8
Barrier functions of oral mucosa
The important function of the oral epithelium in protecting the underlying tissues and glands of the oral mucosa has already been mentioned in Chapter 1. This protective role includes the ability to resist mechanical damage resulting from activities such as mastication, to limit the entry of toxins and microorganisms present in the oral cavity, and to mount an immunoprotective response should they do so.
The patterns of differentiation in oral epithelium that produce a surface layer with appropriate properties to resist the forces to which it is subject have been described in detail in Chapter 3. These are principally keratinization, in which surface-cornified cells become filled with a compact array of condensed cytokeratin filaments, bounded by a thickened cell envelope and surrounded by an external lipid matrix, and non-keratinization in which the mature cells in the outer portion of the epithelium become large and flat and possess a cross-linked protein envelope, but retain nuclei and other organelles, and the cytokeratins do not aggregate to form bundles of filaments as seen in keratinizing epithelia. This chapter will describe the characteristics of the permeability barrier and immunoprotective responses in oral mucosa.
8.1 THE PERMEABILITY BARRIER
As cells leave the basal layer and enter into differentiation, they become larger and begin to flatten and to accumulate lipids. A portion of the accumulating lipid is packaged in small organelles known as membrane-coating granules or lamellar granules that become evident in the prickle cell layer (see Fig. 8.1) (Martinez and Peters, 1971). At the boundary between the granular and cornified layers, the membrane-coating granules migrate to the superficial (apical) aspect of the keratinocyte, where the bounding membrane of the organelle fuses with the cell plasma membrane so that the lipid lamellae are extruded into the extracellular spaces of the surface layer (Landmann, 1988). Here it undergoes enzymatic processing to produce a lipid mixture consisting of ceramides, cholesterol, and fatty acids. This intercellular lipid is uniquely organized into a multilamellar complex that fills most of the intercellular space of the stratum corneum. The barrier properties of the stratum corneum are related to the phase behavior of the intercellular lipids. Thus, the membrane-coating granules are believed to be responsible for the formation of the superficial permeability barrier in stratified squamous epithelium; the evidence for this is presented in a subsequent section.
Figure 8.1 Diagram to show the major structural changes during maturation and formation of the permeability barrier in keratinized epithelium.
Insert: Membrane-coating granules are formed in the Golgi region (gr) in the prickle cell layer and migrate to the superficial cell membrane as cells reach the granular layer. Here, the membrane of the granule (gcm) fuses with the cell plasma membrane, and the lipid lamellae (lb) are extruded into the intercellular space and become rearranged into intercellular lamellae (icl) that lie parallel to the horny cell envelope (hec) of the keratinized cell, containing keratin filaments (kf). We are grateful to Dr. Philip Wertz for providing the insert.
In the epidermis, the short stacks of lipid lamellae that are extruded from the membrane-coating granules fuse at the edges to produce multiple broad lipid sheets which fill most of the extracellular spaces of the stratum corneum; in keratinized oral epithelium, about 50% of the intercellular space of the stratum corneum is occupied by desmosomes (Schroeder, 1981), and the interdesmosomal regions are frequently dilated. Although the extruded membrane-coating granule contents fuse to form multiple broad lipid sheets in the intercellular spaces of the stratum corneum of this tissue, the number of individual lamellae in oral tissue is less than that observed in epidermis.
The major lipid classes present in epidermal and oral stratum corneum are similar (Law et al., 1995), but the proportions of these lipids differ, and there is nearly an order of magnitude difference in barrier function as judged by permeability to water. Lipid composition will also influence the organization of the barrier lipids.
Although multiple, broad, lamellar sheets are present in both epidermal and oral stratum corneum, the highly ordered appearance of the multiple lipid sheets seen in epidermal stratum corneum is not observed in oral stratum corneum. In the oral tissue, the interdesmosomal portions of the intercellular space tend to be dilated with a few broad lamellae at the periphery of the dilation and less well-organized lamellae and non-lamellar material, possibly consisting of desmosomal breakdown products, filling the center of the dilation.
In non-keratinized oral epithelia (see Fig. 8.2), the accumulation of lipids is less evident than in keratinized epithelia. As cells reach the upper one-third to one-quarter of the epithelium, membrane-coating granules become evident at the superficial aspect of the cells and appear to fuse with the plasma membrane so as to extrude their contents into the intercellular space. The membrane-coating granules found in non-keratinizing epithelia are spherical in shape, membrane-bounded, and measure about 0.2 µm in diameter (Squier, 1977). They have often been referred to as cored granules because of their appearance in transmission electron micrographs of conventional, osmium tetroxide-fixed, tissue. Such granules have been observed in a variety of human non-keratinized epithelia, including oral mucosa, uterine cervix (Grubb et al., 1968), and esophagus (Hopwood et al., 1978). Only a small proportion of the granules in non-keratinized epithelium contain lamellae, which may be the source of short stacks of lamellar lipid scattered throughout the intercellular spaces in the outer portion of the epithelium (Wertz et al., 1993).
Figure 8.2 Diagram to show the major structural changes during maturation and formation of the permeability barrier in non-keratinized epithelium. Membrane-coating granules containing short stacks of lipid lamellae and electron-lucent material migrate to the superficial cell membrane of cells in the intermediate layer of the epithelium. The membrane of the granule fuses with the cell plasma membrane and the lamellate contents are extruded into the intercellular space as shown in the electron micrograph in the top right panel. We are grateful to Dr. Philip Wertz for providing the panel insert.
In contrast to the appearance of the intercellular spaces of the surface layer of keratinized epithelia, those of the superficial layer of non-keratinizing epithelia contain electron-lucent material, which may represent non-lamellar phase lipid, with only occasional short stacks of lipid lamellae. It is the absence of organized lipid lamellae in the intercellular spaces that accounts for the greater permeability of this tissue. It seems likely that the lamellae seen in the intercellular spaces in the non-keratinizing epithelia are derived from the lamellar material in the membrane-coating granules, and it is possible that these lamellae might have a lipid composition distinctly different from the overall composition. If these structures are enriched in ceramides, cholesterol, and fatty acids and are well ordered, they could account for much of the diffusional resistance of these tissues to aqueous penetrants.
It is also noteworthy that epidermis and all of the oral epithelia synthesize appreciable quantities of glycosylceramides (Squier et al., 1991) but differ in the extent of deglycosylation in the formation of their barriers. In epidermis, where the barrier is most effective, more than 98% of the sphingolipid in the barrier was ceramide. In gingival and palatal stratum corneum, 73% and 77%, respectively, of the sphingolipid had been converted to ceramides, whereas in the barriers from the buccal epithelium and the floor of the mouth, only about 5%–6% of the glycosylceramides had been converted.
8.1.1 Membrane-Coating Granules and the Location of a Permeability Barrier in Oral Mucosa
Intercellular tracers such as horseradish peroxidase or lanthanum that can be visualized in the light or electron microscope have been used to demonstrate the location of the permeability barrier in oral mucosa (Squier, 1973; Squier and Rooney, 1976). In keratinized mucosa, the limit of penetration of the tracers was at the boundary of the granular and keratinized layers, as in epidermis. When the procedure was carried out using non-keratinized oral mucosa, the tracers failed to penetrate the outer one-third to one-quarter of the epithelium. This coincided with the level at which the membrane-coating granules of this tissue appear to fuse with the superficial cell membrane and extrude their contents into the intercellular space. Although these granules differ morphologically from those of keratinized epithelium, they appear to be homologous with the granules of keratinized epithelia in their location and behavior (Squier, 1977).
If the presence of membrane-coating granules in a stratified squamous epithelium is a prerequisite for the formation of a permeability barrier, then tissues from which they are absent might be expected to lack such a barrier. Epithelial cells from skin and keratinizing oral mucosa maintained in a submerged culture system show poor differentiation, and membrane-coating granules have rarely been described in ultrastructural studies. When such cultures are treated topically with horseradish peroxidase, it readily penetrates between the cells of the superficial layer, indicating that a permeability barrier is not present (Squier et al., 1978). The use of raised (interface) cultures has been found to facilitate epithelial differentiation, including the development of membrane-coating granules in epidermis (Madison et al., 1988) and oral epithelium (Lillie et al., 1988). The permeability of such a system tends to be slightly higher than that of skin in vivo (Cumpstone et al., 1989), although equivalence has been reported (Cannon et al., 1994). Thus, in the junctional epithelium of the tooth, and in tissue culture systems, the presence or absence of membrane-coating granules can be related to the permeability properties of the tissue.
8.1.2 Other Permeability Barriers in Oral Mucosa
Although the superficial layers of the oral epithelium represent the primary barrier to the entry of substances from the exterior, it is evident that the basement membrane also plays a role in limiting the passage of materials across the junction between epithelium and connective tissue (Alfano et al., 1977). Thus, intravenously injected horseradish peroxidase can enter the intercellular spaces of the epidermis, but the passage of the larger molecules of the protein, thorotrast, is restricted (Wolff and Honigsmann, 1971). A similar mechanism appears to operate in the opposite direction. When labeled albumin is applied to the surface of the oral mucosa of animals sensitized to this protein, immune complexes that are formed in the epithelium are trapped above the basement membrane, suggesting that whereas immunoglobulins can traverse this region, the larger immunocomplexes do not (Tolo, 1974). The accumulation of materials in the basal region may represent the nonspecific binding of charged molecules to components of the basal lamina.
8.1.3 Aging and the Permeability of Oral Mucosa
As discussed in Chapter 7, the oral mucosa shows few changes that can be unambiguously ascribed to aging. In some regions, there is a slight thinning of the epithelium with a concomitant flattening of the epithelial–connective tissue interface (Williams and Cruchley, 1994). The limited information available on the permeability of oral mucosa indicates that there is a trend toward decreased permeability to water with age which is statistically significant for floor of mouth mucosa from females (Squier et al., 1994). It is of interest to note that in skin, where the morphological changes with age are more marked than in oral mucosa, there have been a number of reports which demonstrate a significant decrease in permeability with age; the reasons for this have been discussed by Squier et al. (1994).
8.1.4 The Permeability Barrier and Reactive Changes in the Oral Mucosa
Inflammation
The most common mucosal reaction to insult or disease is inflammation. The abundant vasculature ensures rapid infiltration of the tissue with inflammatory cells in response to mechanical or chemical insult. A mild degree of inflammation stimulates epithelial proliferation, leading to hyperplastic changes, whereas severe inflammation suppresses proliferation (Johnson, 1994) so that the epithelium may become thinner or even be lost (i.e., ulcerated).
A common site for mucosal inflammation is under dentures. Riber and Kaaber (1980) found inflammatory changes of the palatal mucosa after 12 months in one-third of a group of denture wearers. Permeability measurements on the inflamed tissue under the dentures revealed a threefold increase in permeability as compared to values for normal palate (Riber and Kaaber, 1978). This effect seemed to be associated with a shift in epithelial differentiation in the presence of chronic inflammation toward that of non-keratinization with a concomitant increase in permeability.
8.1.5 Permeability Barrier Function and Extrinsic Factors
A variety of extrinsic factors can bring about changes in the oral mucosa that have a potential for altering permeability. The most frequent of these is irritation from physical damage or from chemical irritants such as tobacco and tobacco smoke, alcohol, toothpastes, and mouth rinses. Assuming that inflammation is not severe (see previous section), oral epithelium responds to irritation by an increase in cell proliferation that results in a thickening of the tissue; keratinized regions tend to show a thickening of the keratinized layer (hyperkeratinization), and non-keratinized regions frequently develop a keratinized layer in these circumstances. The most dramatic hyperkeratotic changes in the oral mucosa are seen in the palate of smokers and at sites in the mouth such as the cheek, inner lip, and gums, where users of snuff or chewing tobacco place the product. These reactive changes presumably offer greater mechanical protection to the tissue but do not appear to improve its barrier properties. Indeed, hyperkeratotic hamster cheek pouch, induced by chemical or mechanical means, shows permeability values that are either no different from, or are greater than, normal tissue (Squier and Hall, 1985). Such findings are consistent with data from skin, where the thickened epidermis of the palmar and plantar regions has a higher permeability than thin skin (Kligman, 1964) and where the pathological thickening that is evident in conditions such as psoriasis and in various ichthyoses, results in an increased permeability to water (Grice, 1980). The reduced barrier function in conditions of epithelial thickening is probably a reflection of the increased cell proliferation and faster epithelial transit time characteristic of hyperplasia; this reduces the opportunity for membrane granule extrusion and adequate formation of the intercellular barrier material.
8.2 IMMUNOLOGIC BARRIER FUNCTION OF ORAL MUCOSA
Microorganisms in food, water, and air are a formidable challenge to the oral lining. At times, oral mucosal surfaces are exposed to high concentrations of environmental microorganisms and/or their products in respirable air, saprophytic microorganisms on foodstuffs, and microorganisms in water. Microorganisms residing in the oral cavity are also a burden; there are more than 500 diverse phylotypes of bacteria present in saliva (approximately 108 microorganisms/mL) and dental plaque (approximately 1011 microorganisms/gram) (Aas et al., 2005). Oral tissues respond to the continual exposure to these microorganisms by creating immunologic barriers via innate and adaptive immune responses. This limits local infection, colonization, invasion, and inflammation by potential pathogens. The immunologic barrier defined by the innate immune response is prominent and consists of antimicrobial peptides, antimicrobial proteins, chemokines, cytokines, and neuropeptides, which are found in the oral tissues, saliva, and gingival crevicular fluid.
Antimicrobial peptides are small, potent molecules composed of anionic or cationic amino acid residues with broad spectrum antibiotic-like activities (Brogden, 2005). They also have many ancillary innate and adaptive immune functions (Yang et al., 2004). Antimicrobial peptides differ in size, amino acid residue composition, and mechanism of antimicrobial activity. These peptides are expressed in many tissues of the oral cavity. They have potent antimicrobial activity, neutralize lipopolysaccharide, promote wound healing, and have synergistic activity with conventional antibiotics. Antimicrobial proteins are larger and contain more than 100 amino acid residues, are often lytic enzymes, nutrient-binding proteins, or they contain sites that target specific microbial macromolecules (Ganz, 2003). Examples include lactoferrin and lysozyme; both are found in saliva and gingival crevicular fluid. Cytokines and chemokines have important roles in regulating and directing both innate and adaptive immune responses. Neuropeptides are peptides with neural or neuroendocrine signaling functions. Interestingly, some cytokines, chemokines, and neuropeptides also have direct antimicrobial activity contributing to the innate immune response barrier (Brogden et al., 2005).
8.2.1 Antimicrobial Peptides and Immune Mediators in Oral Tissues
Oral tissues, including salivary glands, produce antimicrobial peptides, antimicrobial proteins, cytokines, and chemokines. The specific expression profiles for cells isolated from oral tissues are listed in Table 8.1. mRNA for human β-defensin 1 is strongly expressed in the broad spinous cell region and localized throughout the nucleated layers of the epithelium (Dale et al., 2001), and the peptide is present in the well-differentiated cells of the upper spinous and granular layers. mRNA for human β-defensin 2 is induced in the spinous and granular layers of the epithelium, and the peptide is present in the upper granular layers of epithelium with spotty presence in the spinous layers. Human β-defensin 3 is expressed in the basal layer in healthy tissue, occasionally extending into the spinous layers and upper layers (Lu et al., 2005). Human neutrophil peptide (HNP) α-defensins 1–3 and the cathelicidin LL-37 are both found in polymorphonuclear leukocytes in the connective tissue and junctional epithelium (Dale et al., 2001).
Table 8.1 Prominent mediators produced by individual cells in the oral cavity.
aDiamond et al. (2001), and Krisanaprakornkit et al. (1998).
bBickel et al. (1996), Formanek et al. (1999), Joly et al. (2005), Mathews et al. (1999), Ohta et al. (2008), and Xu et al. (2009).
cImatani et al. (2001), Ohta et al. (2008), Scheres et al. (2010), and Yamaji et al. (1995).
dCutler and Teng (2007), and Pingel et al. (2008).
eAltstaedt et al. (1996), Gudmundsson et al. (1996), Kasama et al. (2005), and Rodriguez-Garcia et al. (2010).
Myeloid dendritic cells produce a great variety of cytokines involved in T-helper (Th) cell responses for cell-mediated immunity (Th1 cytokines), humoral immunity (Th2 cytokines), autoimmunity and inflammation (Th17 cytokines), proinflammatory cytokines, anti-inflammatory cytokines, and chemokines. Human gingival epithelial cells, keratinocytes, and oral fibroblasts produce a lesser variety of proinflammatory cytokines and chemokines. Polymorphonuclear leukocytes can also produce cytokines, generally in response to inflammatory stimuli (Kasama et al., 2005).
8.2.2 Antimicrobial Peptides and Immune Mediators in Oral Secretions
Many of the antimicrobial peptides, cytokines, and chemokines/>
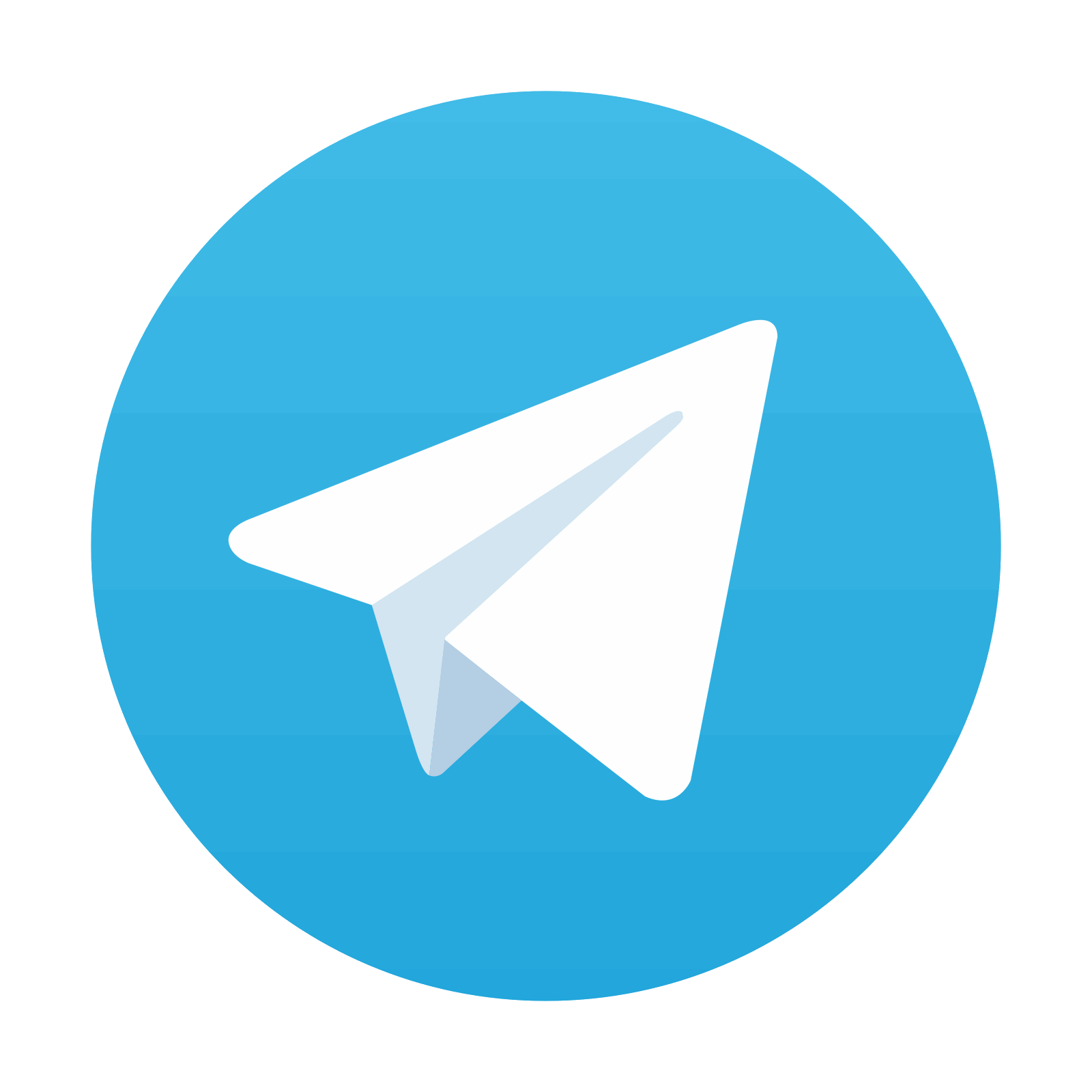
Stay updated, free dental videos. Join our Telegram channel

VIDEdental - Online dental courses
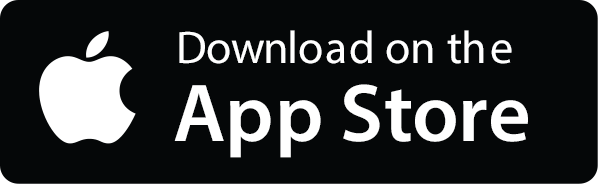
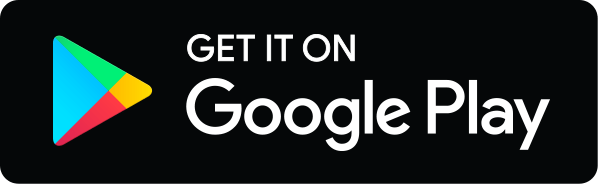