4
Orthodontic applications of alveolus decortication
Neal C. Murphy
Department of Orthodontics, Case Western Reserve University, School of Dental Medicine, Cleveland, OH, USA
Department of Periodontics, Case Western Reserve University, School of Dental Medicine, Cleveland, OH, USA
Introduction
The last half of the 20th century witnessed countless improvements in the orthodontic specialty, and those of the last 10–15 years have been particularly profound. Improvements of appliance aesthetics, self-ligating bracket systems, clear aligners, computerized imaging systems, implant-like anchorage screws, and many other developments have elevated the expectations of clinicians and patients alike. These recent advances offer convenience and esthetic advantages that make orthodontic therapy more acceptable, especially to adults. Despite all these innovative changes, the length of the orthodontic treatment still haunts the specialty as a major deterrent for many patients. So, in the last two decades, enterprising periodontists have resurrected and refined century-old methods of accelerating orthodontic therapy.
The procedures in general are referred to as “surgically facilitated orthodontic therapy” (SFOT). Originally, two basic procedures were performed, but these are now generally eschewed by periodontists because of their excessive morbidity. These traditional surgeries included crude segmental osteotomies luxated with chisels and mallets and rudimentary corticotomies that were undisciplined in their applications. Popularized largely by the intrepid studies of one clinical professor of periodontology,1 the rudimentary methods have been refined to discrete and minor outpatient procedures using selective alveolar decortication (SAD). Today, a growing consortium of visionary clinicians worldwide is refining and developing new incarnations (Figure 4.1) of SAD and continuing this global renaissance for a new generation of periodontists and orthodontic specialists.
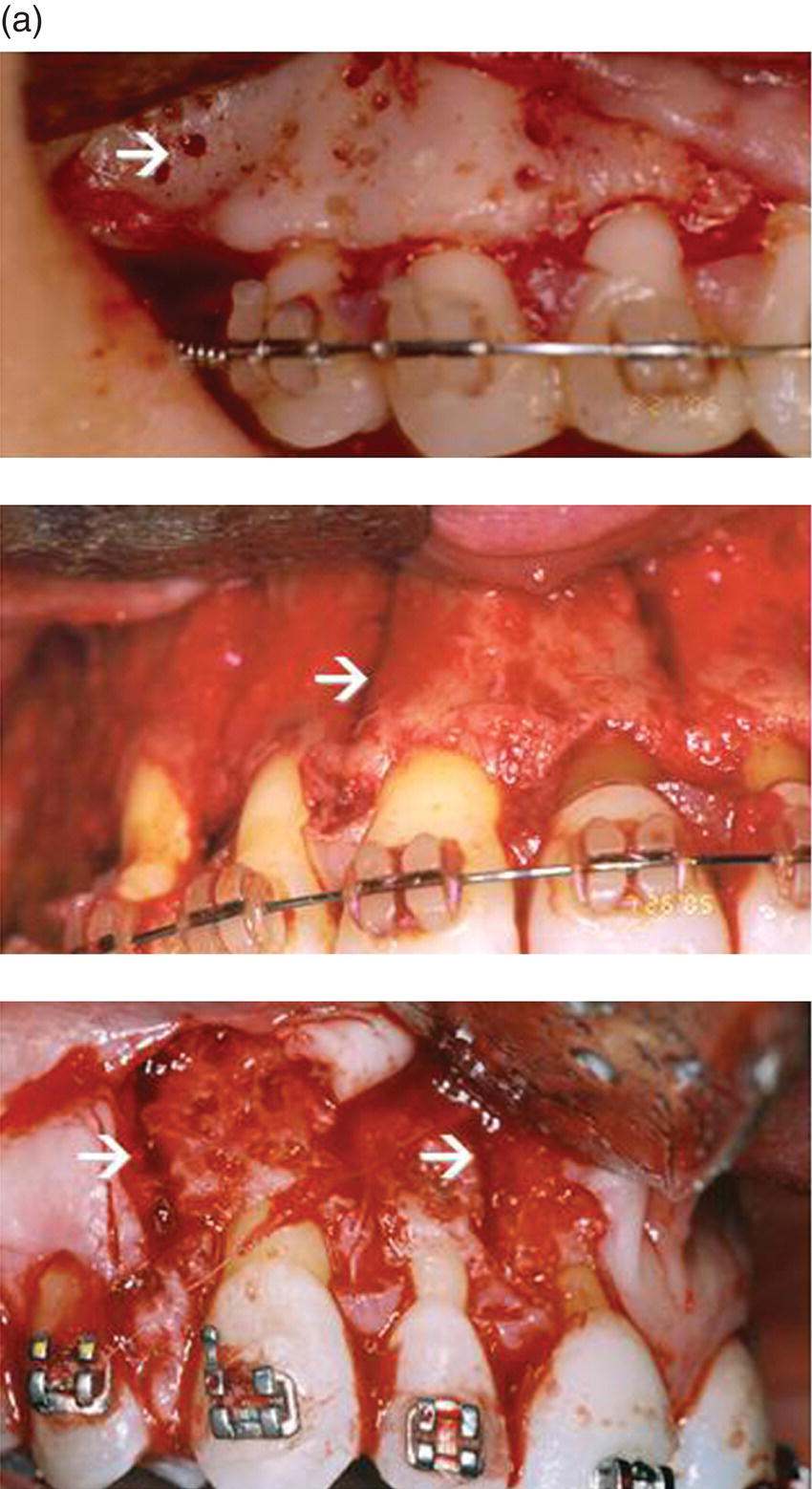
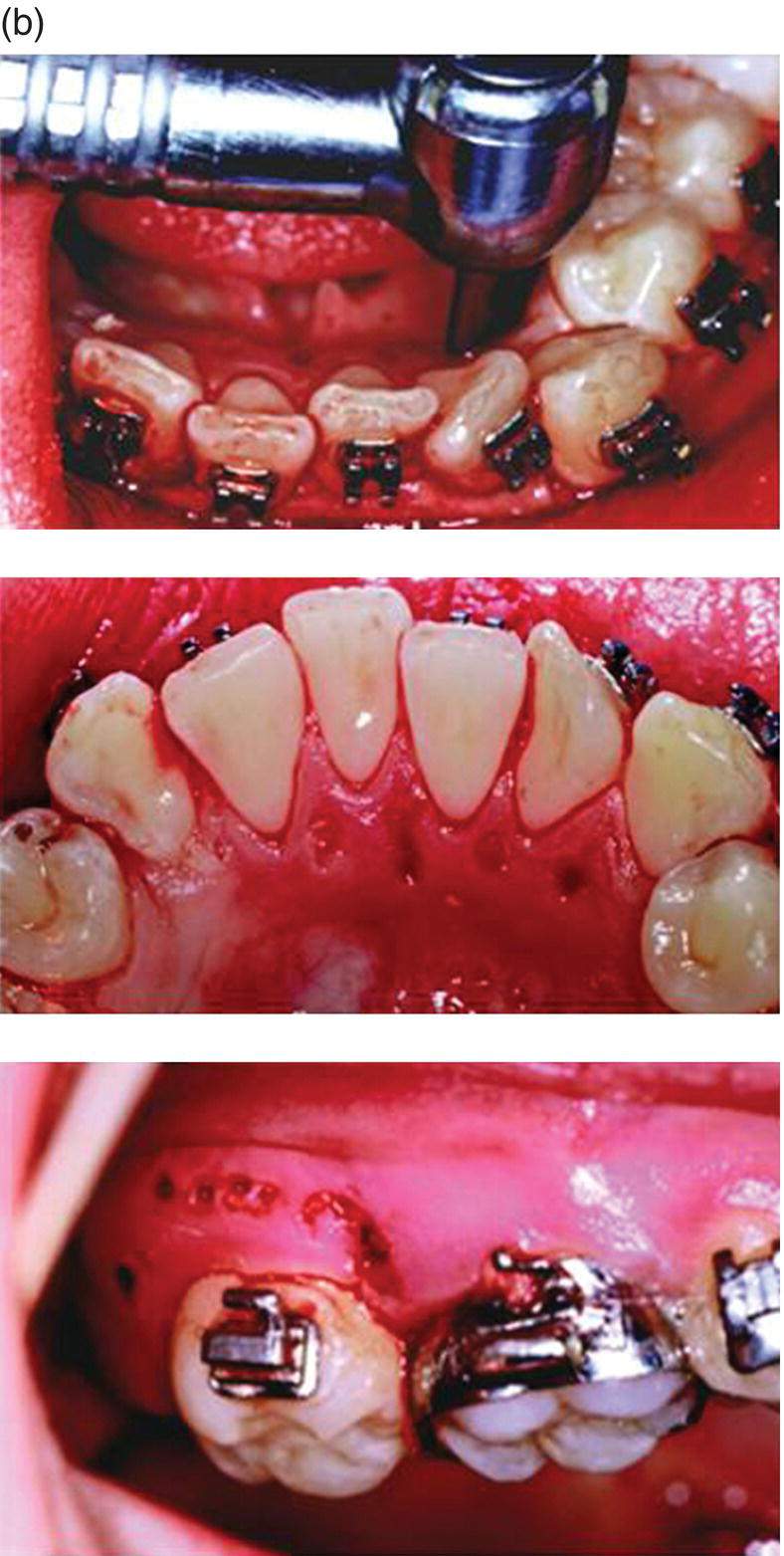
Figure 4.1 This demonstrates a series of selective alveolar decortication techniques to engineer accelerated tooth movement. The decortication quantitatively can be minimal, moderate, or aggressive. Qualitatively, they may be punctate or linear. Combinations vary by doctor preference and degree of osteopenia needed for a given amount of orthodontic tooth movement (OTM). (a) SAD induces a therapeutic, reversible regional osteopenia to facilitated accelerated OTM. The osteopenic state is extended by the strain of OTM or indefinitely by trans-mucosal perturbation (perforation) (TMP). The pattern of decortication is irrelevant, but the degree of decortication is commensurate with the degree of required therapeutic osteopenia. Here, we see three styles of decortication: top – a conservative punctate (arrow); middle – a moderate linear (arrow), and bottom – an aggressive combination (arrows). (b) TMP. This is an epigenetic perturbation of healing morphogenesis by perforation of the alveolus with a high-speed, surgical-length irrigated dental round bur. Top: TMP technique; middle: TMP to induce therapeutic transient osteopenia for mandibular incisor labial tipping; bottom: TMP to accelerate OTM of maxillary second molar.
(source: UniversityExperts.com used with permission.)
Most recently, even these manipulations have been enhanced with sophisticated grafting. Interestingly, these innovations – (Periodontally) Accelerated Osteogenic Orthodontics™ ((P)AOO™)2 and periodontal stem cell therapy (PSCT) (Figure 4.2) – have been enthusiastically received by patients. But they are attracted to them neither for scientific reasons nor the minimal morbidity they enjoy. Rather, patients prefer them because they reduce total treatment time.
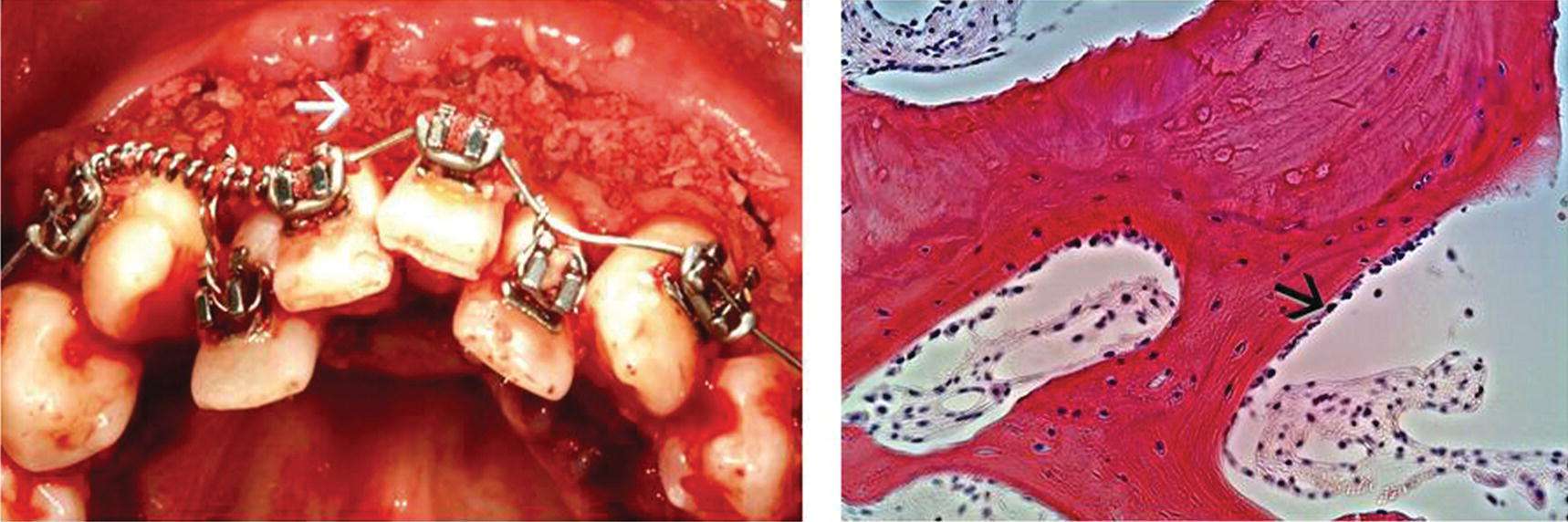
Figure 4.2 Refinements of SAD engineered to augment the mass of the alveolus bone. Left: PAOO is SAD with a demineralized bone matrix (DBM), so called “dead bone” at white arrow. DBM is also referred to as demineralized freeze-dried bone allograft (DFDBA). Right: H&E specimen of sample taken from alveolus after augmentation with PSCT, PAOO with a viable Mesenchimal Stem Cell (MSC) allograft, so-called “live bone,” at black arrow.
(source: UniversityExperts.com used with permission.)
However, the surprisingly high degree of patient acceptance of such tissue engineering – singularly reducing treatment times by 200–400% – should not eclipse other professional advantages. These include but are not limited to, less infection, fewer caries, and less gingival damage (Waldrop, 2008), or attachment loss (Zachrisson and Alnaes, 1974). Traditional side effects, such as root resorption and decalcifications (Levander and Malmgren, 1988; Geiger et al., 1992; Segal et al., 2004; Fox, 2005; Bishara and Ostby, 2008; Pandis et al., 2008) and compromised patient compliance (Royko et al., 1999), also may be a thing of the past when SAD and its regenerative variants are employed.
In our fast-paced world there have been many other attempts to shorten the orthodontic treatment time, such as rapid distraction of the canines (Liou and Huang, 1998), local application of prostaglandin (Spielmann et al., 1989), pulsed electromagnetic fields (Showkatbakhsh et al., 2010), mechanical vibration (Nishimura et al., 2008), low-intensity laser (Cruz et al., 2004; Yamaguchi et al., 2010), minor perforation (perturbation) of the adjacent alveolus bone (Murphy, 2006; Murphy et al., 2012), and the derivatives, corticision (Kim et al., 2009), Piezocision (Dibart et al., 2009), and piezopuncture (Kim et al., 2013).
However, these pale in comparison to the profound structural changes that are evoked by the salient incarnations of decortication-facilitated orthodontics (Bell and Levy, 1972; Anholm et al., 1986; Gantes et al., 1990; Suya, 1991; Wilcko et al., 2001, 2003), PAOO and PSCT in particular. In a very recent study, Long et al. (2013) conducted a systematic review of interventions for accelerating tooth movement. They compared low-level laser therapy, corticotomy, electrical current, pulsed electromagnetic fields, and dentoalveolar or periodontal distraction. They concluded that, among these five interventions, the decortication procedure was safest and clearly able to accelerate orthodontic tooth movement.
The basic concepts
SAD,3 as it has evolved into the 21st century, employs an intentional, but minor, superficial “injury” to the cortical bone. Its origin lies in less discrete procedures first described in the late 19th century as an expedient way of surgically treating malocclusion and found its way into the American literature in the mid 20th century (Köle, 1959). At the cell level, mechanical microfractures of the osteon will induce remodeling that adapts the bone to novel mechanical stimuli, and SAD is the macroscopic, clinic-level analogue. At the turn of the 21st century, Wilcko et al. (2001) added the alveolar augmentation (bone grafting) to the SAD protocol and termed the innovation PAOO (Wilcko et al., 2001, 2003). This technique, combining alveolar decortication with bone grafting with DFDBA or MSCs to expand alveolar volume, exploits both the SAD-induced opportunity for rapid tooth movement and the enlargement of alveolus bone volume to accommodate dental arch expansion.
To explain the startling results of this pioneering work the designers called upon established histophysiologic mechanisms of decalcification and recalcification of the alveolus bone that is commonly encountered after periodontal osseous surgery. This posited mechanism is supported by the medical orthopedic literature (Bogoch et al., 1993; Frost, 1989) wherever it is applied to SAD. Therefore, a decalcification–recalcification description of the alveolus bone response is a direct and legitimate extrapolation of histophysiology seen in long bone fractures. The concept was extended further into 21st century tissue engineering with the addition of MSCs (Murphy et al., 2012).
Since the validity of SAD procedures has been scientifically validated by independent replication and the scientific justification is sound, the next developmental challenge is determining how predictable and realistic goals may be achieved with this nascent science: oral tissue engineering. Thus, the aim of this chapter is to explain how various alveolar decortication procedures can play a significant role in orthodontic treatment.
Orthodontic implications of bone injury: the regional acceleratory phenomenon
When bone is traumatized in any way, a very specific and dynamic healing process occurs at the site of the bone “injury” that is proportional to the extent of the insult. This is best described by Frost in the orthopedic literature as a regional acceleratory phenomenon (RAP) (Shin and Norrdin, 1985; Frost, 1989a,b). The term “regional” refers to the demineralization of both the trauma site and histological reaction in the adjacent bone. The term “acceleratory” refers to an exaggerated or intensified metabolic response nearby that extends slightly into the marrow (spongiosa). This metabolic burst is a manifestation of normal remodeling that speeds up healing.
For SAD, the demineralization is later followed by subperiosteal appositional osteogenesis. There is a localized surge of coordinated osteoclastic and osteoblastic activity that, in the early phases, manifests a decrease in bone density with an increased bone turnover. The RAP begins within a few days of the surgery and becomes amenable to practical orthodontic tooth movement after 14 days. Then, depending on the degree of decortication, the demineralization peaks after 2–4 months – in the absence of orthodontically induced strain – and diminishes as remineralization sets in.
As alveolar decortication became popular, various animal experiments were conducted to explain its effects on the alveolar bone and orthodontic tooth movement potential. Sebaoun et al. (2008) evaluated the effects of decortication per se in the absence tooth movement in a split-mouth rat model. They found that at 3 weeks the catabolic activity (osteoclast count) and anabolic activity (apposition rate) were threefold greater in decortication sites. Calcified spongiosa decreased by twofold. Surgical injury to the alveolus that induced a significant increase in tissue turnover by week 3 dissipated to a steady state by postoperative week 11, and the impact of the injury was localized to the area immediately adjacent to the decortication. These data confirmed that alveolar decortication induced a RAP response in the alveolus like that in long bones, but Sebaoun et al. did not integrate the study with actual tooth movement.
Lee et al. (2008) investigated the effects of corticotomy-facilitated orthodontic therapy with micro-computed tomography (CT). Later, Baloul et al. (2011) compared the effects of alveolar decortication with and without tooth movement. In an award-winning4 experiment, the researchers studied three groups: decortication only, tooth movement only, and a “combined group” (tooth movement and decortication). They used morphologic analysis, quantitative micro-CT for structural analysis, and quantitative polymerase chain reaction to analyze messenger RNA gene expression associated with both osteoclasts and osteoblasts. Their data conclusively established that alveolar decortication enhances the rate of tooth movement during the initial tooth displacement phase with a “coupled” mechanism of bone cell activation adding to the natural histologic phenomena of orthodontic treatment.
The term “coupling” refers to the fact that resorption and apposition are not strictly sequential or independent events; they are simultaneous and functionally connected with each other, overlapping in their effects (King and Keeling, 1995; Proff and Romer, 2009), and vary by location, intensity, and duration.
Practical applications
These findings are directly applicable to any clinical setting, because they suggest accelerating the rate of tooth movement treatment and engineering RAP can impact orthodontic treatment planning in two major ways. First, manipulating the consequent osteopenia with SAD enhances the relative degree of orthodontic anchorage. Second, to increase the mass of the alveolus and expand the envelope of possible tooth movement, treatment necessitates a kind of concentrated or focused biomechanical protocol during an osteopenic ”window of opportunity.”
Enhancing relative anchorage
The practice of orthodontics is largely dependent on the availability of anchorage. Anchorage, by definition, is a body’s resistance to displacement. Newton’s third law suggests that for every force there is an equal and opposite reactive force. This explains differential rates of movement of two opposing objects which, practically speaking, is inversely related to the mass of the moved objects or resistance the force encounters. Thus, orthodontic treatments are designed with this law in mind, the goal being to resist unwanted tooth movement. According to Proffit (2000), in treatment planning,
…it is simply not possible to consider only the teeth whose movement is desired. Reciprocal effects throughout the dental arches must be carefully analyzed, evaluated, and controlled. An important aspect of treatment is maximizing the tooth movement that is desired, while minimizing undesirable side effects.
In orthodontic movement, segments of teeth that resist movement are often ligated together, with thin stainless steel wire or elastic chain (Figure 4.3), to serve as “anchors” and used to pull or push against other segments to be moved. Usually, the anchor segment will contain more teeth or teeth with greater “root enface”5 (Murphy et al., 1982) than the segment of teeth that are to be moved. This concept of differential anchorage is important in most orthodontic treatment, especially in cases of severe arch length deficiency (ALD); that is, “crowding” or skeletal dysplasias. In fact, treatment of certain malocclusions is often defined by the available anchorage; for example, “a maximum anchorage case.”
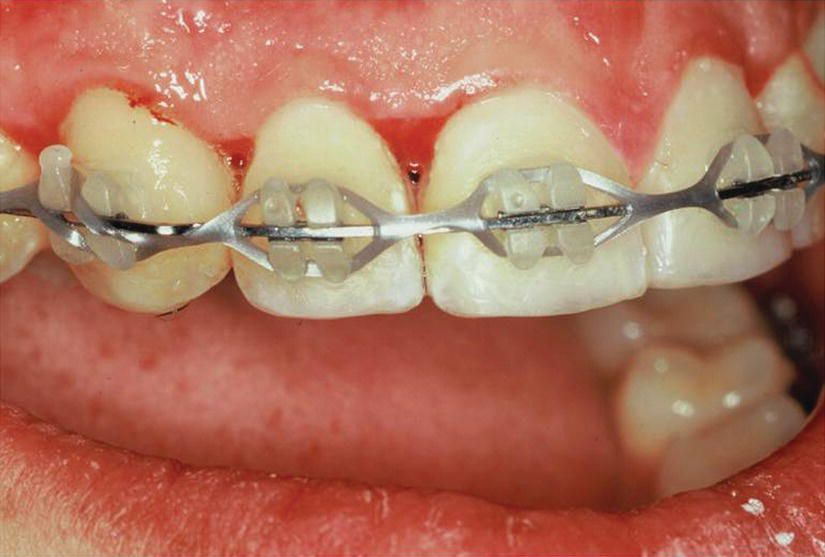
Figure 4.3 Orthodontic anchorage may consist of any combination of dental or tissue borne appliances. Usually, posterior teeth are ligated together for anchorage, but here, following SAD surgery, an elastic chain forms “anchor value” in the maxillary anterior sextant.
(source: UniversityExperts.com used with permission.)
There are numerous ways in which orthodontics has tried to augment anchorage, including auxiliary devices such as headgear, transpalatal arches, palatal acrylic pads (Nance appliances), and mini-implants of various designs. Many of these appliances are uncomfortable for patients or lead to “anchorage loss (slippage),” an untoward movement of dental anchorage units. Also, lower levels of patient compliance are common where extraoral traction or intraoral elastics interfere with patients’ psycho-social imperatives. Thus, treatment outcomes can become compromised.
When movement of anchor teeth is not important the term “minimal anchorage” is employed. For example, if an extraction space needs closing by the mesial movement of posterior teeth in addition to the posterior movement of anterior teeth, the term “minimal anchorage” is appropriate. Where very little posterior tooth movement is desired as anterior teeth are pulled against them, the term “maximum anchorage” is used. However, maximum anchorage is often more of a wish than a reality because even in this ideal scheme some posterior tooth movement may occur.
Recently, titanium screws used as temporary anchorage devices (TADs) have been shown to provide excellent absolute anchorage where no posterior anchor movement whatsoever can be demonstrated. Because titanium screws do not have a reactive periodontal ligament the titanium anchors are as perfectly stable as ankylosed teeth. So TADs have, over the last two decades, emerged as very acceptable anchorage adjuncts to orthodontic treatment. However, owing to a number of limitations, such as patient disdain and premature exfoliation, they may be as unreliable as extraoral traction.
Tissue resistance to tooth movement must also be considered in assessing anchorage potential by estimating volume and density of the alveolar bone in addition to the cross-sectional area of the roots perpendicular to tooth movement vector sum. This osseous tissue that must be resorbed for a tooth to move thus contributes greater or lesser “anchorage value” (Roberts, 2005). That is, where there is less bone and less dense bone in the trajectory, there is less anchorage value. So, rendering a given volume of bone less dense through SAD increases the relative resistance of the opposing biomechanical anchorage units. This is why SAD, PAOO, PSCT, or TMP should not be used within 4–6 mm of ligated molars or TADs.
In addition to these histological assets, the shrewd use of “relative anchorage” provided by SAD allows orthodontists to avoid notoriously unpredictable anchorage paraphernalia, such as intraoral elastics and extraoral traction (headgear) that rely on capricious adolescent compliance.
Maximizing the window of opportunity
Following the reactive demineralization after SAD, there is a 3–4 month clinical “window of opportunity” to move teeth rapidly through demineralized bone before it remineralizes. But this can be extended to 4–6 months in orthodontically strained bone or indefinitely with transmucosal perforation (TMP) of the bone with an irrigated high-speed round bur (Murphy, 2006; Murphy et al., 2012). Thus, the criticism (Mathews and Kokich, 2013) that RAP is too effete or transient for clinical use may be legitimately dismissed as spurious, naive, or premature.
If applying orthodontic force has the intention of lengthening the duration of the RAP effect, orthodontic activations must be made every 2 weeks after the decortication. The orthodontic force presumably delays the maturation of immature woven bone to mature lamellar bone around the decortication the way a mobile fracture fails to recalcify (malunion). This may occur by creating microfractures to the osteon and delays remineralization.
Since the effect of the decortication can diminish with time, the major tooth movements should be completed in the first few months of the treatment. So, the clinician should plan the mechanics to take advantage of this time frame. If possible, one should combine procedures using longer appointment times, if necessary to finish the major tooth movements. Caveat: undesirable delays caused by inefficient biomechanics, not an uncommon fate for unschooled neophytes, should not be unfairly attributed to SAD procedures per se. But fallacious critiques do indeed occur and, indeed, are inevitable with any clinical innovation.
A brief history of reduced treatment time
Early scientific literature discussing SAD contains mostly clinical case analyses. For example, after the seminal article by Köle (1959) Gantes et al. (1990) reported a safe but undeniable reduction of orthodontic treatment time by approximately 50%. Later, Wilcko et al. (2001, 2003) presented cases safely treated in approximately 6 months with PAOO. Then more sophisticated research studied the efficacy of the SAD and PAOO technique with master’s theses quality at St Louis and Boston universities under the astute and able tutelage of Professor DJ Ferguson. (See Chapter 1.)
Among these, Hajii (2000) studied decrowding of the mandibular anterior segment with conventional non-extraction and extraction treatment and then compared the outcomes with corticotomy-facilitated non-extraction therapies. This university-level research validated the hypothesis that PAOO significantly decreased the orthodontic treatment times in cases of mandibular Arch Length Deficiency (ALD). The average treatment times were 6.1 months for non-extraction PAOO in contrast to 18.7 months and 26.6 months for conventional non-extraction and extraction treatments respectively. Fulk (2001) reported similar results in mandibular crowding cases, demonstrating that average treatment times could be as short as 6.6 months for PAOO instead of 20.7 months for the conventional treatment group. Similar results were reported for maxillary ALD cases in replication studies by Skountrianos (2003).
Minimal effects
Fischer (2007) evaluated the effects of SAD (corticotomy) in patients with bilaterally impacted canines. In a split-mouth design, impacted canines were treated conventionally on one side and the other side had corticotomy-assisted exposure of the impacted canine. Fischer concluded that SAD could reduce treatment time by merely 30%, but a close analysis demonstrates major experimental design flaws that biased the results. So this has to be taken into account when analyzing Fischer’s data. Had the bone volume on each side been equal then the accelerated rate would have been much greater than 30%. Yet Fischer’s study shows that even when applied somewhat inappropriately, which may be the reality in private practice, SAD and PAOO can make a clinically significant contribution to the orthodontic treatment plan.
Another split-mouth design to evaluate the effects of corticotomy-facilitated orthodontics was done by Aboul-Ela et al. (2011), where canines were retracted following first premolar extractions in class II, division I cases. They found that during the first 2 months the average monthly rate of canine retraction was two times faster on the side subjected to decortication. These data suggest that PAOO outcomes can claim a treatment time almost universally faster than traditional methods.
It is important to note that the total “hands-on” treatment adjustment time – namely, time spent with the patient receiving specific personalized care (“chair time” or “doctor time”) – is the same as conventional therapy. However, with SAD derivatives the time intervals between adjustments are compressed to reduce total treatment time, the time from bonding to debonding. This means that SAD-facilitated orthodontic therapy has earned a legitimate place in any informed consent where patients are concerned about notorious orthodontic time-related side effects like root resorption and infection-induced damage (Zachrisson and Alnaes, 1974; Wennstrom et al., 1993; Waldrop, 2008).
Grafting with decortication
Increased alveolus mass and increased distances through which teeth are moved may be greater gifts from PAOO than the mere acceleration of tooth movement rate. The ultimate spatial limits of treatment prior to the introduction of the Wilcko research was dictated by perceptions that the “alveolar housing” of the dentition was immutable. This is not true. Alveolus bone by its very histological and ontological nature is malleable. However, this malleability, a kind of single-generation “phenotype plasticity,” occurs only under specific conditions in healthy tissue. The functional matrix hypothesis of Moss (1997a–d; Moss-Salentijn and Moss, 1997) tells us that the roots are the supporting framework or matrix of alveolar bone. That is, the osteogenic framework and “container” of bone mass dictates the functional development of alveolar phenotype.
When the alveolus periosteum is supported further from a given point by more tooth eruption, a longer root, or periosteum elevated by a bone graft, a potential space is realized. Since bone is a reactive tissue, then, analogous to water, bone tends to “fill the container” in which it is placed. So the bone graft, besides providing bone morphogenetic protein or viable allografts to induce osteogenesis, also serves as a volumetric scaffold within which endogenous stem cells can replicate and differentiate into functional osteoblasts. This is the developmental basis of the alveolus form that justifies safe tooth movement and augmentation of periodontal–alveolus mass.
The limits of orthodontic tooth movement are thus defined by the “walls” of the alveolus; namely, the periosteum and the periodontal ligament. Wherever these “walls” are built, within a reasonable phenotypic range, the bone will develop within them. So orthodontist are wise to define “available space” not by a projection of an aligned and uprighted dentition, but rather by the labial limits of the marginal gingiva and bony walls of the alveolus.
Without PAOO, orthodontic treatment of teeth beyond the limits of the labial or lingual alveolar plate can lead to dehiscence formation (McComb, 1994; Wennstrom, 1996; Zachrisson, 1996; Melsen and Allais, 2005; Joss-Vassalli et al., 2010) and predispose the patient to recession (Zachrisson, 1996; Melsen and Allais, 2005), especially where chronic infection inhibits adaptive fibroplasia (Aleo et al., 1974). In a case where there is already alveolar dehiscence formation before the orthodontic treatment, tooth movement may be contraindicated. But in some cases the alternative – premolar extraction – can lead to a flattened profile, premature aging, arrested development and a generally unaesthetic, “dished-in” appearance to the lower face. PAOO and PSCT solve this dilemma by grafting the defective areas at the beginning of orthodontic treatment to help prevent further destruction and allow movement through a greater distance.
This saving grace was demonstrated by Ahn et al. (2012) and Kim et al. (2011) independently. They reported the use of alveolar decortication and bone augmentation in decompensation of the mandibular teeth in class III patients. Both reports concluded that alveolar decortication with bone augmentation reduced complications such as gingival recession, alveolar bone dehiscence, and bone loss in the mandibular anterior region in the treatment of class III patients undergoing orthognathic surgery.
Since bone grafting increases both the alveolar volume and the dimensions through which teeth can be moved, it follows that more severely crowded cases can now be treated without tooth extraction. Indeed, Ferguson et al. (2006) reported that the limits of orthodontic tooth movement, posited by Sarver />
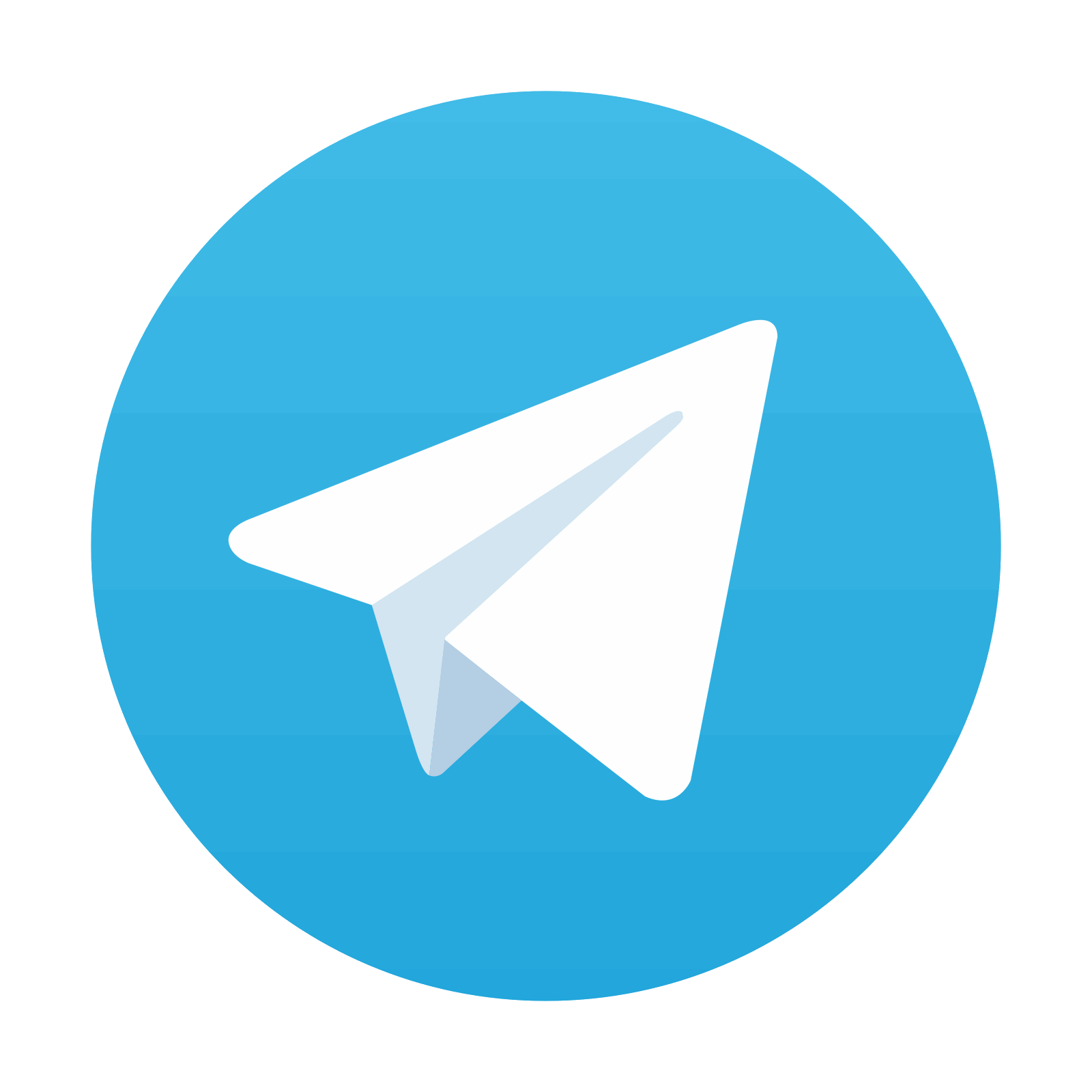
Stay updated, free dental videos. Join our Telegram channel

VIDEdental - Online dental courses
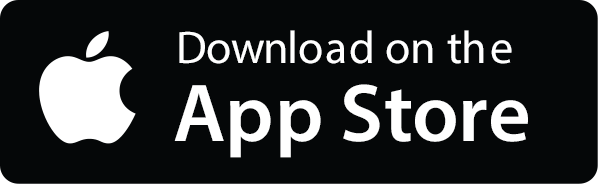
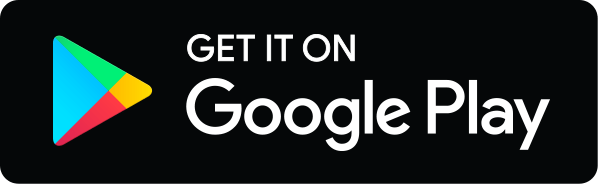