Introduction
Rapid prototyping is a fast-developing technique that might play a significant role in the eventual replacement of plaster dental models. The aim of this study was to investigate the accuracy and reproducibility of physical dental models reconstructed from digital data by several rapid prototyping techniques.
Methods
Twelve mandibular and maxillary conventional plaster models from randomly chosen subjects were selected and served as the gold standard. The plaster models were scanned to form high-resolution 3-dimensional surface models in .stl files. These files were converted into physical models using 3 rapid prototyping techniques: digital light processing, jetted photopolymer, and 3-dimensional printing. Linear measurements on the plaster models were compared with linear measurements on the rapid prototyping models. One observer measured the height and width of the clinical crowns of all teeth (first molar to first molar) on all models (plaster and replicas) using a digital caliper. All models were measured 5 times with a 2-week interval between measurements.
Results
The intraobserver agreement was high (intraclass correlation coefficient >0.94). The mean systematic differences for the measurements of the height of the clinical crowns were −0.02 mm for the jetted photopolymer models, 0.04 mm for the digital light processing models, and 0.25 mm for the 3-dimensional printing models. For the width of the teeth, the mean systematic differences were −0.08 mm for the jetted photopolymer models, −0.05 mm for the digital light processing models, and −0.05 mm for the 3-dimensional printing models.
Conclusions
Dental models reconstructed by the tested rapid prototyping techniques are considered clinically acceptable in terms of accuracy and reproducibility and might be appropriate for selected applications in orthodontics.
A significant contribution to the current efficiency in orthodontic practices is the digitization of dental models. Such models can be produced directly and indirectly, the former from a direct scan in the mouth and the latter from a scan of an impression. The digital data collected by the scan are then translated into a 3-dimensional (3D) model that can be viewed on a 2-dimensional computer screen. The advantage of this method compared with the conventional method (plaster models) is substantial; it has benefits in efficiency, ease of use, longevity (no risk of physical damage), and storage space (a hard disk instead of physical space).
However, working without plaster models is not yet customary. Although using digital models for diagnostic purposes is in general positively perceived by orthodontists, the tendency is still to supplement digital models with plaster models, especially when dealing with more complex problems. Practitioners prefer physical models over digital models because the former are more tangible and therefore more practical in direct communications with colleagues. Furthermore, physical models are still needed for appliance manufacturing. These models—conventionally plaster models produced from an alginate impression—can now also be reconstructed from the 3D data of the digital models by a manufacturing process called rapid prototyping ( Fig 1 ). This process of model reconstruction by adding layers of material is computer based and reconstructs physical models from 3D digital data through a variety of techniques.
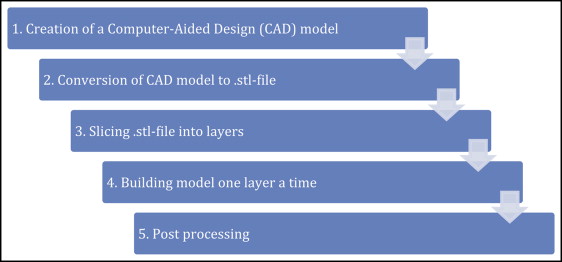
The applications of rapid prototyping in orthodontic practices are increasing. However, before rapid prototyping can be implemented, its accuracy and reproducibility must be evaluated. So far, only 1 study has compared 2 plaster models with their replicas created by stereolithography. The authors concluded that the mean difference in the z-plane (vertical direction) was highly significant for the replica, probably because of the thick layers of clear resin (0.15 mm) from which it was built. In addition, the study recommended investigation of other rapid prototyping techniques such as digital light processing and poly-jet modeling, which have thinner layers. Another study has recently compared stereolithographic replica models, although this time with the real dentition on several skulls and the digital models made from its scans. These stereolithographic models also showed statistically significant differences, but they were regarded as clinically insignificant.
Contrary to dental models reconstructed by rapid prototyping, digital models have been much studied for accuracy and reproducibility. Digital models are slightly less accurate than their original plaster models yet clinically acceptable. A recent systematic review concluded that digital models obtained from different techniques offer a high degree of validity in linear measurements compared with those made on plaster models directly and are generally clinically acceptable. Therefore, digital models are recommended as an alternative to conventional plaster models.
This study focuses on several rapid prototyping techniques that have not been evaluated so far. The purpose was to assess the accuracy and reproducibility of physical models reproduced by 3 different techniques ( Table I ) compared with plaster models for alternative and regular use in orthodontic practices.
Technique | Principle | Advantages | Disadvantages |
---|---|---|---|
Digital light processing, liquid based | Similar to stereolithography, uses visible light-sensitive resins instead of ultraviolet laser for curing each layer | Faster than stereolithography High accuracy |
Limited materials |
Jetted photopolymer, liquid based | Uses an array of ink-jet print heads to deposit tiny drops of building material and supporting material to form each layer of a part | Smooth surface finish High accuracy |
Feature detail and material properties less than stereolithography |
3D printing, powder based | Uses an ink-jet printing head that deposits a liquid adhesive that binds the material of each layer | Low costs Fast Easy to use |
Limited materials Less accuracy, surface finish, and part strength |
Material and methods
A total of 12 high-quality mandibular and maxillary pretreatment plaster models of 6 patients were randomly selected from a cohort of patients from the Department of Orthodontics, University Medical Center, University of Groningen, The Netherlands, and considered as the gold standard. Criteria to standardize the materials were (1) a complete permanent dentition erupted from first molar to first molar (no extractions), (2) normal crown morphology, (3) no carious lesions or interproximal wear, and (4) good-quality dental casts (no broken parts or air bubbles). The plaster models represented a variety of dental malocclusions.
All plaster models were scanned using a dual sensor laser scanner (RPS 450; Laser Design, Minneapolis, Minn). The point clouds produced were cleaned and triangulated to a polygon model with software (Geomagic, New York, NY). The resolution was kept high by minimal noise reduction and minimal removal of point clouds. The high-resolution 3D surface models were turned into .stl files and converted into physical models ( Fig 2 ) using 3 rapid prototyping techniques: digital light processing (Envisiontec, Gladbeck, Germany), jetted photopolymer (Objet Geometries, Rehovot, Israel), and 3D printing (Z-Corp, Rock Hill, SC).
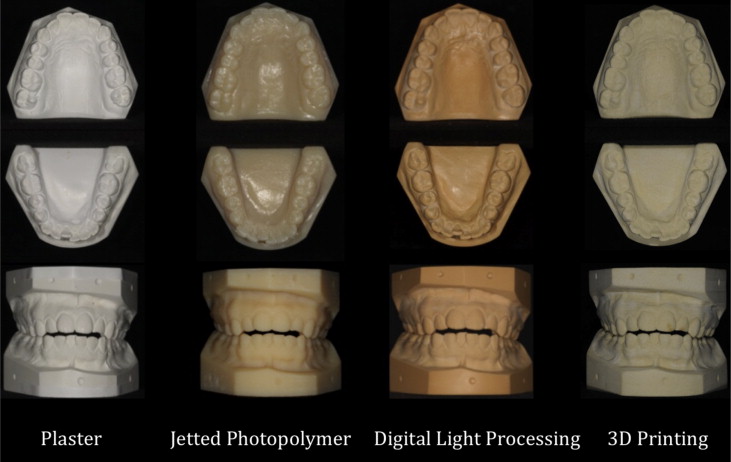
One observer (A.H.) made the linear measurements on all models (plaster and replica) using a calibrated electronic digital caliper (model H-59112; Fino, Bad Bocklet, Germany) with an accuracy of 0.01 mm. These measurements were done 5 times with a 2-week interval between measurements. The linear measurements were (1) mesiodistal measurements of all elements (first molar to first molar), taken at their greatest width, perpendicular to the occlusal plane ; and (2) clinical crown height measurements of all elements (first molar to first molar), measured as the distance from the most apical concavity of the gingival margin to the incisal edge or the occlusal surface of an element.
Raw data were obtained by multiplying the number of maxillary and mandibular plaster models (12), the number of elements (12), the measured directions per element (2), and the number of rapid prototyping techniques used plus the gold standard (3 + 1); this resulted in 1152 measurements. All measurements were done 5 times, resulting in a maximum of 5760 scores.
Statistical analysis
To determine the agreement between the plaster models and the replicas, a Bland-Altman analysis was used. The Bolton analysis was performed on the model pairs to compare the differences in tooth-size discrepancies between the plaster models and the replicas. The intraobserver reliability of the repeated measurements was determined by the intraclass correlation coefficient (ICC) based on absolute agreement. For the analyses, SPSS software (version 18; SPSS, Chicago, Ill) and MedCalc software (version 12.2.1; Medcalc, Mariakerke, Belgium) were used.
Results
The intraobserver correlation coefficient was high ( Table II ) for all measurements on all the dental models. The ICC was at least 0.935 (95% confidence interval [CI], 0.891-0.978), indicating that all models have high reproducibility.
ICC (95% CI) | |||||||||||||
---|---|---|---|---|---|---|---|---|---|---|---|---|---|
Measurement | Model | 1 | 2 | 3 | 4 | 5 | 6 | 7 | 8 | 9 | 10 | 11 | 12 |
MD | Plaster | 0.999 (0.998-1.000) | 0.995 (0.990-0.999) | 0.999 (0.999-1.000) | 0.998 (0.996-0.999) | 0.998 (0.995-0.999) | 0.999 (0.997-1.000) | 0.996 (0.992-0.999) | 0.996 (0.991-0.999) | 0.994 (0.986-0.998) | 0.999 (0.997-1.000) | 0.998 (0.995-0.999) | 0.998 (0.995-0.999) |
DLP | 0.997 (0.993-0.999) | 0.992 (0.982-0.982) | 0.997 (0.994-0.999) | 0.993 (0.984-0.998) | 0.995 (0.989-0.998) | 0.994 (0.987-0.998) | 0.982 (0.960-0.994) | 0.987 (0.971-0.996) | 0.944 (0.879-0.981) | 0.992 (0.982-0.997) | 0.989 (0.976-0.996) | 0.985 (0.966-0.995) | |
JP | 0.996 (0.992-0.999) | 0.984 (0.965-0.995) | 0.993 (0.985-0.998) | 0.984 (0.964-0.995) | 0.983 (0.962-0.994) | 0.992 (0.982-0.997) | 0.984 (0.964-0.995) | 0.958 (0.908-0.986) | 0.968 (0.929-0.989) | 0.978 (0.952-0.993) | 0.979 (0.952-0.993) | 0.975 (0.944-0.992) | |
3DP | 0.998 (0.996-0.999) | 0.994 (0.987-0.998) | 0.996 (0.992-0.999) | 0.996 (0.990-0.999) | 0.997 (0.993-0.999) | 0.984 (0.964-0.995) | 0.991 (0.980-0.997) | 0.965 (0.924-0.988) | 0.987 (0.972-0.996) | 0.935 (0.861-0.978) | |||
CCH | Plaster | 0.999 (0.998-1.000) | 0.995 (0.990-0.999) | 0.999 (0.999-1.000) | 0.998 (0.996-0.999) | 0.998 (0.995-0.999) | 0.999 (0.997-1.000) | 0.996 (0.992-0.999) | 0.996 (0.991-0.999) | 0.994 (0.986-0.998) | 0.999 (0.997-1.000) | 0.998 (0.995-0.999) | 0.998 (0.995-0.999) |
DLP | 0.997 (0.993-0.999) | 0.992 (0.982-0.999) | 0.997 (0.994-0.999) | 0.993 (0.984-0.998) | 0.995 (0.989-0.998) | 0.994 (0.987-0.998) | 0.982 (0.960-0.994) | 0.987 (0.971-0.996) | 0.944 (0.879-0.981) | 0.992 (0.982-0.997) | 0.989 (0.976-0.996) | 0.985 (0.966-0.995) | |
JP | 0.996 (0.992-0.999) | 0.984 (0.965-0.995) | 0.993 (0.985-0.998) | 0.984 (0.964-0.995) | 0.983 (0.962-0.994) | 0.992 (0.982-0.997) | 0.984 (0.964-0.995) | 0.958 (0.908-0.986) | 0.968 (0.929-0.989) | 0.978 (0.952-0.993) | 0.979 (0.952-0.993) | 0.975 (0.944-0.992) | |
3DP | 0.998 (0.996-0.999) | 0.994 (0.987-0.998) | 0.996 (0.992-0.999) | 0.996 (0.990-0.999) | 0.997 (0.993-0.999) | 0.984 (0.964-0.995) | 0.991 (0.980-0.997) | 0.965 (0.924-0.988) | 0.987 (0.972-0.996) | 0.935 (0.861-0.978) |
As illustrated in the Bland-Altman plots, the agreement between the plaster and jetted photopolymer models was high for both clinical crown height ( Fig 3 ) and mesiodistal ( Fig 4 ) measurements. The mean systematic differences were −0.02 mm for clinical crown height measurements (limits of agreement, 0.37 to −0.41 mm) and −0.08 mm for mesiodistal measurements (limits of agreement, 0.34 to −0.49 mm).
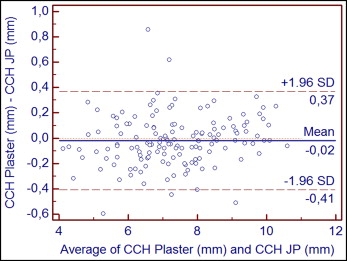
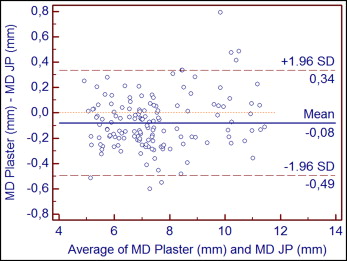
In addition, the Bland-Altman plots demonstrated that agreement between the plaster and the digital light processing models was also high for both clinical crown height ( Fig 5 ) and mesiodistal ( Fig 6 ) measurements. The mean systematic differences were 0.04 mm for clinical crown height measurements (limits of agreement, 0.34 to −0.26 mm) and −0.05 mm for mesiodistal measurements (limits of agreement, 0.34 to −0.45 mm).
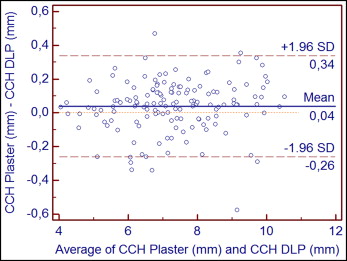
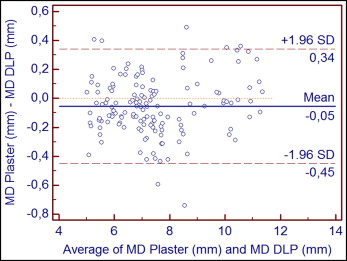
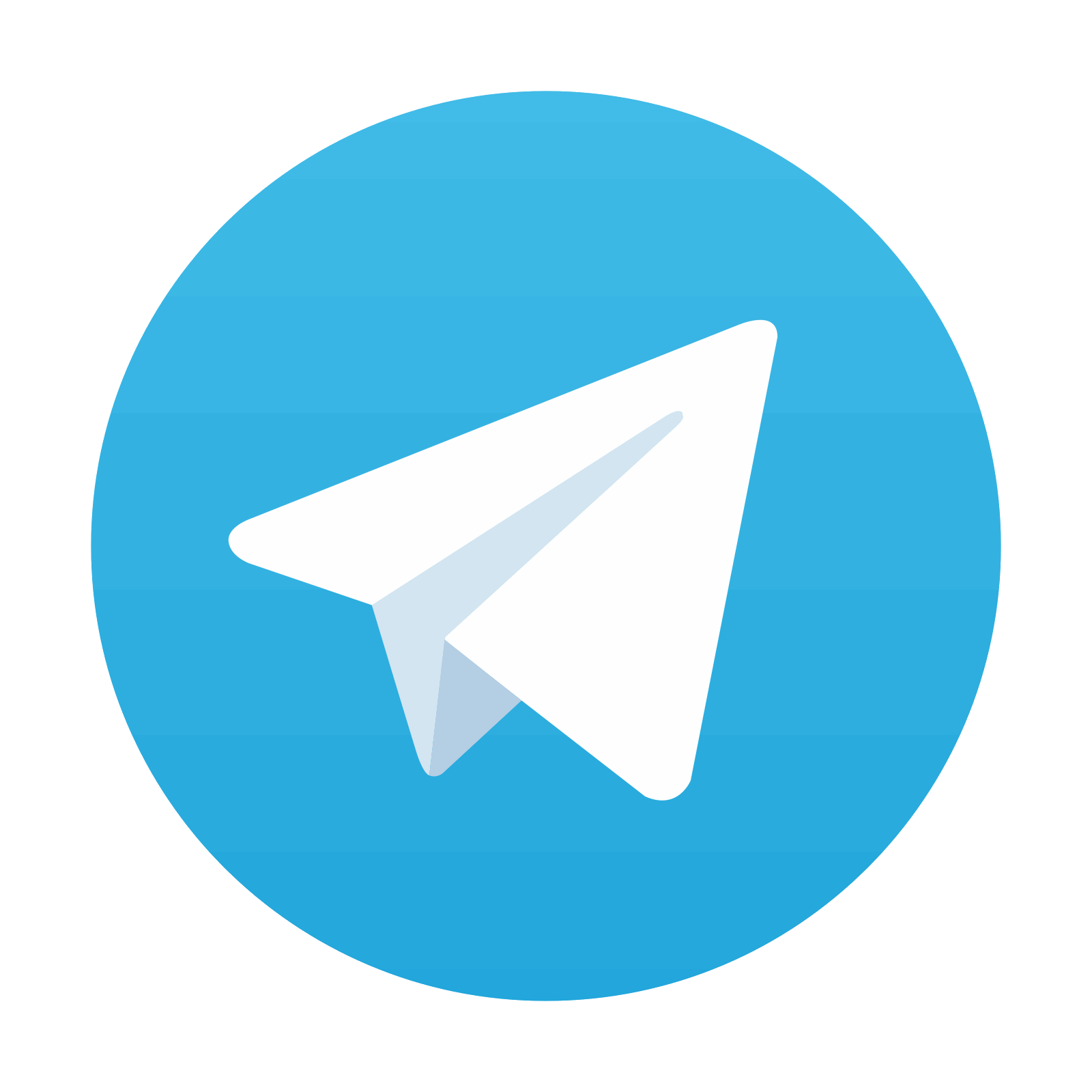
Stay updated, free dental videos. Join our Telegram channel

VIDEdental - Online dental courses
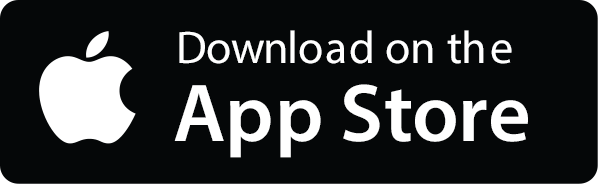
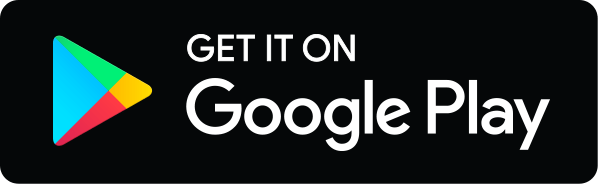
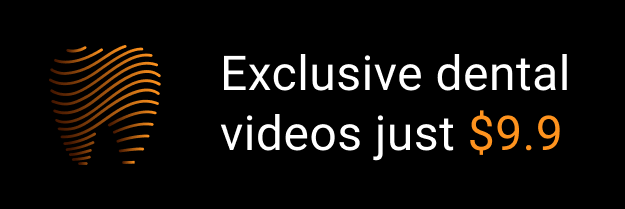