9
Treatment efficiency with the use of skeletal anchorage and corticotomy
Birte Melsen1 and Cesare Luzi1,2,3
1 Department of Orthodontics, School of Dentistry, Aarhus University, Aarhus, Denmark
2 University of Ferrara, Ferrara, Italy
3 Private Practice Limited to Orthodontics, Rome, Italy
Skeletal anchorage systems
Skeletal anchorage was introduced into orthodontics when the limits of conventional orthodontic anchorage were encountered. These limits were evident when the number of teeth available was insufficient for the establishment of a reactive unit that could serve as anchorage, or when all teeth had to be displaced in the same direction and no equilibrium could be obtained. It is often when faced with a desperate situation that humans becomes inventive. The first study of the reaction to metal inserted into bone was done by Humphry (1878), who inserted metal ligatures in the mandibular ramus in order to study the modeling of the growing mandible. With the same purpose, metal indicators were used by Bjork and Skieller (1972) to differentiate between displacement and modeling of the individual bones of the craniofacial skeleton. Already, Gainsforth and Higley (1945) had showed an interest in the tissue reaction to loading of metal inserted into bone. They applied forces to vitallium screws immediately after insertion into the mandible. The failure rate was 100%, and almost 25 years passed before Linkow (1970) suggested using metal blade implants for prosthodontics as anchorage. This approach did, however, have a limited indication and did not catch much attention. Sherman (1978) caught up on these results and recommended that a healing period would lead to higher stability. The interest in tissue reaction around loaded metal devices was most likely a spinoff from the development of dental implants (Albrektsson et al., 1986), and several researchers (Steinberg, 1978; Roberts et al., 1984) performed histological studies of the bone surrounding implants inserted in femora of rabbits.
The development of the dental implants had a direct impact on orthodontics, and the first application of intraoral extra-dental anchorage that allowed for an otherwise impossible treatment was done by Roberts et al. (1989), who, following loss of a first lower molar, inserted a small dental implant in the retromolar region and used it as anchorage for the mesial displacement of the second and third molars without adverse force systems to the anterior teeth that were in a desirable position (Roberts et al., 1990). This application of skeletal anchorage was widening the spectrum of orthodontics as such a displacement could not be done with conventional appliances. During the same period, Odman and co-workers pointed out how dental implants could be used as anchorage (Odman et al., 1988; Thilander et al., 2001). The limitation, however, is that the situation must be such that the dental implant is needed and can be placed before the orthodontic treatment is initiated.
The first case report introducing skeletal anchorage that did not originate from the dental implants described how a surgical wire inserted through a hole in the infrazygomatic crest could serve as anchorage for retraction of upper front teeth in patients with insufficient teeth for posterior anchorage (Melsen et al., 1998). This method was used in 15 patients over the years, but soon the need for skeletal anchorage that could serve as a reactive unit for other types of tooth movements became obvious. Meanwhile, a case report published by Creekmore and Eklund (1983) demonstrated how a surgical screw could serve as anchorage for intrusion and proclination of the upper front teeth. When published, this paper attracted little interest; but many years later, when special orthodontic anchorage screws had been described by Kanomi (1997) and screws replacing the zygoma ligatures were used by Costa et al. (1998), Creekmore and Eklund’s paper became a key reference.
Since the turn of the century, numerous skeletal anchorage systems have been introduced. The skeletal anchorage systems now referred to as temporary anchorage devices (TADs) came from two different sources. One category originated from the dental implants, thus relying on osseointegration, with a specially treated surface comprising conventional implants. Onplants (Block and Hoffman, 1995) and specially produced small dental implants (Wehrbein and Merz, 1998) used as palatal anchorage or, as described above, as retromolar implants (Roberts et al., 1990) are examples of this category. The other group comprised screws originating from the surgical world and included plates fixed with two or more screws (Umemori et al., 1999; Sugawara, 2005). Some of the screws require predrilling correspond to the full length of the TAD, whereas others are self-drilling and can be inserted directly. The morphology of the head of the TAD varies and may accordingly allow for one-, two-, or three-dimensional control and does, as such, have a significant impact on the spectrum of usage of these units (Melsen, 2005).
The indication for the usage of skeletal anchorage has widened, and a multiplicity of different TADs have been introduced. The application of TADs has made it possible both to perform treatments otherwise considered impossible and to facilitate the outcome of conventional treatments, as the need for compliance could be often reduced. TADs make it feasible not only to avoid adverse effects to the reactive units, but also reduce treatment time, as it is possible to apply goal-oriented force systems displacing teeth more directly from the original to the planned position, thereby reaching the treatment goal in less time and without useless tooth displacement.
Increasing the rate of tooth movement and shortening treatment time
Increasing the rate of treatment had already been attempted by Yamasaki et al. (1984), who injected prostaglandin into the periodontal ligament, enhancing initial tooth displacement. The principle was abandoned as it appeared that the anabolic effect was not following the katabolic effect boosted by the prostaglandin. Electrical stimuli were suggested by Davidovitch et al. (1980). This attracted considerable interest for a period, but was later abandoned, possibly due to the complexity of the appliances. Lately, vibration has been introduced, and shockwaves generated are shown to speed up the rate of tooth movement (the brand name AcceleDent® reflects the goal of the appliance delivering the shockwave) (Nishimura et al., 2008). Photobiomodulation (infrared light therapy) has also been suggested (Youssef et al., 2008; Yoshida et al., 2009; Yamaguchi et al., 2010), but has not yet caught the general market. However, the first and foremost approach related to enhance the rate of tooth movement has been corticotomy, which was first presented in the German world by Byloff-Clar (1967a,1967b). However, the technique did not gain very much popularity, and almost 10 years passed before the first publication with the keyword corticotomy appeared in English (Lines, 1975). This paper described corticotomy as part of a rapid palatal expansion, and the subsequent papers focused on the combination of maxillofacial surgery and corticotomy. The influence on tooth movement was not brought to the attention of orthodontists until the principle was presented by the Wilcko brothers as “Wilckodontics” (Wilcko et al., 2009). The corticotomy aims at the generation of a regional acceleratory phenomenon. When this occurs, the turnover is locally accelerated, which is then reflected in an increased rate of tooth movement (Iino et al., 2007; Iglesias-Linares et al., 2011). The “surgery first” approach recently introduced by Sugawara et al. (2010) is built on the same principle.
The corticotomy suggested by the Wilcko brothers included a full-thickness flap in addition to cutting and decortication. As a result, the bone goes through a phase of temporary osteopenia which facilitates the accelerated osteogenic osteotomy. However, recent studies performed on rats demonstrate that a similar acceleration of the molar movement can be obtained by performing three shallow holes 5 mm mesial to a rat molar prior to loading (Teixeira et al., 2010).
Treatment time, on the other hand, is not only influenced by the rate of tooth movements, but also, as mentioned above, by the distance the teeth have to be displaced. When, as usually advocated, a treatment is started with a leveling with a round wire, a lot of adverse effects and undesirable displacements take place as the force system generated will be dictated not by the treatment goal, but by the inter-brackets configuration, as described by Burstone and Koenig (1974) (Figure 9.1). If the treatment is guided by goal-oriented tooth movement, minimizing the total displacement of the individual teeth, treatment time can be reduced. This implies that the treatment has to be divided into phases, each of which has a specific treatment goal. A database from the office of one of the authors (BM) demonstrates that, in that office, where the treatments are not dependent on growth (adult patients) the median treatment time was 15.6 months. This can be considered a short treatment time compared with the reported average treatment time of close to 2 years or more. A probable explanation may be that the individual tooth was displaced as closely as possible along a straight line to the treatment goal. The initial leveling was avoided, meaning not only less jiggling, but also reduced risk of root resorption (Chan and Darendeliler, 2004). When the treatment time in relation to treatment with Suresmile® was examined in a very large study and compared with the common treatment time, Sachdeva et al. (2012) found a reduction of 8 months from the 23 months reported by the control clinics to 15 months in the practices working with Suresmile® designed appliances. This difference can, as the previous survey, be well explained by the tooth movements being more goal oriented than with the plain straight-wire technique, where the displacements are determined by the geometries, the mutual relation of the brackets, which in reality means that there is no way the displacement of the individual tooth can be predicted as the degree of freedom would be 6 (three directions and three moments) to the power of 12 when inserted into 12 brackets.
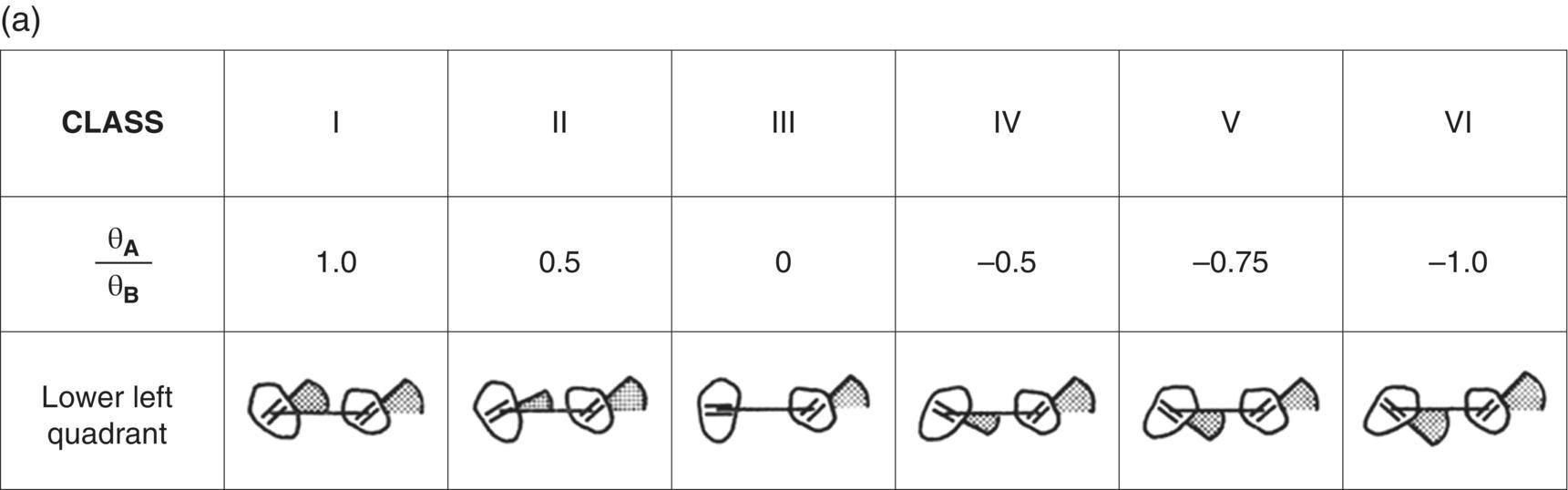
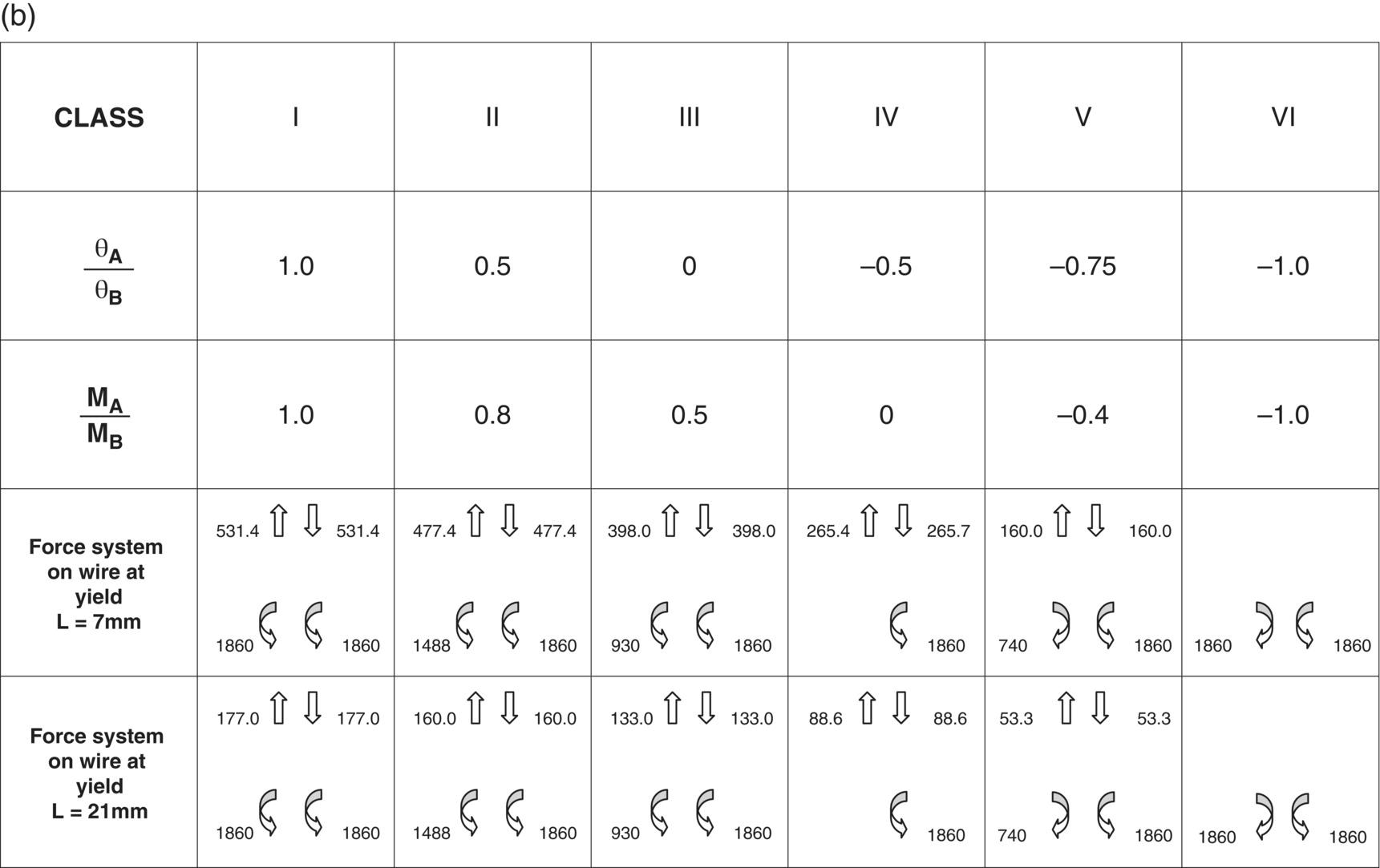
Figure 9.1 (a) Classification of the relationship between two teeth or units of teeth. The six basic geometries are based on the ratio between the angle of the bracket slots to a line connecting the midpoints of the brackets. The geometry is independent on the inter-bracket distance.
Source: Burstone and Koenig (1974). Reproduced with permission of Elsevier and Dr Charles J. Burstone.
(b) Force systems developed with respect to the bracket in the different geometries when a 0.016″ stainless steel wire is applied. Only the initial force is described; the effect within the bracket is disregarded.
Source: Burstone and Koenig (1974). Reproduced with permission of Elsevier and Dr Charles J Burstone.
A different approach to the shortening of the treatment time would be to take advantage of the fact that trabecular bone has a higher turnover than the cortical bone. This fact was used by Ricketts when referring to “cortical anchorage” and recommending the orthodontist to move the roots against cortical bone and secondarily use these teeth as anchorage (Diedrich, 1993). Displacement of the roots first in relation to space closure is, on the other hand, based on the wish to move the roots forward within the trabecular bone, which is generally surrounding the apical part of the roots (Figure 9.2). Thereby, a gradual modeling of the cortex is initiated, paving the way for the subsequent displacement of the tooth into even a narrowed or atrophic alveolar process or into an area with a maxillary sinus extension (Figure 9.3). This has sometimes been considered a limitation, but with a line of action of the force apical to the center of resistance of the tooth it can be done (Figure 9.4). With the control of the force system, even a significantly atrophied alveolar process or even a nonexisting alveolar process can be rebuilt (Figures 9.5 and 9.6). Moving the crowns first by means of tipping occurring during leveling will frequently lead to an adverse displacement of the apices in the opposite direction, leading to a need for an even larger movement of the root than at the start of treatment. This is especially relevant when vertical displacements are part of the leveling. The tooth to be extruded will simultaneously be subject to buccal root torque. On the contrary, the intrusion will lead to lingual root torque (Figure 9.7). The displacement of the roots through cortical bone can be facilitated by corticotomy, especially when space closure involves displacement of teeth through heavily atrophied alveolar processes. Corticotomy was introduced to surgeons in order to facilitate maxillary surgery and to orthodontists with the purpose of increasing the rate of tooth movement. However, new indications have emerged whereby the spectrum of orthodontics once more has been widened. The application of corticotomy combined with bone grafting has made it possible to move lower incisors buccally without iatrogenic damage to the cortical bone (De Clerck and Cornelis, 2006). The bone grafting combined with corticotomy has been recommended in patients with need for labial displacement of retroclined incisors, and the authors have demonstrated both increased rate of tooth movement and improved labial bone quality and quantity (Kim et al., 2011; Shoreibah et al., 2012). Especially the forward tipping of incisors has been warned against, but Allais and Melsen (2003; Melsen and Allais, 2005) demonstrated that by controlled tooth movement an overjet could be reduced without iatrogenic damage, and a 20-year follow-up revealed that the results could be maintained (Figure 9.8) (Lindtoft, 2010). There is, however, no doubt that the combination of well-controlled force systems, corticotomy, and bone grafting will open the door to a different approach to the treatment of increased overjet, especially in adult individuals with lower alveolar retrusion. There are thus a number of factors that can contribute to the widening of the orthodontic possibilities, and this chapter is dedicated to the demonstration of how the application of skeletal anchorage can contribute to an improved service for our patients. It is not enough just to have access to absolute anchorage; the mechanics should deliver a line of action of the force leading the tooth the shortest distance to the goal with a minimum variation in force direction and a low load–deflection rate. Well-controlled tooth movement can be used to build up bone, whether by displacing a molar through the maxillary sinus or an atrophic alveolar ridge. This was done by Fontenelle (1982) with a force system that allowed for the displacement of a molar mesially “with bone.”
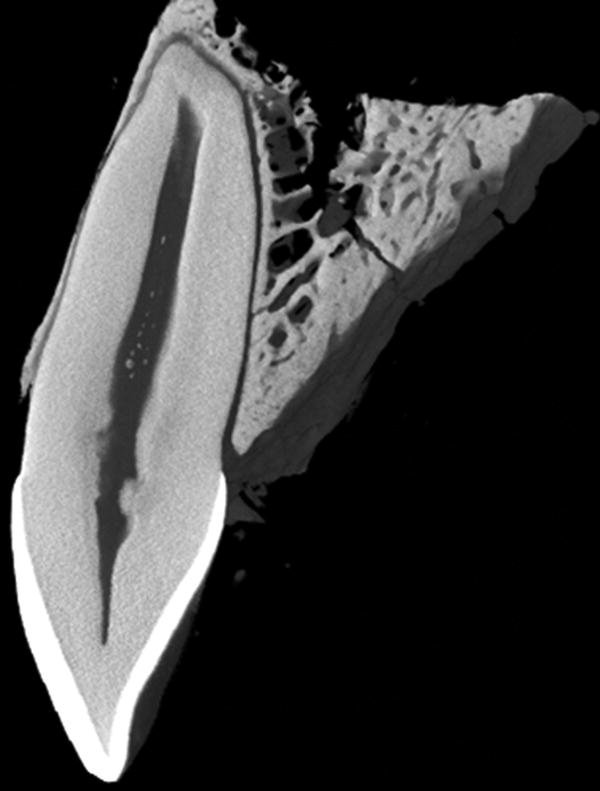
Figure 9.2 Sagittal cut through a micro-computed tomography reconstruction of a lateral incisor from a 30-year-old. Note the extremely thin cortex of the buccal alveolus with a fenestration at the mid-root level.
Adapted from: Morten G Laursen, MSc thesis, Aarhus University, 2007, with permission from Dr Michel Dalstra.
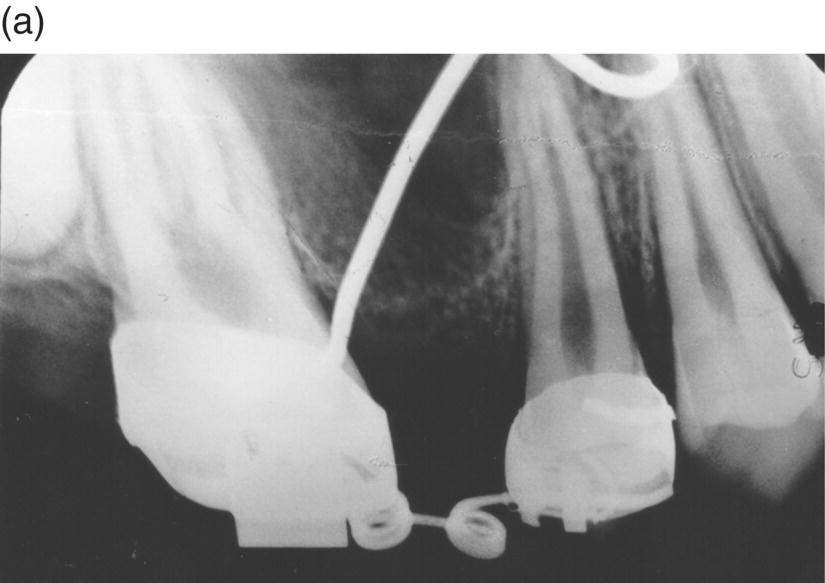
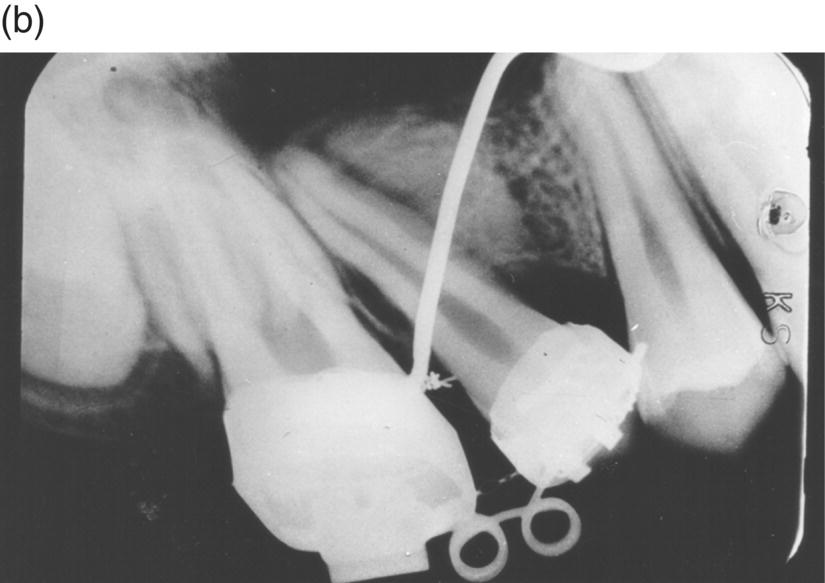
Figure 9.3 Periapical radiographs of a space closure bringing the premolar root distally against the maxillary sinus: (a) at the start of treatment; (b) 2 months later. As the uprighting of the premolar root took place it can be observed how the new bone is generated displacing the maxillary sinus.
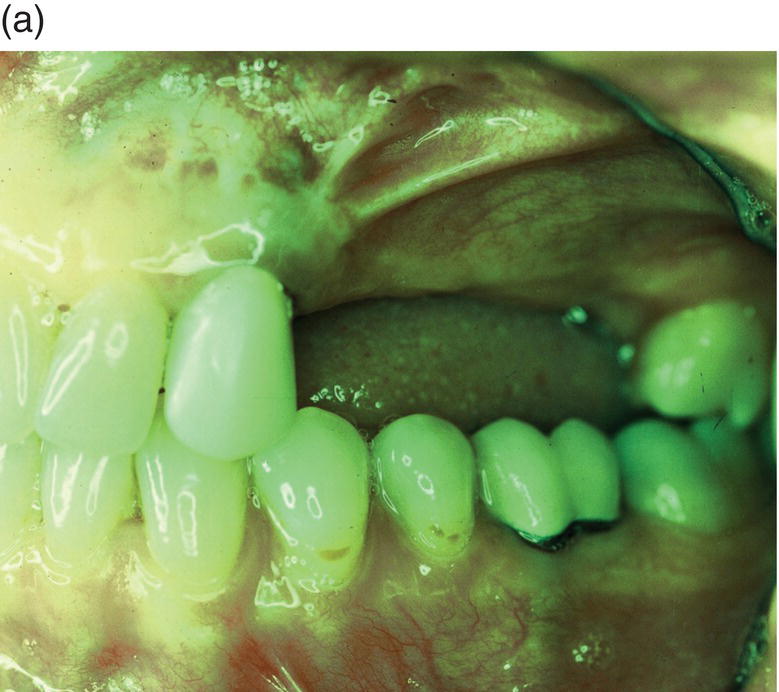
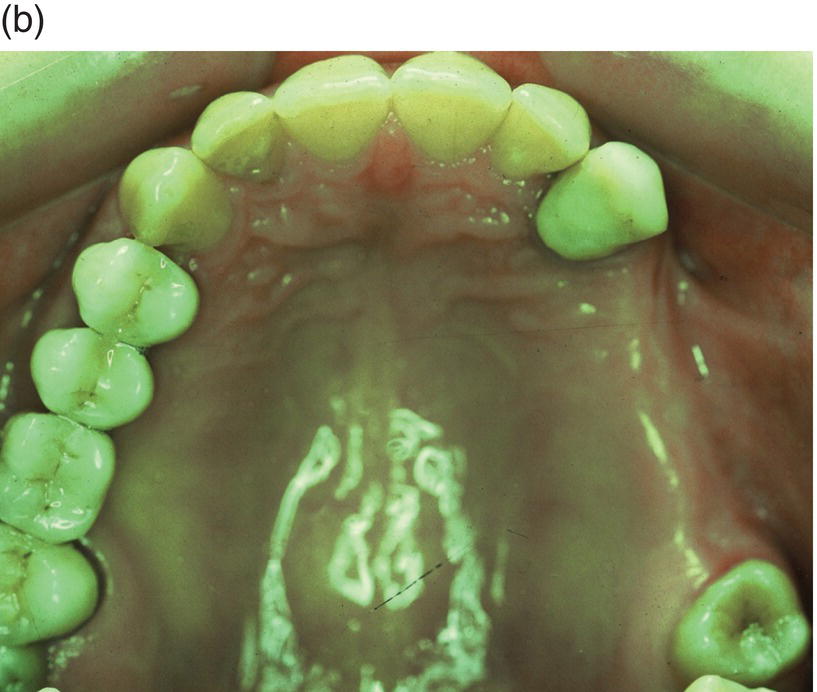
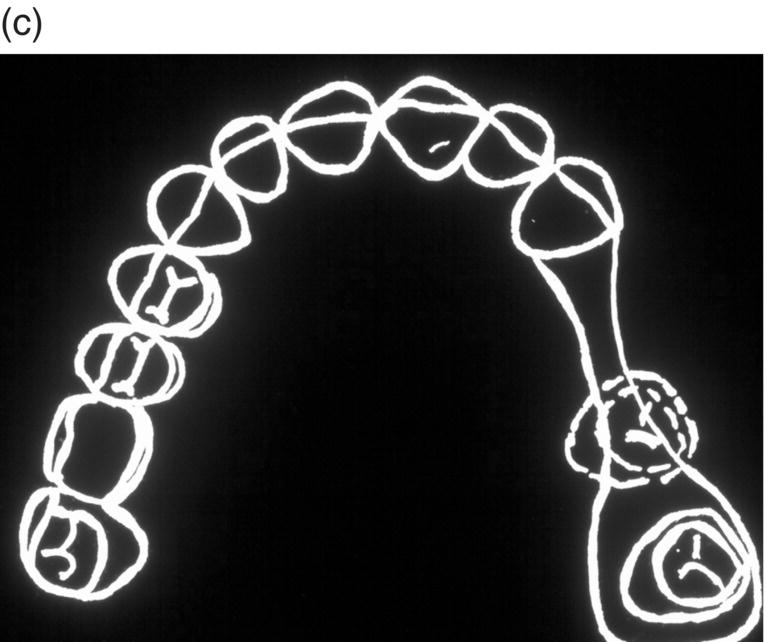
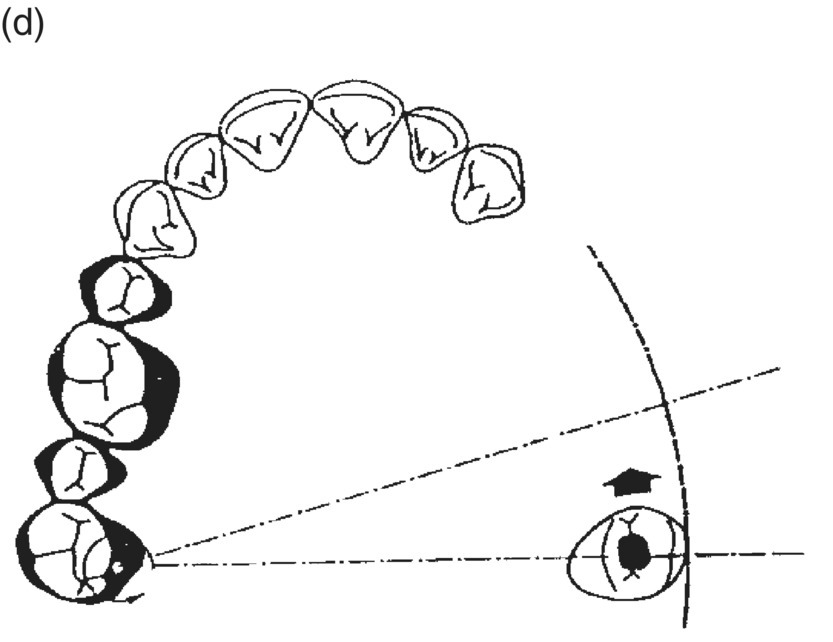
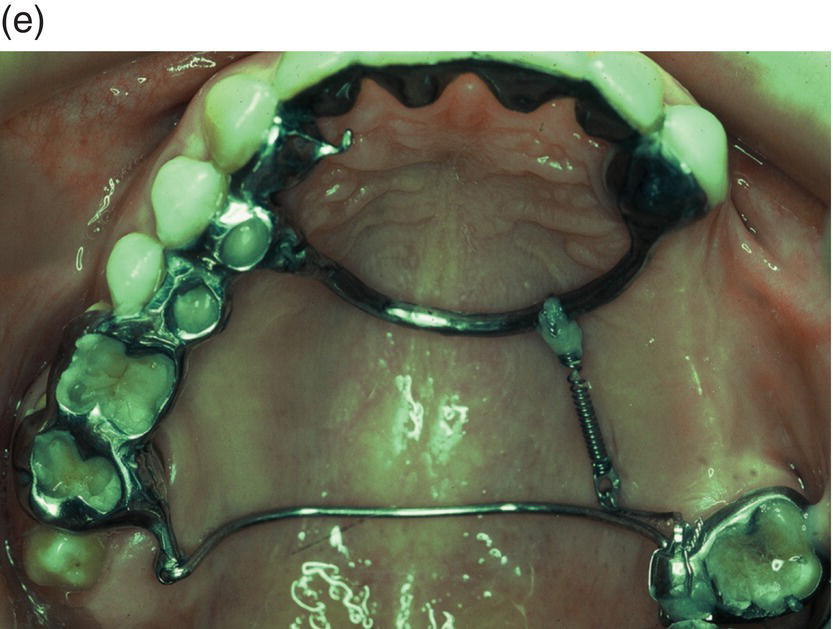
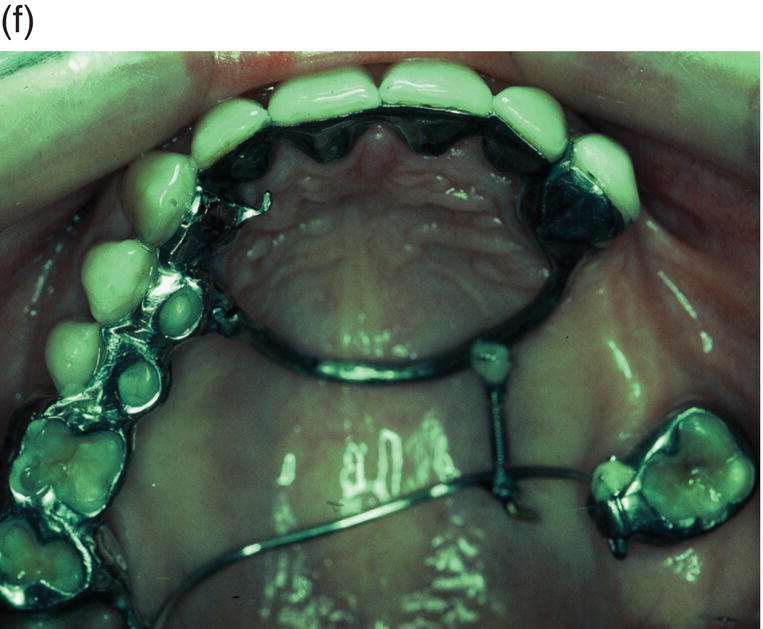
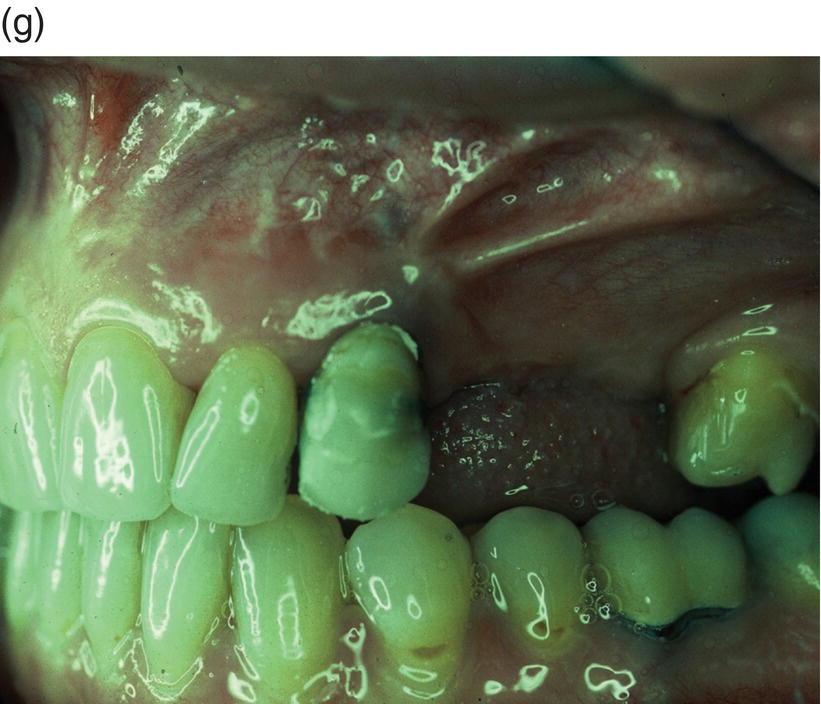
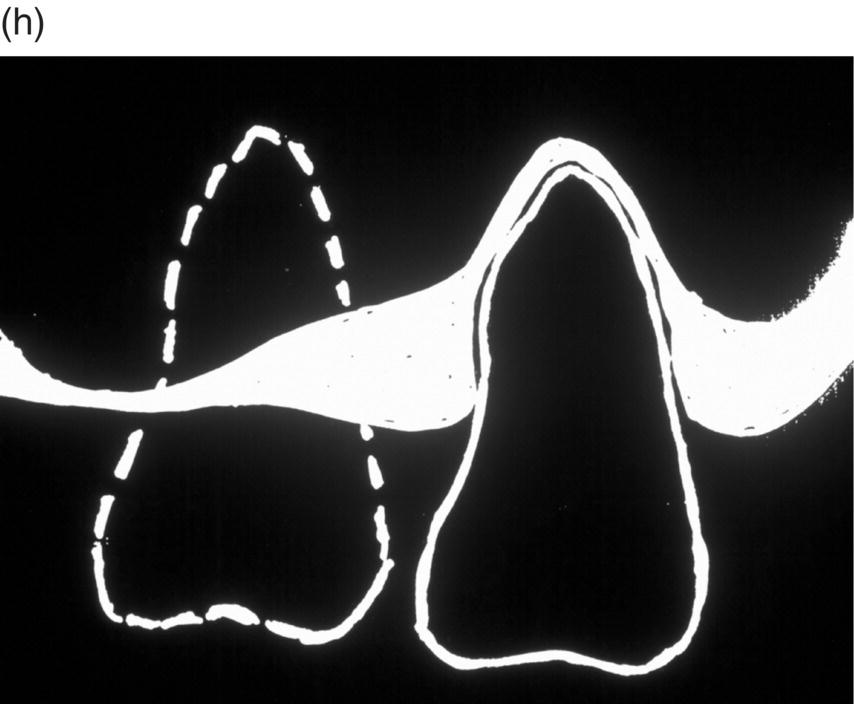
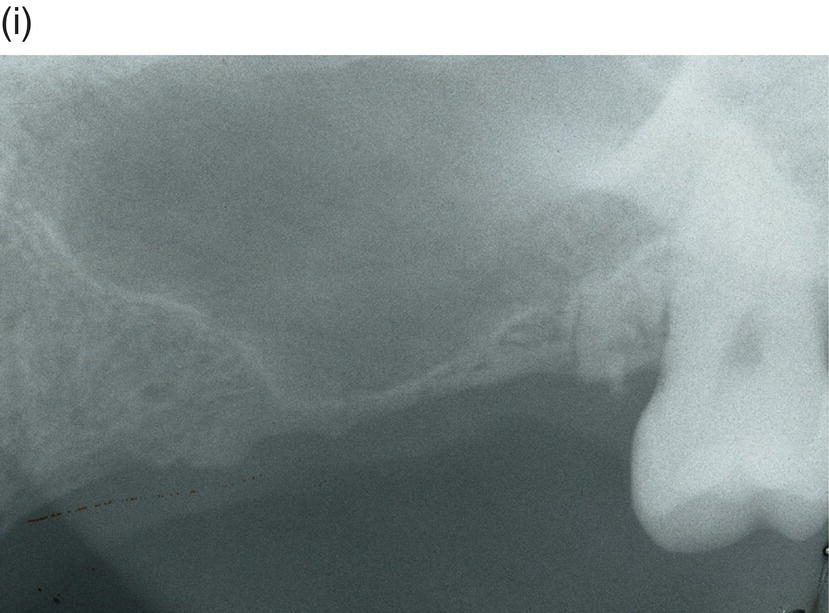
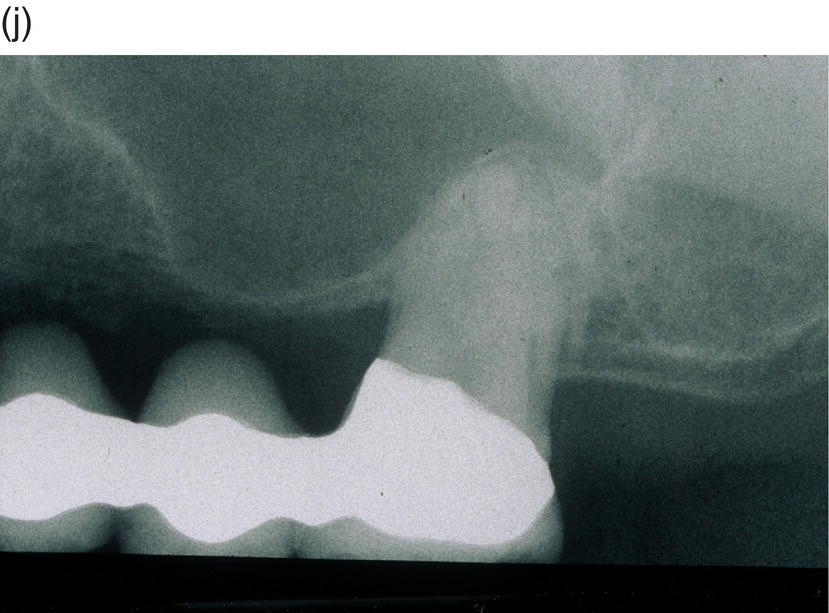
Figure 9.4 Hinge mechanics. (a,b) Patient who had lost all teeth between 23 and 28. The span was too long for a replacement with a bridge and the solution with more implants was not recommendable either, because of atrophy of alveolar bone and extension of the maxillary sinus leaving a paper-thin bone in the area. (c) Drawing illustrating the principle of hinge mechanics. (d) The anchorage unit was composed of all teeth apart from the third molar to be moved. These teeth were consolidated by a cast splint. The cast retention also comprised a transpalatal bar extending from nos. 14 to 24 and an extension palatally from no. 16. This extension was furnished with a vertical tube that served as the centre of rotation for the transpalatal arch serving as guiding mechanics for the mesialization of no. 28. The active unit was a coil spring at the level of the center of resistance from the anterior to the posterior tran/>
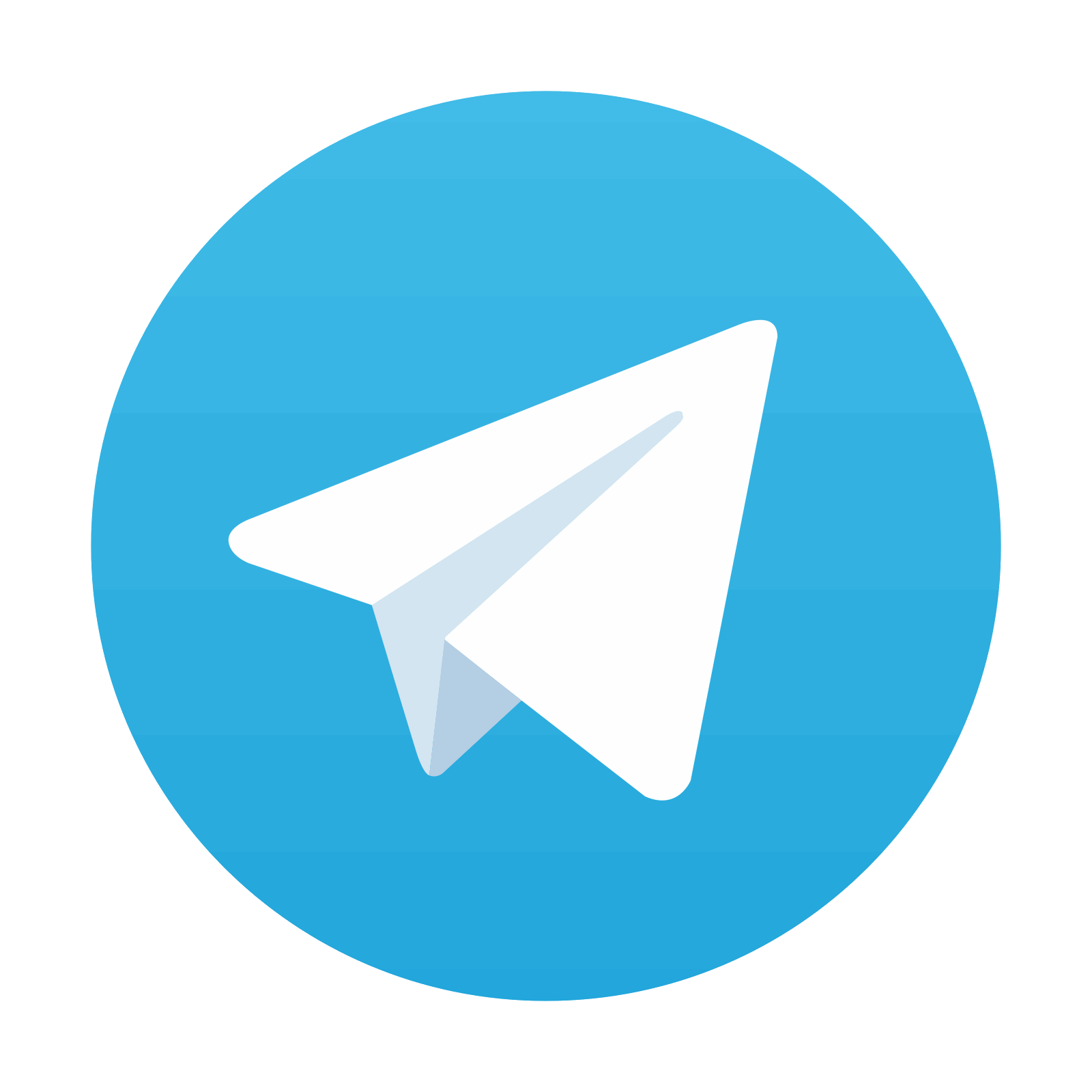
Stay updated, free dental videos. Join our Telegram channel

VIDEdental - Online dental courses
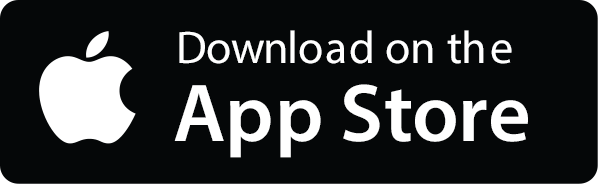
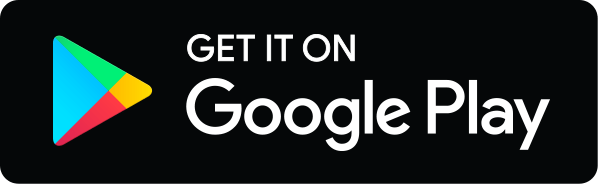