9
Root-End Fillings Using MTA
Seung-Ho Baek1 and Su-Jung Shin2
1 School of Dentistry, Seoul National University, Korea
2 College of Dentistry, Yonsei University, Gangnam Severance Hospital, Korea
INTRODUCTION OF ROOT-END FILLING MATERIALS
Purpose of root-end fillings
Surgical endodontic treatment is performed to resolve the inflammatory and/or procedural problems that cannot be treated by nonsurgical endodontic approaches.
The importance of placing a root-end filling for successful surgical endodontic treatment has been demonstrated in many clinical studies (Altonen & Mattila 1976; Lustmann et al. 1991; Rahbaran et al. 2001; Kim & Kratchman 2006). The major cause of periapical lesions that develop after nonsurgical endodontic treatment is apical leakage of bacteria and bacterial products from the contaminated canal space (Fig. 9.1). The removal of the infected periapical tissues by apical curettage without root-end filling does not eliminate the cause of Pathosis. The removal of the periapical lesion can only temporarily decrease the apparent symptoms and promote radiographic resolution of pathology. Periapical surgery entails not only the removal of the diseased periapical tissue and apex root, but also, resealing the root canal system.
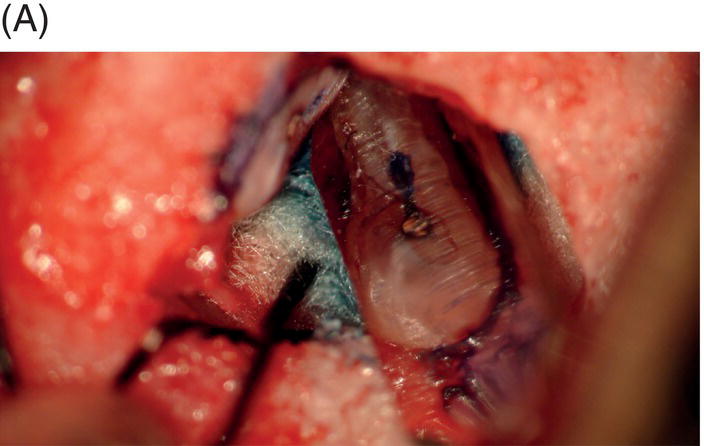
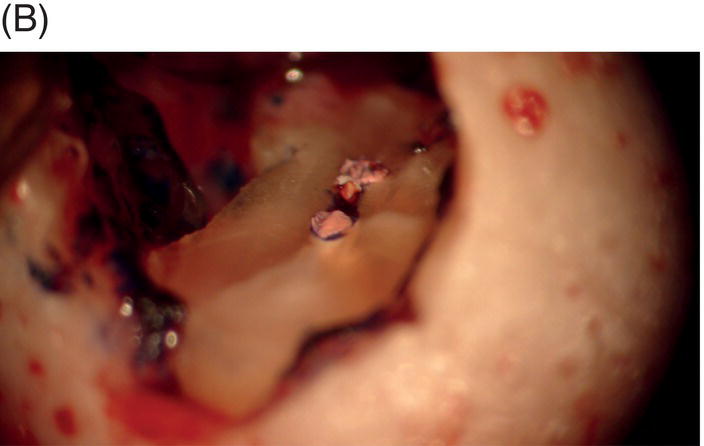
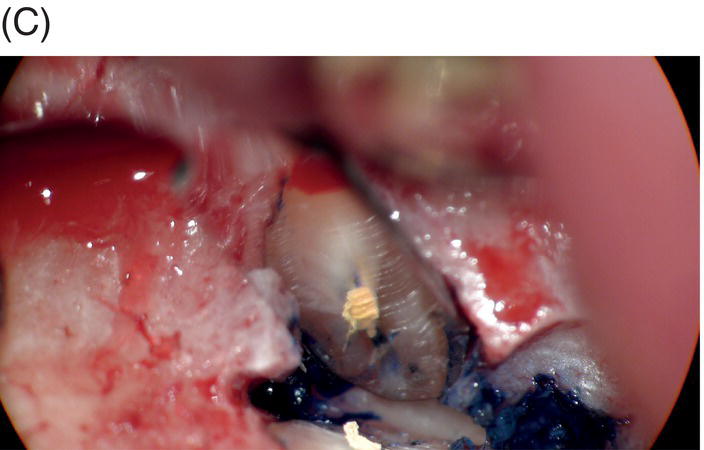
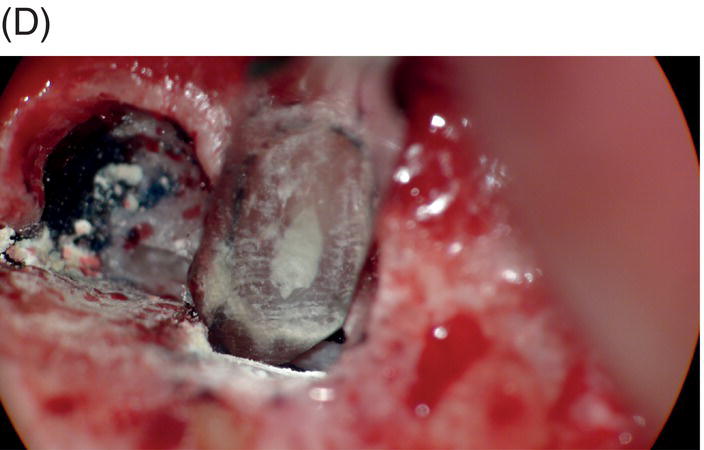
Fig. 9.1 Resected root surfaces that demonstrate apical leakage. (A,B) A 3-mm resected root surface, showing uncleaned areas in both canals. During apicoectomy, the unfilled canal space was examined with methylene blue staining. Although the curettage of apical granulation tissue and the removal of a root tip were performed, these bacterially contaminated surfaces can sustain periapical pathosis without proper retropreparation and root-end filling. (C,D) After a 3-mm root resection was completed, the resected surface was inspected under the microscope to investigate for isthmus presence, cracks or missed foramina. An unfilled canal space was observed, and MTA was applied into the retroprepared cavity.
There are some studies that have reported no significant differences in healing between teeth that were and were not root-end filled (Rapp et al. 1991; August 1996). Kim & Kratchman reported that the careful examination of these studies reveals many problems that are associated with their methodology and conclusions. First, the sample sizes are relatively small, leading to underpowered results. Second, the studies were conducted without microsurgical techniques and used amalgam as the root-end filling material. These old methods possessed inherent problems with steep angles of resection and the inconstancy of marginal adaptation of the root-end filling materials (Kim & Kratchman 2006). Therefore, the outcomes of the studies that are based on these conventional methods are less relevant for modern surgical endodontics.
The major cause of periapical lesions in many surgical cases may be due to leakage of bacteria and their toxins. Previous studies (Altonen and Mattila 1976; Lustman et al. 1991; Rahbaran et al. 2001) demonstrated the importance of root-end fillings in outcomes of apicoectomies by reporting that teeth with root-end fillings showed favorable results compared with those without root-end fillings. Therefore, sealing the root apex with a proper root-end filling material is crucial.
The purpose of the root-end filling is the hermetic sealing of the apex so that remaining bacteria or bacterial byproducts cannot enter or leave the canal.
HISTORY OF ROOT-END FILLING MATERIALS
A number of materials have been suggested as root-end filling materials including gutta-percha, amalgam, gold foil, zinc oxide eugenol (ZOE) cements, polycarboxylate cements, Cavit (3M ESPE, St. Paul, MN, USA), Diaket (ESPE GmbH, Seefeld, Germany), glass ionomer cements (GIC), composite resins, Intermediate Restorative Material (IRM, Caulk/Dentsply, Milford, DE, USA), SuperEBA (Bosworth, Skokie, IL, USA), and mineral trioxide aggregate (ProRoot MTA; Dentsply, Tulsa, OK, USA).
The root-end filling material is placed to fill the root-end cavity, which is prepared with an ultrasonic tip following root end resection. With an understanding of this specific environment in which a root-end filling material is placed, several requirements for an ideal filling material can be established. it is important that the filling materials provide an excellent seal and minimal toxicity to the surrounding tissues. MTA was initially introduced in the early 1990s as a root-end filling material for use in periapical surgery. MTA was initially developed by Dr. Torabinejad and introduced in the early 1990s as a root-end filling materials for use in periapical surgery (Torabinejad et al. 1993). Since then, its clinical applications have broadened to perforation repair, pulp capping, pulpotomy, and apexification (Torabinejad and Chivian 1999). Its popularity might be derived from its excellent sealing ability, biocompatibility, and possible bioactive properties, which may promote hard tissue formation (Torabinejad et al. 1993; Torabinejad et al. 1994; Koh et al. 1998; Torabinejad et al. 1995a; Torabinejad et al.1997). For the past 20 years, there have been numerous studies that aimed to investigate MTA’s physical, chemical, and biological properties, as well as its long-term clinical outcomes.
Amalgam
Amalgam has been used for over 100 years, and currently it is a widely used material in restorative procedures but rarely applied in apical retrofilling during apical surgery. in recent years, many have questioned the safety and integrity of amalgam in general and as a retrofilling material in particular, as it presents many disadvantages, including cytotoxicity, mercury toxicity, corrosion, delayed expansion, and amalgam tattoos embedded in soft and hard tissues (Fig. 9.2) (Dorn & Gartner 1990; Torabinejad et al. 1995a, 1997). The current use of amalgam as a root-end filling material is extremely limited (Chong & Pitt Ford 2005).
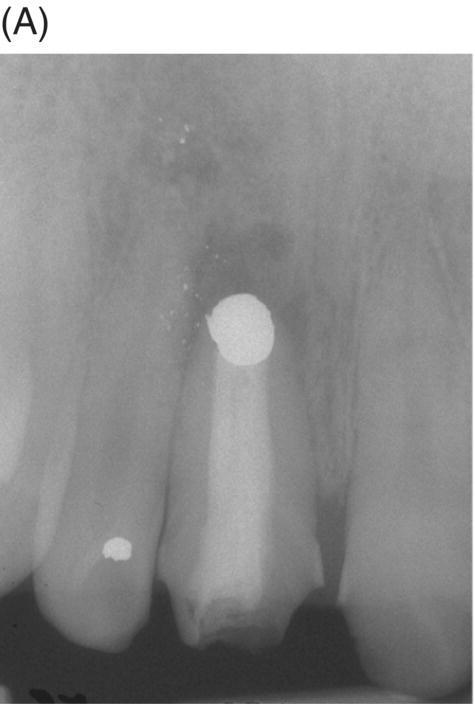
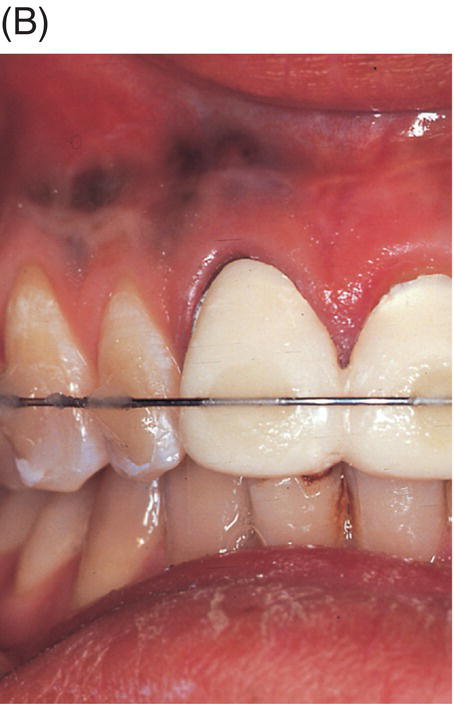
Fig. 9.2 An amalgam tattoo caused by an amalgam root-end filling. (A) A periapical radiograph demonstrated that an apicoectomy was performed on the maxillary right central incisor and a large root-end cavity was filled with amalgam. (B) An amalgam tattoo was broadly observed around the apical gingival area of the tooth.
Source: Seung-Jong Lee, Atlas of Endodontic Practice, 3rd edition, Yenang Inc., p. 385, 2007. Source: Lee et al. 2007. Reproduced with permission of Dr. Seung-Jong Lee.
ZOE-based materials: IRM and SuperEBA
IRM and SuperEBA have been used extensively, as they are considered clinically superior to amalgam as root-end filling materials. Both IRM and SuperEBA are modified forms of ZOE cements. The physical properties of ZOE cements, such as weak compressive and, tensile strengths, a long setting time, and solubility in oral fluids, are improved in both IRM and SuperEBA. They exhibit similar and favorable properties, and they are clinically and histologically superior to amalgam (Baek et al. 2005; Baek et al.2010; Dorn & Gartner 1990).
IRM is composed of 80% zinc oxide powder, 20% polymethylethacrylate, and a 99% eugenol liquid. The sealing ability of IRM is better than that of amalgam, and it is not affected by the liquid/powder ratio (Crooks et al. 1994). The biologic response of IRM is similar to that observed with other ZOE-based materials. IRM appears to be well tolerated in the periapical tissues, but its use like super EBA does not result in regeneration of periapical tissues (Pitt Ford et al. 1994; Harrison & Johnson 1997).
SuperEBA was first suggested as a root-end filling material by Oynick & Oynick (1978). It is composed of 60% zinc oxide powder, 30% alumina, and 6% natural resin, and the liquid is 37.5% eugenol and 62.5% ortho-ethoxybenzoic acid (Table 9.1; Dorn & Gartner 1990; Pitt Ford et al. 1995a; Trope et al. 1996). SuperEBA was shown to be superior to amalgam in terms of sealing ability, apical tissue compatibility, and regeneration potential (Pitt Ford et al. 1995a; Torabinejad et al. 1995c). Rubinstein & Kim reported that the healing success after root-end surgery was 96.4% after one year when SuperEBA was used as a root-end filling material in conjunction with microsurgical techniques (Rubinstein & Kim 1999).
Table 9.1 Composition of SuperEBA.
Powder | Liquid | ||
Zinc oxide | 60% | Eugenol | 37.5% |
Alumina | 34% | Ortho-ethoxy benzoic acid | 62.5% |
Natural resin | 6% |
Until MTA was developed, IRM and SuperEBA cements were the materials of choice for root-end fillings.
Resin-based materials: Retroplast and Geristore
Early studies claimed that resin materials exerted cytotoxic effects on periodontal ligament cells (Tai & Chang 2000; Huang et al. 2002). However, Rud and associates introduced Retroplast (Retroplast Trading, Dybersovej, Denmark] into endodontic surgery with favorable long-term outcomes (Rud et al. 1991, 1996; Yazdi et al. 2007). This material is placed on the concave root resected surface rather than being packed into a class I cavity. Retroplast has been mainly used in Europe. Similarly, Geristore (Den-Mat, Santa Maria, CA, USA) is a hybrid ionomer composite, and it has been used as a root-end filling material, especially in North America (Al-Sabek et al. 2005; Al-Sa’eed et al. 2008).
Al-Sabek and associates reported that human gingival fibroblasts attached and survived when in contact with Geristore, demonstrating less toxicity than IRM and Ketac-Fil (Al-Sabek et al. 2005). An in vitro study reported that extracts of both Geristore and Retroplast increased cell proliferation (Al-Sa’eed et al. 2008). However, conflicting results have been reported (Haglund et al. 2003; Tawil et al. 2009). In a study by Haglund and associates, Retroplast demonstrated decreased cell viability (Haglund et al. 2003). Compared with IRM and MTA, Geristore showed the least favorable histological results, even though there were no obvious radiographic differences between the materials (Tawil et al. 2009).
The major drawback of these resin-type materials is moisture sensitivity, especially to blood, during periapical surgery. Depending on the cavity design and hemorrhage control, the ideal conditions for bonding cannot be obtained in most surgical sites. Some researchers also have claimed handling difficulties with Geristore (Tawil et al. 2009).
Mineral trioxide aggregate (MTA)
MTA was introduced into surgical endodontic treatment as a root-end filling material by Torabinejad and associates in 1993 (Torabinejad et al. 1993). It was shown to have excellent sealing ability (Torabinejad et al. 1994, 1995e, f; Bates et al. 1996), an antimicrobial effect (Torabinejad et al. 1995d), and it promoted osteoblast activity (Torabinejad et al. 1995c; Koh et al. 1998). MTA was also less cytotoxic than amalgam, IRM, or SuperEBA (Torabinejad et al. 1995f; Keiser et al. 2000). The results of studies in dogs and monkeys showed that MTA caused significantly less inflammation than amalgam (Torabinejad et al. 1995a, 1997) when used as a root end filling. MTA also exhibited the most favorable periapical tissue response, as cementum was shown to regenerate in direct contact with the MTA (Torabinejad et al. 1997; Rubinstein & Kim 1999; Baek et al. 2005, 2010).
Gray vs. White MTA
MTA was originally formulated as a gray powder, but white MTA was later developed to overcome tooth discoloration by the gray formula (Dammaschke et al. 2005). However, recent studies have demonstrated that white MTA also induces the discoloration (Felman & Parashos 2013; Camilleri 2014). When the sealing ability of the gray and white MTA was compared, there was no significant difference (Shahi et al. 2007).
There have been controversies among studies that examined whether there were any differences in biocompatibility between the gray and white MTA. Holland and associates evaluated the reaction of rat subcutaneous connective tissue to the implantation of dentin tubes filled with white MTA (Holland et al. 2002). They showed similar results to those reported for gray MTA, which indicated that white and gray MTA have similar mechanisms of action. On the other hand, a cell culture study by Perez and associates showed that osteoblasts behave differently on white MTA, suggesting that this might be due to the surface morphology of the materials (Perez et al. 2003). Most studies reported no significant difference in the biocompatibility between the two variants (Camilleri et al. 2004; Ribeiro et al. 2005; Shahi et al. 2006).
New types of MTA-like cements
Other types of MTA-like materials have been developed and marketed, including MTA angelus (Angelus, Londrina, PR, Brazil), MTA bio (Angelus, Londrina, PR, Brazil), CPM (Egeo, Buenos Aires, Argentina), Endosequence Root Repair Material (RRM) (Brasseler USA, Savannah, GA, USA), OrthoMTA (bioMTA, Seoul, South Korea), and Endocem MTA (Maruchi, Seoul, South Korea). Recent studies have compared these materials with ProRoot MTA. However, one of the major drawbacks of these relatively new products is the lack of clinical data to advocate long term successful outcomes.
REQUIREMENTS OF IDEAL ROOT-END FILLING MATERIALS
The ideal root-end filling material should be easy to manipulate, radiopaque, dimensionally stable, bactericidal or bacteriostatic, nonresorbable, and unaffected by the presence of moisture (Gartner & Dorn 1992). It should also adhere to the prepared cavity walls, seal the root canal system, promote healing, and it should be nontoxic and well-tolerated by periapical tissues (Table 9.2). Many studies have examined the sealing ability and biocompatability of retrograde filling materials, but none of these root-end filling materials, including MTA, fully satisfy these requirements (Aqrabawi 2000).
Table 9.2 Requirements of the ideal root-end filling material (Gartner & Dorn 1992).
Easy to manipulate (proper working time) Radiopaque Long-term dimensional stability Nonresorbable Unaffected by moisture Adhere to the dentin wall Biocompatible Bactericidal or bacteriostatic Seal the root canal system (sealability) Well-tolerated by periapical tissues (biocompatibility) Ability to regenerate periapical tissues (bioactive) Inexpensive |
Advantages and disadvantages of MTA as a root-end filling material
Advantages of MTA
According to numerous previous studies (Torabinejad et al. 1995a, c, f, 1997; Trope et al. 1996; Baek et al. 2005, 2010; Chong & Pitt Ford 2005), MTA is superior or equivalent to other materials in terms of biocompatibility, sealing ability, and bactericidal properties. from a biological perspective MTA has a great advantage over other available materials. In addition to its excellent biocompatibility, MTA comes close to being an ideal filling material, as it has the capacity to promote bone, dentin, and cementum regeneration (Baek et al. 2005; Pitt Ford et al. 1995b). These outstanding features will be discussed later in this chapter.
Most dental filling materials exhibit the best results when they set in a dry environment or when moisture is properly controlled. MTA consists of hydrophilic powder and sets under wet conditions, except in environments with excessive bleeding or water contact. This property (insensitivity to the presence of moisture) is advantageous in most surgical conditions because it is almost impossible to obtain a completely dry environment, despite efforts to control hemorrhage with pressure and cotton pellets.
Disadvantages of MTA
MTA is known to be difficult to place into the prepared root-end cavities. In addition to these handling difficulties, freshly mixed MTA can be washed out whenever there is a significant communication between the oral cavity and MTA, limiting its periodontal applications. In situations without obvious communications, the blood flow in the surgical site will cause a certain amount of cement loss (Formosa et al. 2012).
MTA does not directly bond or adhere to bony or dental structure. The setting time of MTA is approximately 3–4 hours (Torabinejad et al. 1995b), which is considered to be a disadvantage in many clinical situations. The long setting time may evoke an increased solubility, exhibiting a detrimental effect on sealing ability. During apicoectomy, MTA might be exposed to an acidic environment, and the sealing ability of MTA under acidic conditions is controversial. Roy and associates reported that acidic environments did not hinder the sealing ability of MTA (Roy et al. 2001). On the other hand, the more extensive porosity of MTA was observed when it set in direct contact with acid (Namazikhah et al. 2008), and more leakage was observed in MTA when stored in low-pH solution (Saghiri et al. 2008).
To circumvent these problems, additives such as methylcellulose, calcium chloride, and dibasic sodium phosphate were employed to shorten the original setting time of MTA (Ber et al. 2007; Bortoluzzi et al. 2008; Huang et al. 2008). the mixing of MTA powder with different liquids may possibly alter other properties of the material. Adding calcium chloride solution might lower the final compressive strength relative to that of a mixture with sterile water (Kogan et al. 2006).
Another issue is its high cost, as many researchers and clinicians find that MTA is an expensive material (Casas et al. 2005; Mooney & North 2008).
The advantages and disadvantages of MTA are summarized in Table 9.3.
Table 9.3 Advantages and disadvantages of mineral trioxide aggregate (MTA).
Advantages | Disadvantages |
Low cytotoxicity | Difficult to manipulate |
Excellent biocompatibility | Long setting time |
Hydrophilic | Cost |
Radiopaque | |
Sealing ability | |
Bioactive |
MTA AS A ROOT-END FILLING MATERIAL
Cytotoxicity and biocompatibility
The most critical properties of root-end filling materials that come into contact with periapical and periodontal tissue are their cytotoxicity and biocompatibility. The biocompatibility of MTA is important to create an environment conducive to healing and bone fill.
Cytotoxicity of root-end filling materials has been evaluated by various methods, such as cell viability assays for mitochondrial dehydrogenase activity, an agar overlay method, and the evaluation of cell attachment and morphology. The cytotoxicity and biocompatibility of MTA were tested in in vitro cell culture studies, and the results showed the satisfactory attachment of osteoblasts and periodontal cells on MTA. In numerous previous studies, MTA has shown less or equivalent cytotoxicity compared with other root-end filling materials including amalgam, ZOE, IRM, composite resin and GIC. (Zhu et al. 2000; Balto 2004; Yoshimine et al. 2007; Bodrumlu 2008).
The cytotoxic effect on nerves and neural cells has also been measured to evaluate whether a root-end filling material inhibits nerve integrity or regeneration. When neurotoxicity was tested with several root-end filling materials, amalgam, SuperEBA, and Diaket induced neural cell death, while MTA did not (Asrari & Lobner 2003).
Most early studies were performed with a premixed MTA. It was suspected that freshly mixed MTA might create a detrimental environment for cells because it has a high pH during setting (Balto 2004). An inhibition zone was often observed in cell cultures that were grown in contact with fresh MTA. However, this cell-free zone seems to be transient (Fig. 9.3A, B). When fresh MTA was inserted in vivo, no evidence of a cell-free zone was reported (Pitt Ford et al. 1996; Apaydin et al. 2004). When the cytotoxicity of MTA, amalgam, and SuperEBA were measured, fresh MTA showed the least degree of toxicity compared with the other materials. The initial toxicity of MTA before setting may not create a detrimental environment when it is applied into a root-end cavity (Lustmann et al. 1991).
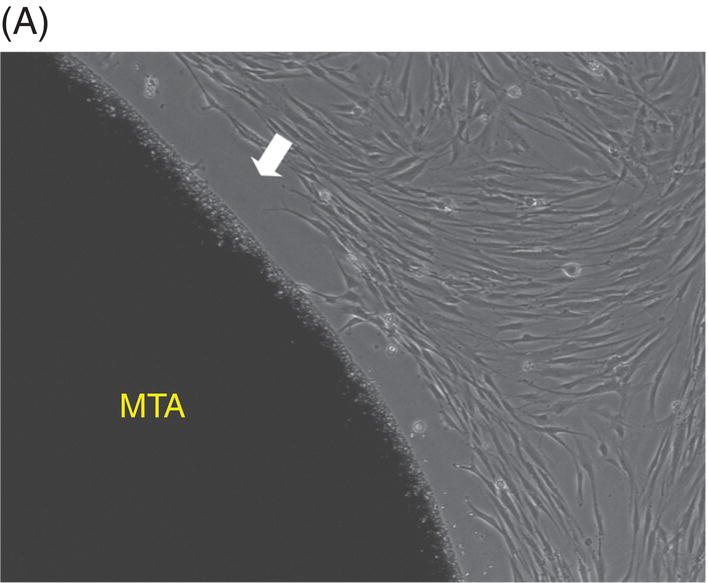
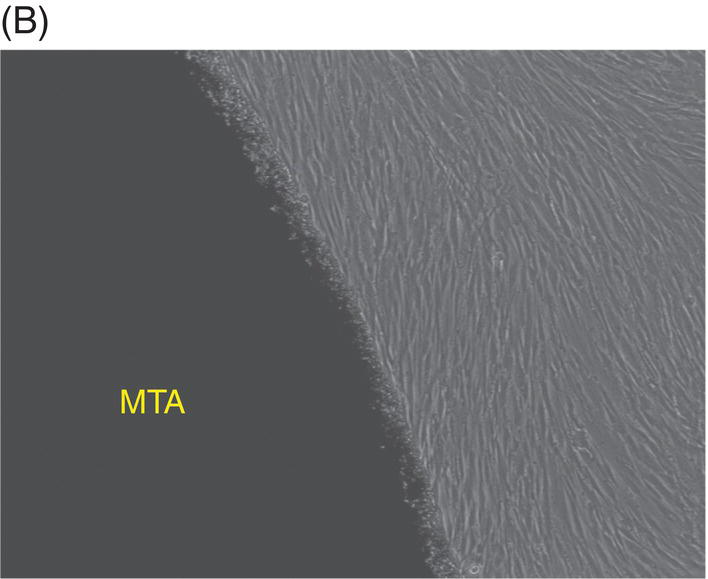
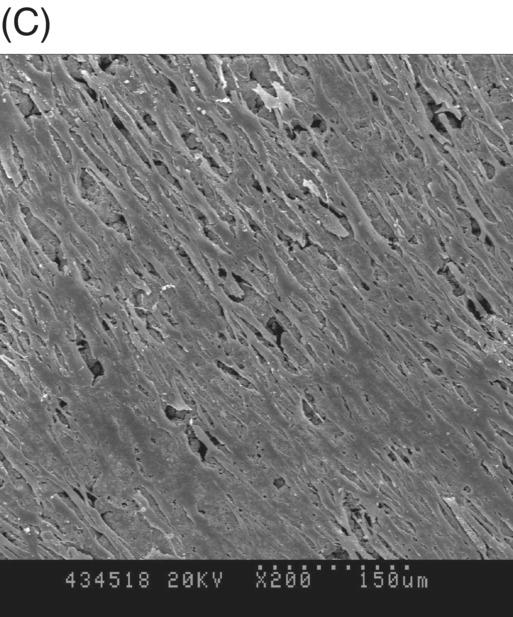
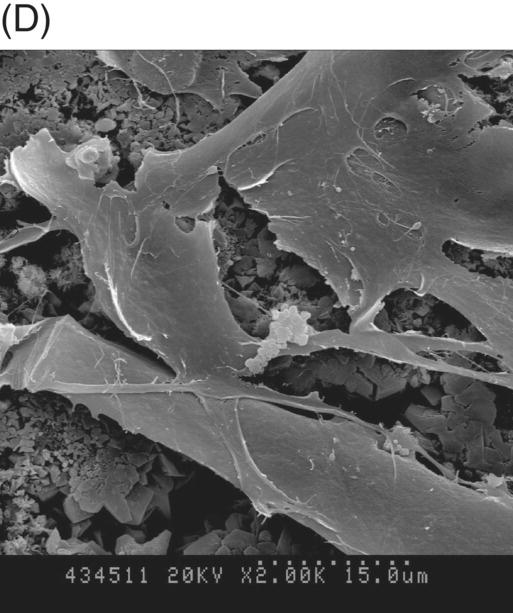
Fig. 9.3 Phase microscopy and scanning electron microscopy (SEM) showing human periodontal ligament (PDL) cells grown on freshly prepared white MTA for 48 (A) and 72 (B–D) hours. (A) The white arrow indicates the cell-free zone between the margin of the MTA and the cells. (B) No inhibition zone between MTA and cells was observed in 72-hour samples. (C) Cells were attached and healthy when they were plated on MTA />
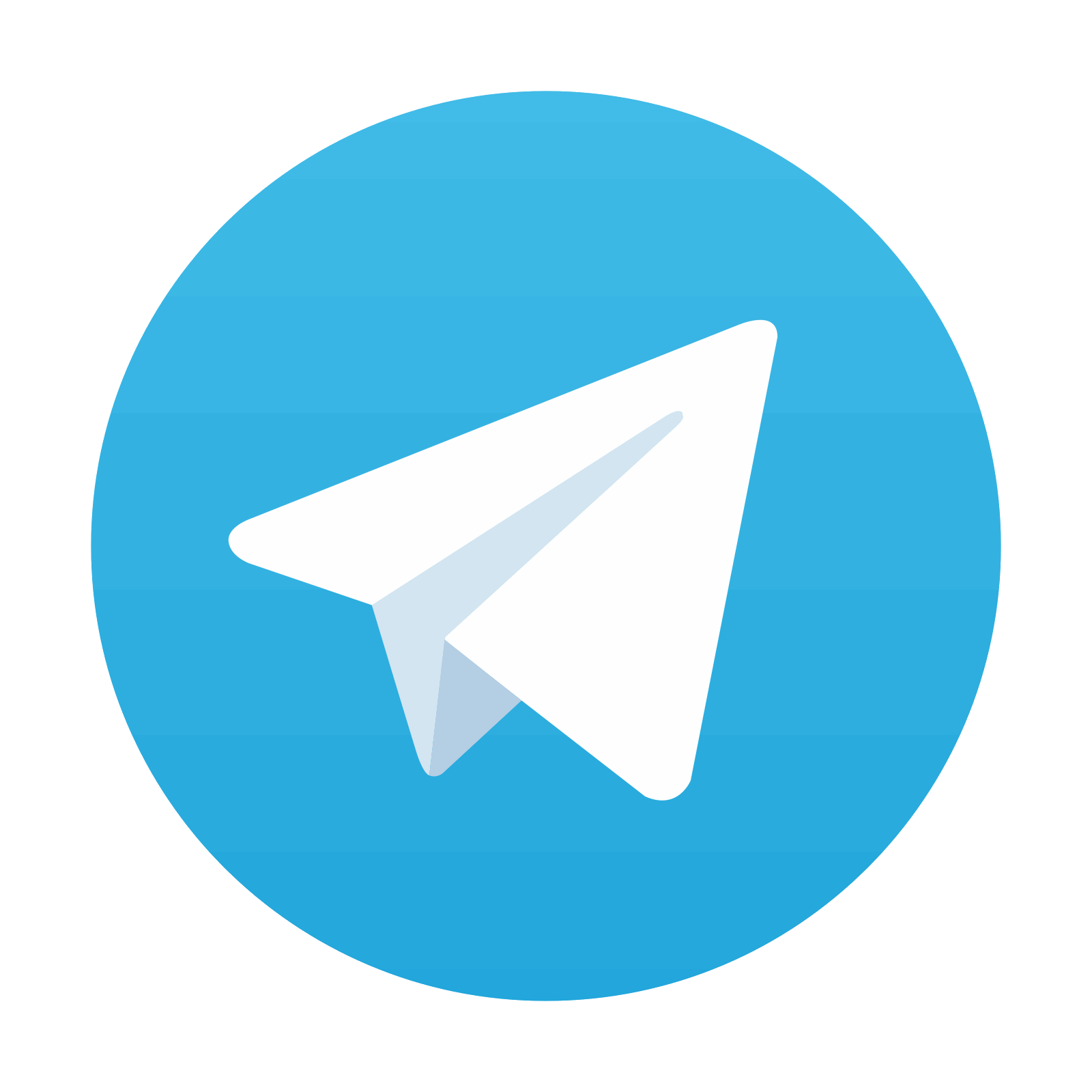
Stay updated, free dental videos. Join our Telegram channel

VIDEdental - Online dental courses
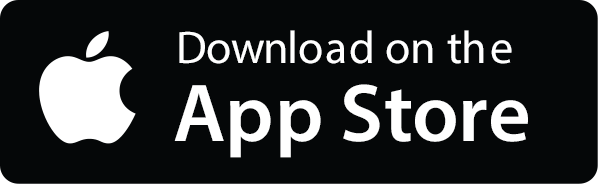
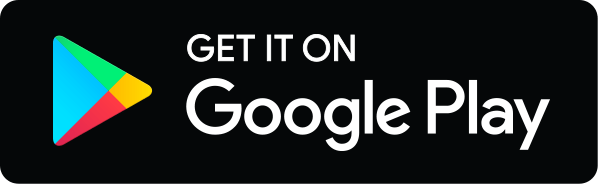