Development of the Tooth and Its Supporting Tissues
Primary Epithelial Band
Chapter 3 explains how, after about 37 days of development, a continuous band of thickened epithelium forms around the mouth in the presumptive upper and lower jaws. These bands are roughly horseshoe-shaped and correspond in position to the future dental arches of the upper and lower jaws (Figures 5-1 and 5-2, A and B). The formation of these thickened epithelial bands is the result not so much of increased proliferative activity within the epithelium as it is a change in orientation of the mitotic spindle and cleavage plane of dividing cells (Figure 5-2, C). Each band of epithelium, called the primary epithelial band, quickly gives rise to two subdivisions which ingrow into the underlying mesenchyme colonized by neural crest cells. These are the dental lamina, which forms first, and the vestibular lamina, which forms shortly afterward and is positioned just in front of the dental lamina.
A key feature of the initiation of tooth development is the formation of localized thickenings or placodes within the primary epithelial bands (Figure 5-3, A). Dental placodes are believed to initiate formation of the various tooth families. It is noteworthy that placodes morphologically similar to those of teeth also initiate the development of other ectodermal appendages, such as hair and feathers. The basic mechanisms and genes involved in the formation and function of all placodes are similar. The balance between stimulatory (FGFs, Wnts) and inhibitory signals (BMPs) is important in determining the site of placodes. Formation and growth of placodes is believed to involve the transcription factor p63, TNF, and ectodysplasin (Eda), among others. Defects in these pathways lead to ectodermal dysplasias characterized by missing teeth (oligodontia), and misshapen teeth (Figure 5-3, B). On the other hand, overactivation of the Eda receptor leads to extra teeth with aberrant morphology. In conclusion, placode formation is a determinant event in tooth development. Smaller-than-normal placodes lead to missing and smaller teeth, whereas larger placodes induce supernumerary and larger teeth.
Vestibular Lamina
If a coronal section through the developing head region of an embryo at 6 weeks of development is examined, no vestibule or sulcus can be seen between the cheek and tooth-bearing areas (Figure 5-4). The vestibule forms as a result of the proliferation of the vestibular lamina into the ectomesenchyme soon after formation of the dental lamina. The cells of the vestibular lamina rapidly enlarge and then degenerate to form a cleft that becomes the vestibule between the cheek and the tooth-bearing area.
Initiation of the Tooth
An intriguing question is how dental development is initiated. When murine (mouse) first arch epithelium is combined with caudal or cranial neural crest in the anterior chamber of the eye, teeth form (Figure 5-5). Epithelium from other sources, such as a limb bud or the second arch, does not elicit this response (Table 5-1). However, after day 12 of development, first arch epithelium loses this odontogenic potential, which then is assumed by the ectomesenchyme so that the ectomesenchyme can elicit tooth formation from a variety of epithelia. For example, recombination of late first arch ectomesenchyme with embryonic plantar (foot) epithelium changes the developmental direction of the epithelium so that an enamel organ is formed. Conversely, if the epithelial enamel organ is recombined with skin mesenchyme, the organ loses its dental characteristics and assumes those of epidermis. What these experiments indicate is that odontogenesis is initiated first by factors resident in the first arch epithelium influencing ectomesenchyme but that with time this potential is assumed by the ectomesenchyme. These experimental findings are mirrored by the expression pattern of transcription and growth factors in these tissues. Some of the genes involved in tooth formation are listed in Box 5-1 and are discussed in the following text (see Table 5-1).
TABLE 5-1
Outcome of Various Recombinations of Epithelium and Neural Crest
COMBINATION | TEETH | BONE | CARTILAGE | NEURAL CREST |
Neural crest and mandibular epithelium | + | + | + | + |
Neural crest and limb epithelium | – | + | + | + |
Neural crest alone | – | – | + | + |
Mandibular epithelium alone | – | – | – | – |
From Lumsden AGS. In Mederson PFA, editor: Development and evolutionary aspects of the neural crest, New York, 1987, John Wiley & Sons.
The earliest histologic indication of tooth development is at day 11 of gestation, which is marked by a thickening of the epithelium where tooth formation will occur on the oral surface of the first branchial arch. What are the signals mediating the initial steps in tooth development? (Figure 5-6.) To date, the earliest mesenchymal markers for tooth formation are the LIM-homeobox (Lhx) domain genes (transcription factors), Lhx-6 and Lhx-7. Both of these genes are expressed in the neural crest–derived ectomesenchyme of the oral portion of the first branchial arch as early as day 9 of gestation. Experimental data demonstrate that the expression of Lhx-6 and Lhx-7 results from a signaling molecule originating from the oral epithelium of the first branchial arch. If second arch mesenchyme is recombined with first branchial arch oral epithelium, Lhx-6 and Lhx-7 will be induced. However, if first branchial arch mesenchyme (which expresses Lhx-6 and Lhx-7) is recombined with second branchial arch epithelium, expression of both genes will be down-regulated quickly. A prime candidate for the induction of Lhx genes is secreted fibroblast growth factor-8 (Fgf-8); this growth factor is expressed at the proper place and time in the first branchial arch and is able to induce Lhx-6 and Lhx-7 expression in in vitro experiments.
This explains in rather simple terms the establishment of the oral-aboral axis. The next question in terms of developmental signals is what controls the position and the number of tooth germs along the oral surface? Again from the experimental data available, the signals for these aspects appear to originate from the oral epithelium. Fgf-8 already has been shown to play a role in the oral-aboral axis and seems to have a role in determining the positions where the tooth germs will form. The Pax-9 gene is one of the earliest mesenchymal genes that define the localization of the tooth germs. Pax-9 gene expression colocalizes with the exact sites where tooth germs appear. Pax-9 is induced by Fgf-8 and is repressed by bone morphogenetic proteins (BMP-2 and BMP-4). Fgf-8, Bmp-2, and Bmp-4 are expressed in nonoverlapping areas, with Pax-9 being expressed at sites where Fgf-8 but not Bmp is. Of course, a number of other genes are also expressed in oral epithelium at the same time. Whether they directly regulate the expression of Fgf-8 or Bmps is not clear at this time. Signaling molecules often regulate the expression of transcription factors that turn out to regulate the expression of those same signaling molecules. Little is known about the regulatory mechanisms of signaling molecules, and untangling the network of regulatory events can be difficult. At least 12 transcription factors are expressed in odontogenic mesenchyme, and some have redundant roles. To date, more than 90 genes have been identified from the oral epithelium, dental epithelium, and dental mesenchyme during the initiation of tooth development. The reader is directed to the Gene Expression in Tooth web page (http://bite-it.helsinki.fi) for a more up-to-date and complete list. The level of complexity becomes evident quickly in that generating a single knockout mutant often is not sufficient to determine the role played by specific genes, especially when they are members of a large family. For example, Dlx-1 and Dlx-2 show a tooth phenotype only in double knockout mutants, and not all the teeth are affected. This may be explained by the compensatory action of other Dlx genes (e.g., Dlx-5 and Dlx-6). Thus the evidence from experimental embryology, recombinant DNA technology, and immunocytochemistry indicates that first arch epithelium is essential for the initiation of tooth development.
In mice, expression of Shh is localized to the presumptive dental ectoderm at E11 and is thus another good signaling candidate for tooth initiation (Figure 5-7). Shh knockout mice have little development of the facial processes, and thus any role in tooth initiation cannot be identified from these. Mutations in Gli genes that are downstream mediators of Shh action suggest a role in early tooth development because Gli2-/- and Gli3-/- double mutant embryos do not produce any recognizable tooth buds. Addition of Shh-soaked beads to oral ectoderm can induce local epithelial cell proliferation to produce invaginations that are reminiscent of tooth buds. Shh thus appears to have a role in stimulating epithelial cell proliferation, and its local expression at the sites of tooth development implicates Shh signaling in tooth initiation.Cbfa1, also referred to as Osf2, is a transcription factor that plays a critical role during bone formation (see Chapter 6). Its expression in dental mesenchyme is associated with the early signaling cascades regulating tooth initiation. It regulates key epithelial-mesenchymal interactions that control advancing morphogenesis and histodifferentiation of the enamel organ. Lack of expression of Cbfa1 causes cleidocranial dysplasia syndrome characterized by bone defects and multiple supernumerary teeth.
Tooth Type Determination
The determination of specific tooth types at their correct positions in the jaws is referred to as patterning of the dentition. The determination of crown pattern is a remarkably consistent process. Although in some animals teeth are all the same shape (homodont), in most mammals they are different (heterodont), falling into three families: incisiform, caniniform, and molariform. Two hypothetical models have been proposed to explain how these different shapes are determined, and evidence exists to support both. The first is the field model, which proposes that the factors responsible for tooth shape reside within the ectomesenchyme in distinct graded and overlapping fields for each tooth family (Figure 5-8). The fact that each of the fields expresses differing combinations of patterning homeobox genes supports this theory. The clone model proposes that each tooth class is derived from a clone of ectomesenchymal cells programmed by epithelium to produce teeth of a given pattern (Figure 5-9). In support of this contention, isolated presumptive first molar tissues have been shown to continue development to form three molar teeth in their normal positional sequence. Possibly both models can be combined, for temporal factors may play a role. For instance, the coded pattern of homeobox gene expression in the ectomesenchyme might be expressed following an epithelial signal, as was the case for tooth initiation. Furthermore, as with tooth initiation, ectomesenchyme eventually assumes the dominant role in crown pattern formation. Recombination of molar papilla with incisor enamel organ results in molar development; conversely, recombination of incisor papilla with molar enamel organ results in incisor development (Figure 5-10).
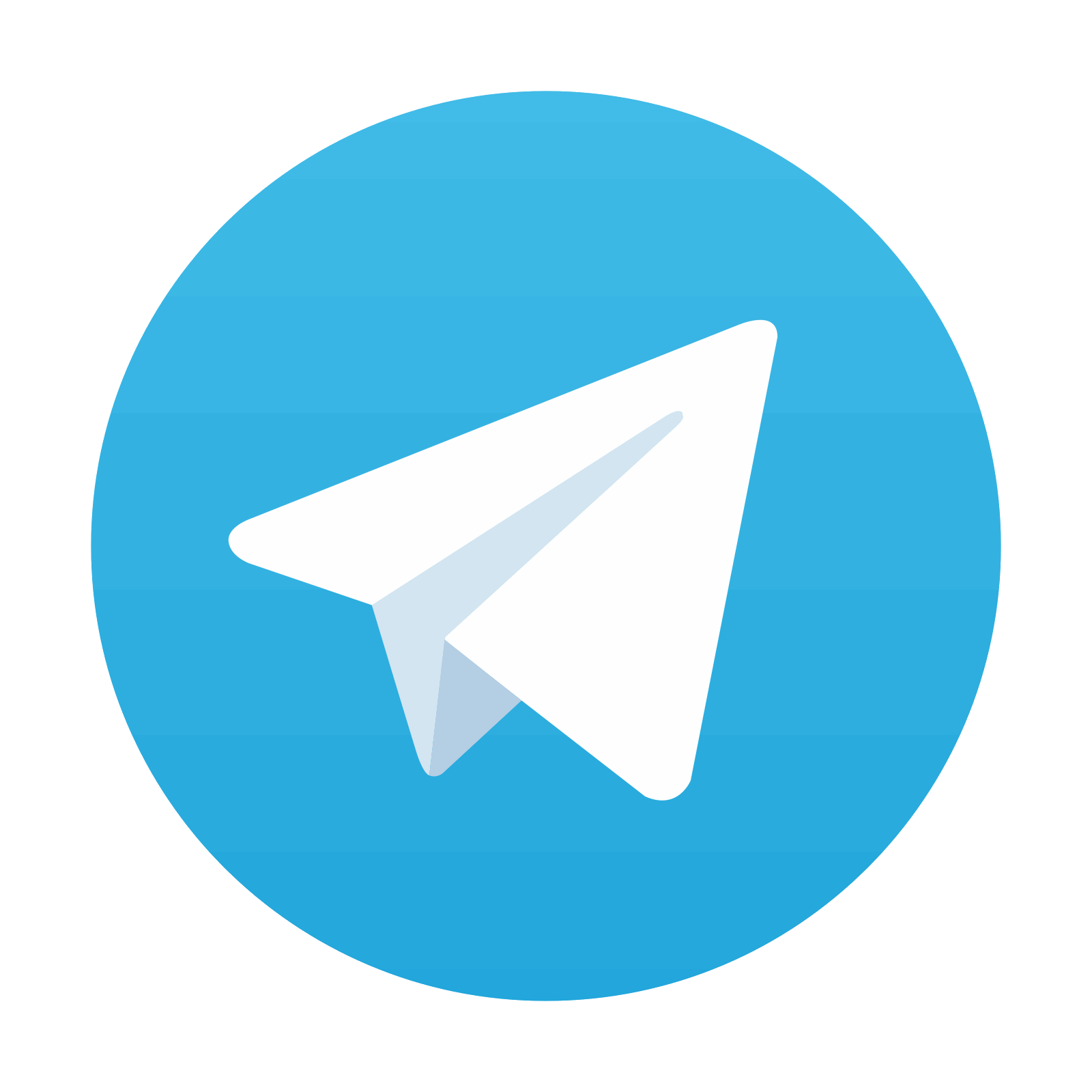
Stay updated, free dental videos. Join our Telegram channel

VIDEdental - Online dental courses
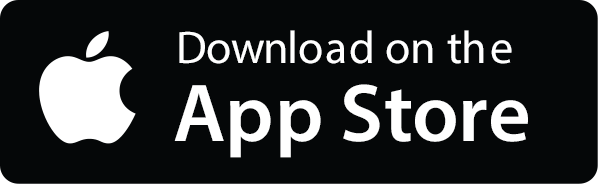
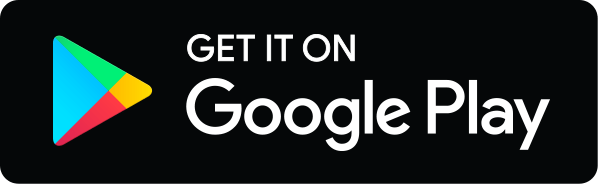