw is called ‘tent screw.’ Tent screws, titanium mesh or titanium-reinforced membrane and graft materials beneath the membrane have been advocated to maintain the desired space during the bone grafting process. The osseous defect, which itself can provide adequate space for new bone regeneration is called ‘favourable osseous defect.’ This kind of defect does not need any tent screw but the walls of the osseous defect itself keep tenting the barrier membrane to maintain the space for new bone regeneration (
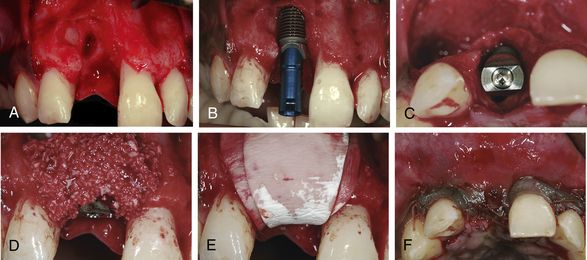
Fig 13.9 The favourable osseous defect, where implant placement has been possible within the osseous envelope of the defect. (A–F) The walls of the defect have provided adequate space maintenance for the graft by tenting up the membrane from underneath
(Courtesy: Dr Peter Randelzhofer, DDS, private practice, Germany).
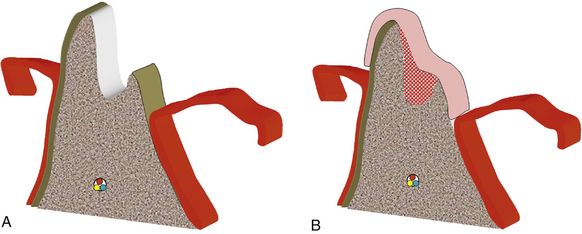
Fig 13.10 An unfavourable bone defect is grafted using particulate bone graft covered with barrier membrane. (A and B) The barrier membrane collapsed in the defect, resulting in compromised space for new bone formation of desired volume and contour.
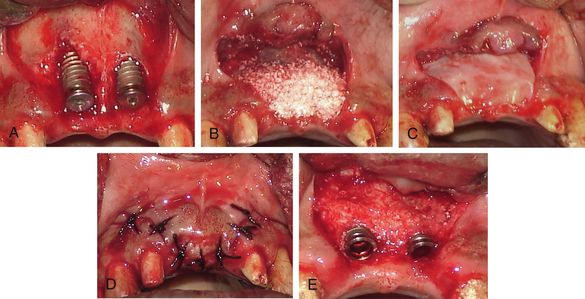
Fig 13.11 Unfavourable osseous defects, where implants could not be placed within the bony envelope. (A–E) Even the defects have been adequately grafted and covered with barrier membrane; the uncovering of the implants shows inadequate amount of bone formation because of inadequate space maintenance and loss of graft.
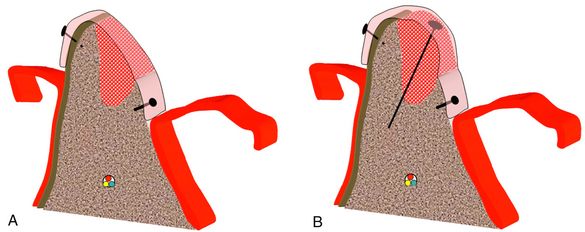
Fig 13.12 (A) The barrier membrane, which is stabilized using fixation screws (membrane tacks) to prevent the membrane from collapsing. (B) Using a long tent screw, which supports the membrane from underneath, results in better space maintenance for the graft and new bone formation of the desired volume and contour.
CASE REPORT
Lateral and vertical bone augmentation in the posterior mandible using tent screws (Courtesy: Dr Jun Shimada, Japan) (
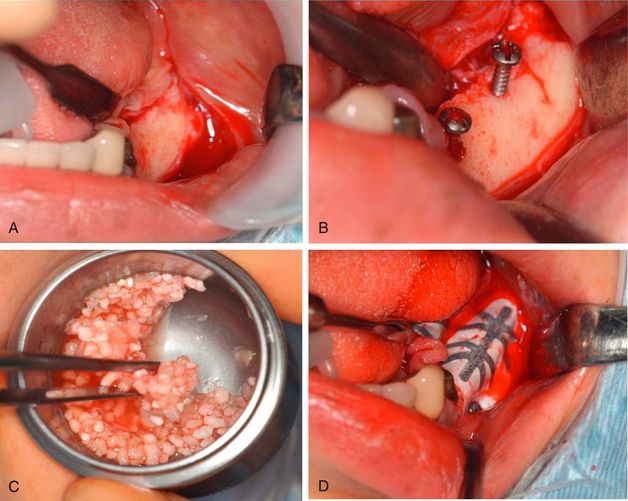
Fig 13.13 (A) The edentulous posterior mandibular ridge shows vertical as well as horizontal bone deficiency. (B) Two tent screws are fixed to the host site keeping them well emerged out of the bony surface. (C) A particulated allograft mixed with platelet-rich plasma (PRP) is used to augment the deficient area to the desired dimensions and covered with titanium-reinforced nonresorbable TXT membrane, which is supported by the (D) elevated tent screws from underneath.
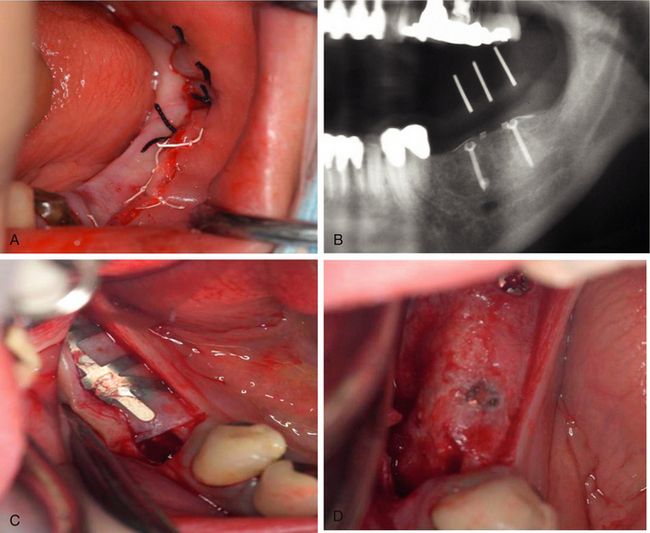
Fig 13.14 (A) The periosteum is released and sutured to achieve a watertight primary closure. (B) Postgrafting radiograph. (C) The site is uncovered after 4 months of healing period and the TXT membrane is removed. (D) The grafted site shows a remarkable amount of new bone regeneration.
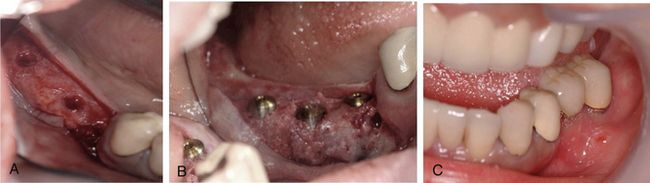
Fig 13.15 (A) The tent screws are removed and (B) implants of adequate sizes are inserted. (C) Clinical view after implants have been restored in function.
Soft tissue closure
The primary closure of the soft tissue is mandatory for the success of the grafting procedure, as it prevents the loss of graft from the site and prevents infection. However, if primary closure is not achieved (e.g. socket grafting), a nonresorbable cytoplast TXT membrane can be used to cover the grafted site to prevent any microbial invasion into the graft or loss of graft material. The primary soft tissue closure ensures healing by primary intension and requires minimal soft tissue collagen formation and soft tissue remodelling. It also minimizes postoperative discomfort to the patient. Often, it becomes difficult to achieve a primary closure over the grafted site because the volume underneath the flap increases after bone grafting. In such cases, primary closure can be achieved by releasing the periosteum of the flap so that it can be advanced to achieve the primary closure. There are two techniques for releasing the flap to achieve primary closure.
Submucosal space technique
This technique, developed by Misch in the early 1980s, is an effective method to expand tissue over larger grafts (greater than 15 × 10 mm in height and width) (
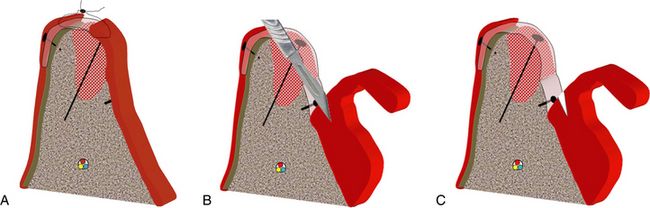
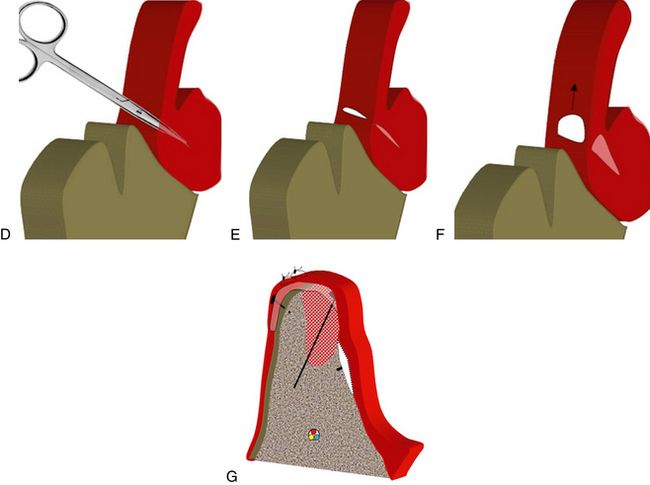
Fig 13.16 (A) Grafting of any bone defect increases the volume of the underlying hard tissue at the site, which may result in difficulty in achieving primary closure of the flap. The tension in sutures may result in suture line opening, which may cause the loss of graft and invasion of soft tissue into the defect area. A scalpel is inserted 1–2 mm deep through the periosteum underneath the facial flap and beyond the mucogingival junction. (B and C) Further, a horizontal incision is given parallel to the crestal incision. (D) Then, a soft tissue scissor is pushed into the facial flap for approximately 10 mm with the blades closed, parallel to the surface mucosa. The thickness of the facial flap is approximately 3–5 mm. The tissue scissors are opened, once at the proper depth. (E) This blunt dissection does not sever any blood vessels or nerves to the facial flap but does create a submucosal space or tunnel. (F and G) Once the submucosal space or tunnel has been created over and beyond the vertical release incisions, the facial flap can be advanced over the graft for tension free primary closure of the flap.
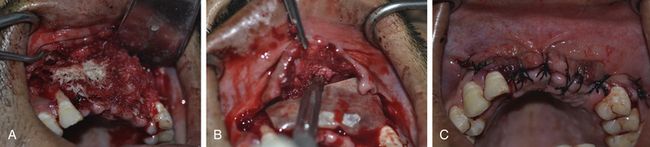
Fig 13.17 (A) Bone grafting has increased the volume of the hard tissue under the flap, which results in difficulty in achieving primary closure of the flap without tension in the sutures. (B) Thus a releasing incision is given through the periosteum of the facial flap for the easy coronal advancement of the flap and (C) to achieve primary closure without any tension in the flap.
Advantage: A large degree of flap advancement can be achieved for a tension-free flap closure covering the large grafted sites.
Disadvantage: As the incision is given into the periosteum, the blood supply to the grafted region and overlying soft tissue is affected, which results in longer time taken for soft tissue healing.
Curvilinear-bevelled incision technique
This is another method of flap advancement. The cut back incisions are made at the vestibular ends of the vertical incisions and the flap is coronally advanced and sutured first at the crestal part, followed by suturing of the vertical incisions at the vestibular half by mobilizing mobile vestibular soft tissue together. Only a limited degree of flap advancement is possible with this technique when compared to the submucosal space technique. The advantage of this technique is that it does not hinder the blood supply to the grafted region and overlying soft tissue because no horizontal incisions are made through the periosteum (
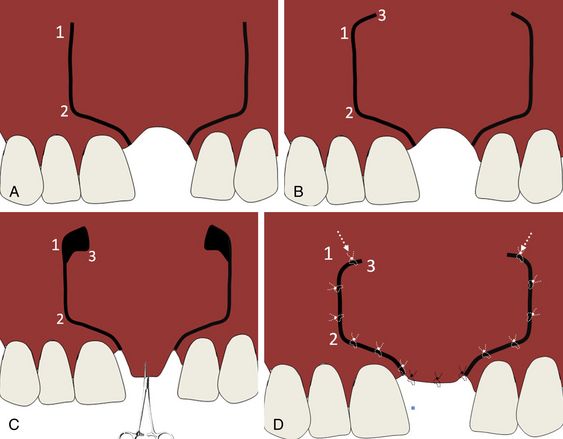
Fig 13.18 (A) Curvilinear-bevelled incision is given, and (B) extended with two cut back incisions at the vestibular ends. (C) The flap is advanced coronally and (D) sutured first at the crystal part with tension-free primary closure followed by suturing of nonkeratinized mobile vestibular tissue area. This technique can be followed for the smaller grafted regions as the coronal advancement of the flap with this technique is less than that obtained with the submucosal space technique but it has the advantage of not hindering the blood supply of the flap as the underlying periosteum remains intact to the flap.
Graft immobilization/fixation
Graft stabilization is a must to achieve a predictable bone augmentation. If the particulate graft material or the bone block graft is not stable at the host site, it cannot develop the blood supply from the host bone for new bone regeneration. This can further result in the graft becoming encapsulated in fibrous tissue and often sequestrated. For barrier membrane or particulate graft to work effectively, no load should be placed on the soft tissue over the graft, because it may cause movement of the graft. If barrier membrane is used, it should be immobilized to the graft site by using bone tacks. The block bone graft should firmly be immobilized to the host bone using multiple fixation screws to avoid any micromovement during bone remodelling (
Prevention of soft tissue ingression/barrier membranes
The epithelium and connecting tissue grows much faster than the new bone formation into a grafted area; hence, a barrier membrane is required to cover the bone graft to prevent the infiltration of the soft tissue into the graft site. A thick cortical plate of the block graft may act as a barrier membrane and often, the membrane is not required in the block grafting cases where a cortical block graft is used. If the periosteum of the flap over the grafted site is intact it can also act as natural barrier membrane and prevent epithelium and connective tissue infiltration into the graft.
Types of barrier membranes
There are various kinds of barrier membranes which are used in bone grafting and implant procedures. These membranes can either be the resorbable type (collagen membranes and pericardial membranes), which need to be completely covered by the soft tissue flap and are resorbed with time, or they are the nonresorbable type polytetrafluoroethylene (PTFE) membranes, which can be left exposed to the oral environment and need to be removed after the underlying graft has been consolidated (
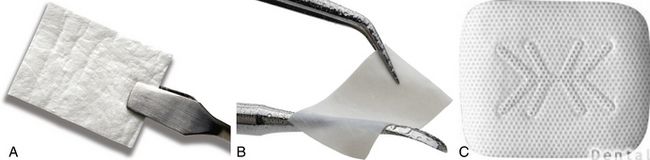
Fig 13.20 (A) Resorbable collagen membrane, (B) resorbable pericardium membrane from Zimmer Dental, and (C) nonresorbable PTFE Cytoplast TXT membrane.
Resorbable collagen membrane
These membranes are used to cover the graft where the primary closure of the flap is possible. These membranes keep preventing soft tissue ingression into the graft during its resorption and new bone formation. These membranes slowly get resorbed in 6–10 months and do not have to be removed (
Resorbable pericardium membrane
It is a biological three-layered membrane, which encases and protects the heart and can be used for guided tissue regeneration. The advantages of these membranes are flexible and adaptable, remodel/are resorbable, tough and resilient, suturable, and space creating.
Nonresorbable high-density PTFE barrier membrane
These are the nonresorbable type of membranes and so need to be removed after graft maturation. These membranes are very useful where soft tissue closure cannot be achieved because being nonresorbable these membranes can be left exposed to the oral environment (
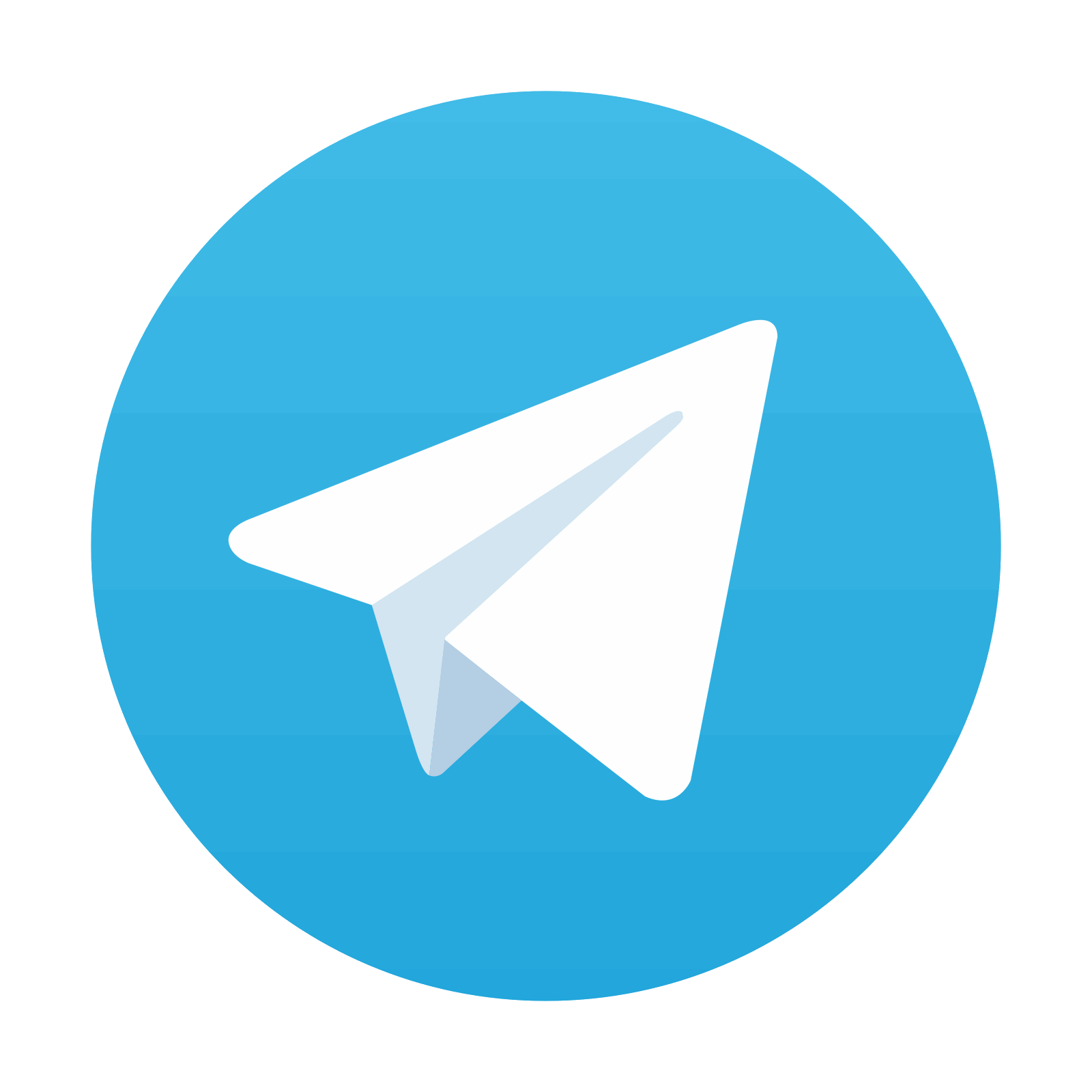
Stay updated, free dental videos. Join our Telegram channel

VIDEdental - Online dental courses
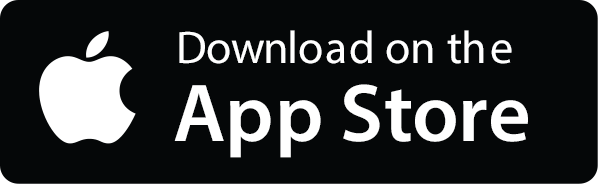
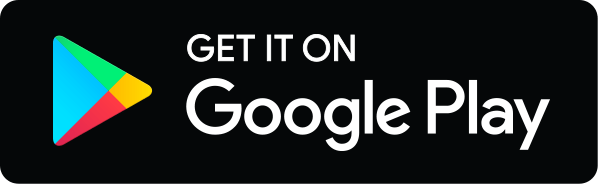