4
MTA in Vital Pulp Therapy
Till Dammaschke,1 Joe H. Camp,2,3 and George Bogen3
1 Department of Operative Dentistry, Westphalian Wilhelms-University, Germany
2 School of Dentistry, University of North Carolina, USA
3 Private Practice, USA
Natural forces within us are the true healers of disease.
—Hippocrates
INTRODUCTION
Vital pulp therapy is a procedure designed to preserve the vitality of the dental pulp. Treatment selection is dependent on the extent of remaining healthy pulp tissue, and includes direct pulp capping and partial or complete pulpotomy. Exposure of the pulp can occur due to caries excavation, trauma, restorative procedures, or anatomical anomalies. The cellular mechanisms involved in pulp repair and bridge formation will be reviewed in order to describe the principles and strategy supporting vital pulp therapy.
When microorganisms challenge the dental pulp, the tissue has an innate capacity for protection and repair (Fig. 4.1). However, when bacteria are precluded from entering the pulp, the tissue exhibits a remarkable regenerative capacity. This phenomenon was demonstrated in a classic investigation where environmentally controlled conventional and germ-free laboratory rats were subjected to experimentally induced and untreated pulp exposures in molar teeth (Kakehashi et al. 1965). The conventional rats were exposed to the normal milieu of microflora while the gnotobiotic rats lived in a completely sterile environment and received sterile food. Both groups of test animals were sacrificed at intervals of 1 to 42 days and their teeth evaluated histologically. The conventional rats developed necrosis of the exposed pulps after 8 days, characterized by chronic inflammation and the formation of periapical lesions due to bacterial invasion. A completely different response was observed in the gnotobiotic rodents. Dentin bridging was evident at 14 days and all older specimens exhibited matrix formation and vital pulp tissue beneath completely sealed reparative dentin bridges. All specimens showed minimal pulpal inflammation histologically and more importantly, the germ-free rats did not develop periapical pathosis.
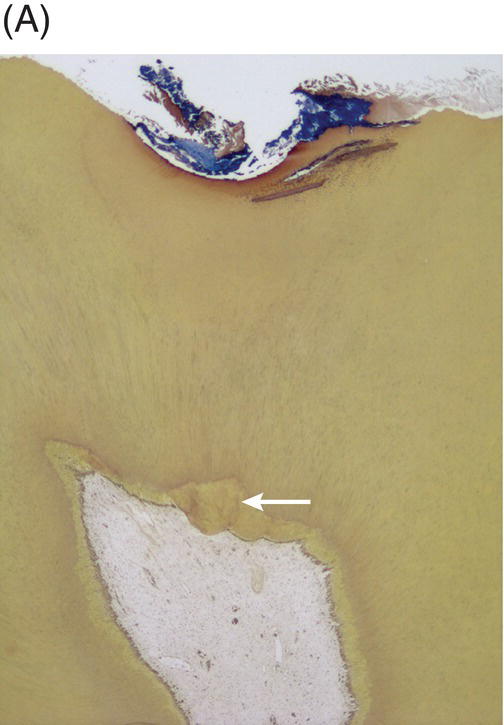
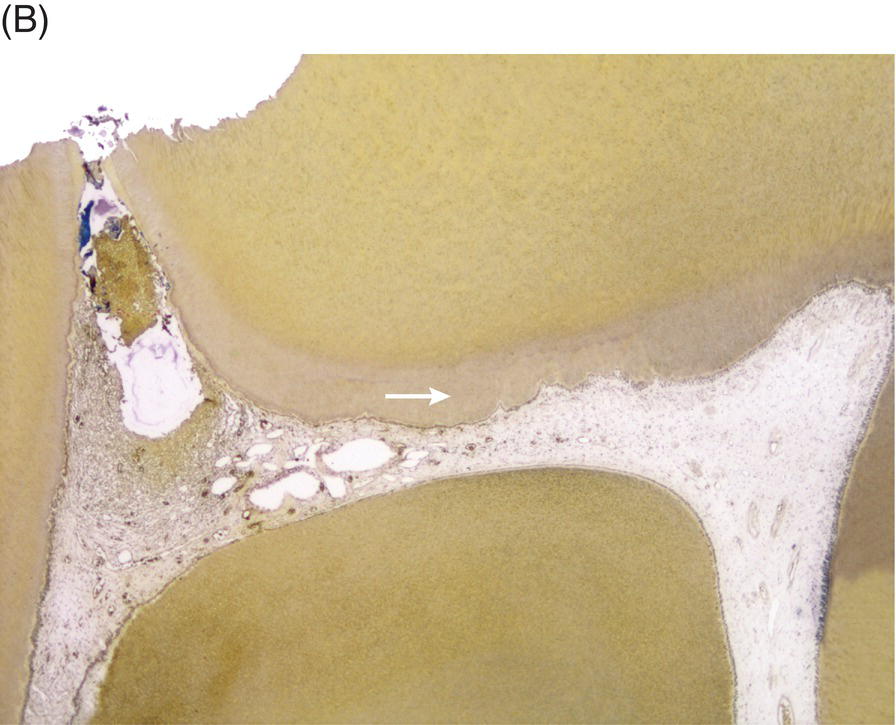
Fig. 4.1 (A) Photomicrograph of an asymptomatic human tooth with a carious lesion, bacterial invasion of dentinal tubules, tertiary dentin formation (arrow) and a vital pulp. (B) Photomicrograph of symptomatic human tooth with penetration of bacteria into distal pulp horn showing pulpal necrosis while mesial pulp tissue remains vital and is uninflamed with normal appearance of dentin, predentin and odontoblast layer. Note formation of tertiary dentin (arrow). Original magnification × 16, Taylor modified B & B Stain.
Courtesy of Dr. Domenico Ricucci.
Histological examination of teeth from germ-free rats revealed an important feature of the healing process. Cells lining the newly-formed hard tissue (odontoblast-like cells) were organized into a single cell layer similar to primary odontoblasts; however, morphologically they were shorter than the original elongated cells. Odontoblasts are terminal, post-mitotic cells that, if damaged, do not have the capacity to divide and generate new odontoblasts. The hard tissue-forming cells observed in the germ-free rats were not conventional odontoblasts, but were specialized secretory cells differentiated from recruited mesenchymal cells (fibroblasts) found within the pulp stroma (Smith et al. 1995).
This study helps in understanding the basic concept and premise for vital pulp therapy. Bacterial contamination of the exposed pulp tissue must be abated by immune-component responses followed by cell recruitment from the dentin–pulp complex and hard tissue formation completed by differentiated progenitor cells rather than by survival or regeneration of the original cells. Minor indirect trauma to pulp tissue (without exposure of the tissue) stimulates existing primary odontoblasts to form reactionary dentin. This hard tissue is distinguished from the reparative dentin formed by differentiated mesenchymal cells after a pulp exposure. These two situations can describe certain instances of indirect and direct pulp capping where the original hard tissue-forming cells may either survive or be irreversibly damaged (American Association of Endodontists 2003).
Clearly, in clinical practice a sterile environment for an exposed pulp cannot be provided, so an artificial barrier between the vital pulp and external environment is placed to protect the pulp during the healing phase. These artificial barriers are pulp capping materials. The primary aim of pulp capping and pulpotomy has been to establish an environment that induces hard tissue formation by the remaining pulp cells and seals the exposure site, ultimately contributing to continued pulp vitality (Schröder 1985; Lim & Kirk 1987; Moghaddame-Jafari et al. 2005). The techniques and rationale for achieving this goal in vital pulp therapy will be described later in this chapter.
ADVANTAGES
Although the effectiveness of conventional root canal treatment is irrefutable, an important advantage of retaining pulp vitality appears to be the continued function of proprioceptive mechanisms and the protective avoidance of excessive occlusal forces during mastication. A root canal-treated tooth requires two and a half times greater occlusal loading to register a proprioceptive response than a tooth with a vital pulp (Randow & Glantz 1986; Stanley 1989). Therefore, greater pressure can be applied to a pulpless tooth before a reflexive response to relieve the force is activated. This diminished protective mechanism in root canal-treated teeth might produce a higher incidence of coronal and radicular tooth fractures (Fuss et al. 2001; Lertchirakarn et al. 2003; Mireku et al. 2010). Root filled teeth also exhibit an increased susceptibility to caries due to either substandard marginal integrity of restorations or a change in their biological environment (Merdad et al. 2011). Furthermore, vital pulp procedures are conservative, comparatively simple and inexpensive treatments that do not require more complex and costly restorative care (Hørsted-Bindslev & Bergenholtz 2003). The long-term retention of teeth with vital pulps and intact protective mechanisms provide superior survival rates compared with root filled teeth (Linn & Messer 1994, Caplan et al. 2005). Therefore, the overall objectives of vital pulp therapy include the elimination of bacteria from the dentin–pulp complex, protection, repair, and promotion of pulp healing in order to postpone more aggressive endodontic and restorative care (Weiger 2001).
PULP RESPONSES TO CAPPING MATERIALS
Many materials, medicaments, and methods have been used historically to treat and protect an exposed pulp. A short list of these agents includes formocresol, ferric sulfate, electrocautery, tricalcium phosphate, and calcium hydroxide (CH). However, all of these treatments and materials have shortcomings when the teeth are evaluated clinically and histologically. Consequently, vital pulp therapy and, specifically, direct pulp capping, have been considered controversial treatment options in permanent teeth due to the inability of traditional materials and treatment protocols to consistently provide a favorable outcome for the involved tooth (Tronstad & Mjör 1972; Langeland 1981; Ward 2002; Witherspoon 2008; Naito 2010).
In order to establish the reliability of materials used for vital pulp therapy, histological assessment of the pulp tissue response is required. Several months after direct pulp capping or pulpotomy, the following responses may be observed:
- regular pulp tissue without signs of inflammation and with a continuous layer of reparative dentin (hard tissue).
- chronically inflamed and infiltrated pulp tissue with the formation of a permeable layer of hard tissue interspersed with tunnel defects.
- highly inflamed pulp tissue with imperfect, incomplete or missing hard tissue formation or dense collagenous scar tissue in the area of pulp injury.
Only the first response can be considered as successful pulp healing because under this condition the pulp tissue will repair itself and survive after injury (Schroeder 1997). Calcium hydroxide is currently the most widely used pulp capping agent and its affect on pulpal tissue has been subjected to comprehensive investigations. Direct pulp capping with CH will be reviewed first to better understand the desirable properties and some disadvantages of this universally accepted pulp capping agent.
DIRECT PULP CAPPING WITH CALCIUM HYDROXIDE
The first published references to direct pulp capping using CH paste (aqueous suspension) were presented by Hermann (Hermann 1928, 1930) and, since the 1960s, hard setting CH salicylate ester cements have been the preferred material. Thus, for many decades, CH has been the standard material for maintaining pulp vitality. Currently, CH products are the best documented and most reliable materials for direct pulp capping, serving as the “gold standard” against which new materials have to be tested (Hørsted-Bindslev et al. 2003). However, more recently, innovative materials have been employed as pulp capping agents, including hydrophilic resins, resin-modified glass ionomer cements, ozone technology, lasers, resins combined with bioactive agents, and various calcium-silicate based cements including mineral trioxide aggregate (MTA; ProRoot MTA, Dentsply/Tulsa Dental Specialties, Tulsa, OK, USA).
Calcium hydroxide exhibits a high pH value and is initially bactericidal. Therefore, the material can neutralize the low pH of acid in carious lesions. It is well known that CH promotes the differentiation of odontoblasts or odontoblast-like cells, which form a hard tissue bridge in the region of the exposed pulp. CH contributes actively to the formation of new hard tissue by induction and up-regulation of the differentiation of odontoblast-like cells (Schröder 1972). Furthermore, low concentrations of CH induce the proliferation of pulp fibroblasts (Torneck et al. 1983).
In general, clinical studies show acceptable histological and clinical results for direct and indirect pulp capping procedures with CH (Dammaschke et al. 2010a). Basic research and clinical studies have reported success rates in excess of 80% for direct pulp capping procedures in humans (Baume & Holz 1981; Hørsted et al. 1985; Duda & Dammaschke 2008; Duda & Dammaschke 2009). By contrast, Barthel et al. (2000) identified teeth with necrotic pulps, root canal fillings, or extractions after 10 years in approximately 75% of the cases examined after direct pulp capping with CH. Thus, pulp capping with CH is not without controversy, as it may not provide reliable bridge formation and pulpal protection for prolonged periods.
Calcium hydroxide has some major drawbacks to consider when selecting a material for pulp capping. The compound exhibits poor bonding to dentin, mechanical instability, and continued absorption after placement (Barnes & Kidd 1979; Cox et al. 1996; Goracci & Mori 1996) (Fig. 4.2). Moreover, the porosities in newly formed reparative dentin known as “tunnel defects” may act as portals of entry for microorganisms as the material absorbs. This can produce secondary inflammation of the pulp tissue and may be responsible for failed maintenance of tooth vitality and dystrophic calcification (Fig. 4.3). As a result, CH does not prevent microleakage over extended periods even when paired with a sealed restoration. Finally, the high pH (12.5) of calcium hydroxide suspensions also causes liquefaction necrosis at the pulp tissue interface (Barnes & Kidd 1979; Cox et al. 1996; Duda & Dammaschke 2008).
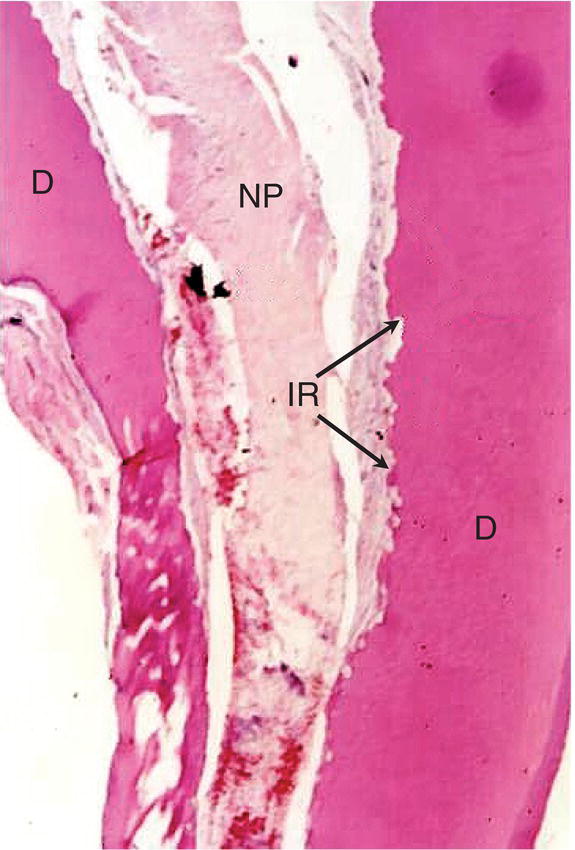
Fig. 4.2 Pulp tissue response to direct pulp capping with Dycal® after 5 months in primary tooth showing pulpal necrosis and internal resorption (D: dentin; NP: necrotic pulp; IR: internal resorption). Magnification ×40.
Source: Caicedo 2008. Reproduced with permission of John Wiley and Sons, Inc.
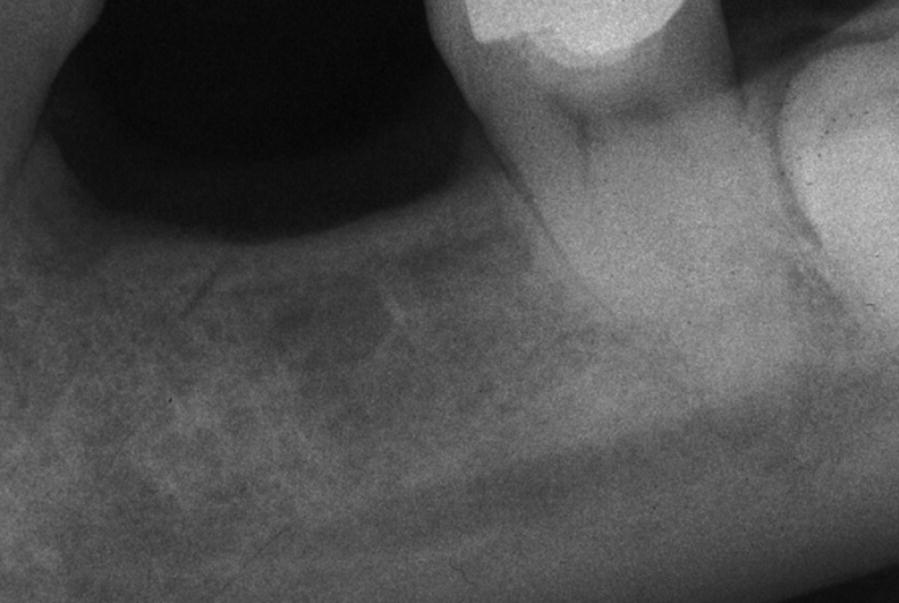
Fig. 4.3 Periapical radiograph of mandibular second molar in a 54-year-old patient. The molar received direct pulp capping approximately twelve years ago, using a hard setting calcium hydroxide (Dycal®). The pulp exhibits a delayed response to cold testing, pulpal calcification and diminished root canal lumen diameter.
MINERAL TRIOXIDE AGGREGATE
Physiochemical properties
The introduction of MTA as a pulp capping material in modern dentistry has transformed treatment outcomes in direct pulp capping from a procedure previously considered unpredictable and often avoided. MTA is a hydraulic calcium–silicate cement powder, which contains different oxide compounds (sodium and potassium oxides, calcium oxide, silicon oxide, ferric oxide, aluminum oxide and magnesium oxide). MTA’s composition is similar to that of a refined Portland cement, which is available in most hardware stores (Camilleri et al. 2005; Dammaschke et al. 2005). Tricalcium silicate is a major component of both and the material is known to be biocompatible and bioactive (Laurent et al. 2009). Bioactivity denotes the positive effect of a medicament or a material on living tissue. A material is called bioactive if it can interact with cells of the human body or show a positive biological effect on cells (Hench & West 1996).
The introduction of MTA to dentistry was an historical milestone in the search and development of bioactive cements used for endodontic purposes. However, the first publications advocating the use of Portland cements in dentistry occurred before the end of the nineteenth century. In 1878 the German dentist D. Witte (Hanover, Germany) described the use of a commercial Portland cement to fill root canals and to treat vital pulp tissue (Witte 1878). Unfortunately, the use of this material was apparently not pursued after this period.
The first commercially available version of MTA (ProRoot MTA) was gray (GMTA) in color, and produced tooth discoloration if used within the clinical crown (Karabucak et al. 2005). The product was therefore reformulated in a yellow–white version as white MTA (WMTA) (Glickman & Koch 2000). GMTA contains chromomorphic ferrous compounds that include tetracalcium aluminum ferrite, which is absent in WMTA (Moghaddame-Jafari et al. 2005). Furthermore, the concentration of aluminum oxide, magnesium oxide, and ferric oxide in WMTA is significantly lower than in GMTA (Asgary et al. 2005). Despite component differences, direct pulp capping studies demonstrate that the histological reactions to GMTA and WMTA are similar (Faraco Júnior & Holland 2001, 2004; Parirokh et al. 2005).
Both GMTA and WMTA induce visible hard tissue formation with only mild signs of inflammation without substantial necrosis (Aeinehchi et al. 2003; Accorinte et al. 2008a, 2008b; Nair et al. 2008). The hard tissue formed is amorphous and without dentinal tubules (Faraco Júnior & Holland 2004; Parirokh et al. 2005) (Fig. 4.4). However, a recent study has shown tunnel defects in reparative dentin formed after MTA pulp capping in non-human primates. This feature of the dentin bridge may be of little consequence as set MTA is structurally stable and nonabsorbable (Al-Hezaimi et al. 2011). Unfortunately, tooth discoloration was also recently described after the use of WMTA in vital pulp therapy (Belobrov & Parashos 2011). This effect can be minimized by the use of dentin bonding agents inside the clinical crown in some MTA applications (Akbari et al. 2012).
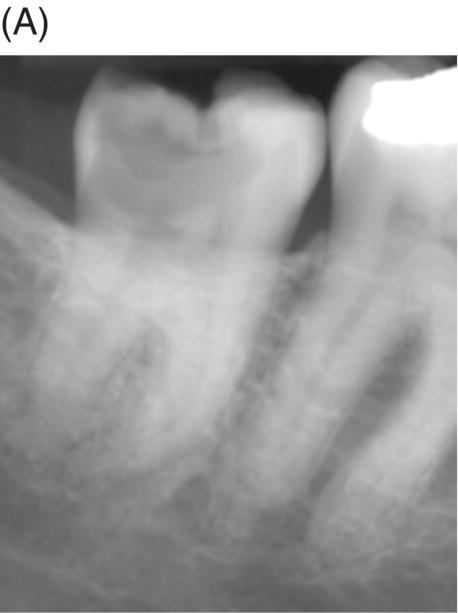
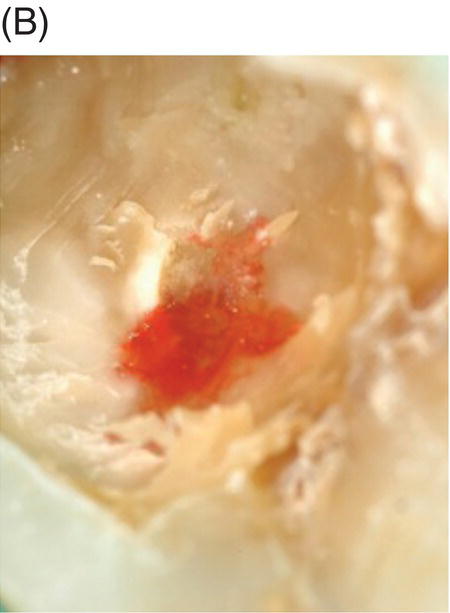
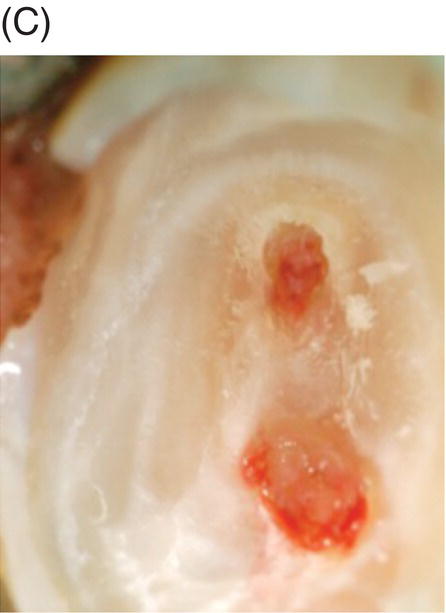
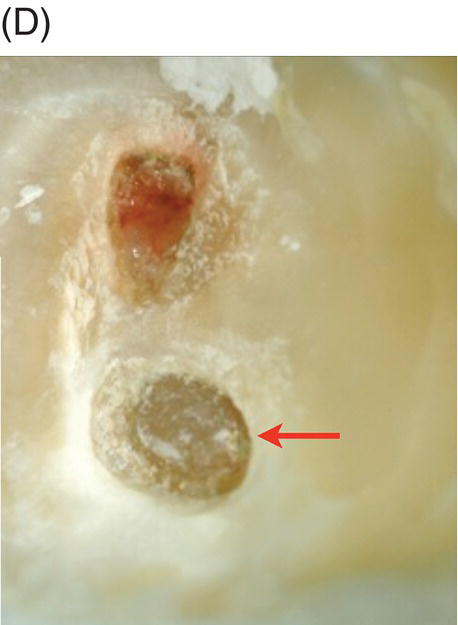
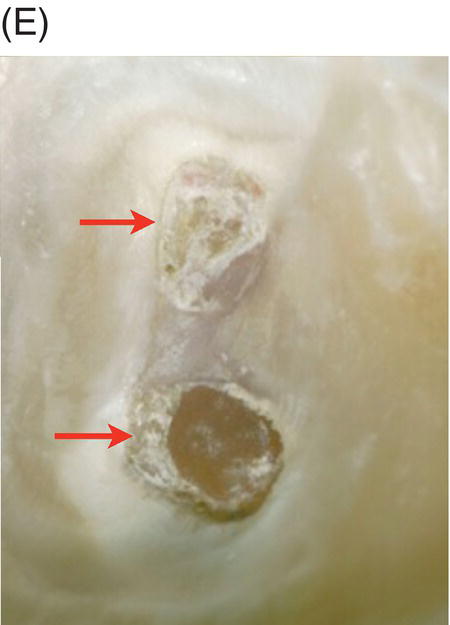
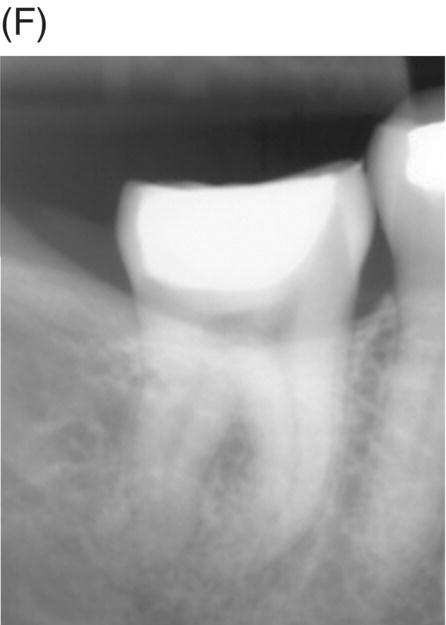
Fig. 4.4 (A) Radiograph of mandibular right asymptomatic second molar in 50-year old patient exhibiting deep caries proximal to pulp roof. (B) Photograph of initial pulp exposure during caries excavation. (C) Clinical photograph showing two large pulp exposures after hemostasis before direct MTA pulp capping. (D) Clinical photograph after re-entry and MTA removal at 3.5 months showing hard tissue formation at one exposure site (arrow). (E) Photograph of tertiary dentin formation after re-entry and MTA removal at 7 months (arrows). (F) Seven-month radiograph after permanent restoration placement. The patient was asymptomatic.
Courtesy of Dr. Domenico Ricucci.
In the clinical setting, MTA is mixed on a glass slab or in a Dappen dish with sterile water or anesthetic solution. After adding water to the MTA powder, it reacts to form a colloidal gel that sets within 4 hours (Torabinejad et al. 1995a). If set MTA comes in contact with tissue fluids, its calcium oxide converts into CH. The CH molecule dissociates into calcium and hydroxyl ions (Holland et al. 1999; Faraco Júnior & Holland 2001; Takita et al. 2006), increasing the pH to a value between 9.22 (Duarte et al. 2003) and 12.5 (Torabinejad et al. 1995a). Therefore, MTA and CH have similar features that include antimicrobial properties (Al-Hezaimi et al. 2005). Furthermore, MTA and CH may exhibit comparable mechanisms that induce new hard tissue formation when in contact with vital pulp tissue (Dominguez et al. 2003). However, MTA exhibits superior mechanical properties that discernibly differentiate the two materials.
The advantages of MTA in direct pulp capping, when compared with CH, include lower solubility, improved mechanical strength, and superior marginal adaptation to dentin (Sarkar et al. 2005). Furthermore, using MTA for direct pulp capping eliminates some of the disadvantages of CH, such as absorption of the capping material, mechanical instability, and subsequent inadequate long-term sealing ability due to leakage (Dammaschke et al. 2010c). MTA is a hydrophilic and hygroscopic cement that allows the material to set in the presence of blood and tissue fluids (Torabinejad et al. 1995a).
It is known that calcium silicate cements like MTA not only have the ability to release calcium and hydroxyl ions after contact with cell and tissue fluid (Borges et al. 2011), but also to form hydroxyapatite crystals on its surface (Sarkar et al. 2005; Bozeman et al. 2006; Gandolfi et al. 2010). The apatite formation contributes to leakage reduction not only by filling the gap along the interface but also via interactions with dentin during intrafibrillar apatite deposition (Han & Okiji 2011). This characteristic “interstitial layer” formation shows a similar composition and structure to hydroxyapatite when placed in contact with dentin and may be the most important physiochemical property of MTA in vital pulp therapy (Sarkar et al. 2005; Bozeman 2006). This feature makes it effective in preventing microleakage, thereby improving the treatment prognosis by providing a biologically active substrate for cell attachment (Sarkar et al. 2005). Furthermore, MTA is antibacterial (Torabinejad et al. 1995d; Ribeiro et al. 2006), not mutagenic (Kettering et al. 1995) and exhibits minor cytotoxicity (Keiser et al. 2000). It does not alter the cytomorphology of osteoblasts (Koh et al. 1998), promotes a biological cell reaction in these cells (Koh et al. 1997; Mitchell et al. 1999) and induces the formation of mineralized tissue (Abedi & Ingle 1995; Holland et al. 2001).
MTA is covered by cementoblasts when used for perforation repair in the area of the periodontal ligament (Holland et al. 2001). Moreover, human osteoblasts attach to MTA’s surface, allowing cell survival (Zhu et al. 2000). Overall, all available studies show MTA to have excellent biocompatibility (Torabinejad et al. 1995b; Pitt Ford et al. 1996; Koh et al. 1997; Torabinejad & Chivian 1999; Keiser et al. 2000) with an exceptional sealing ability against microbial challenges (Torabinejad et al. 1993, 1995e; Torabinejad & Chivian 1999). It can be concluded that current research indicates MTA is the preferable material for direct pulp capping and the rational alternative to CH in vital pulp therapy (Holland et al. 2001; Cho et al. 2013) (Fig. 4.5).
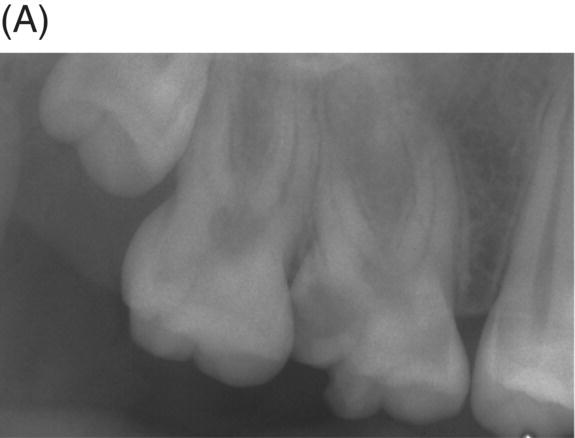
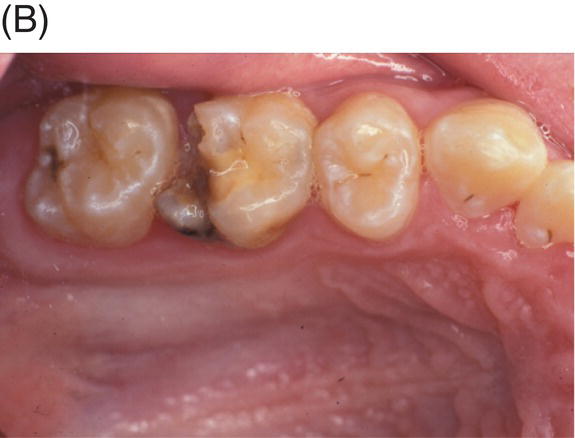
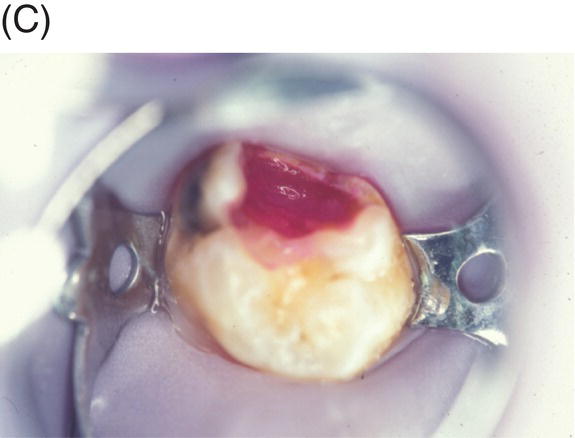
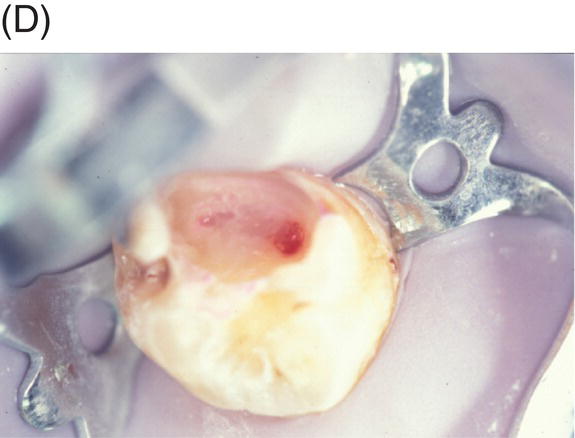
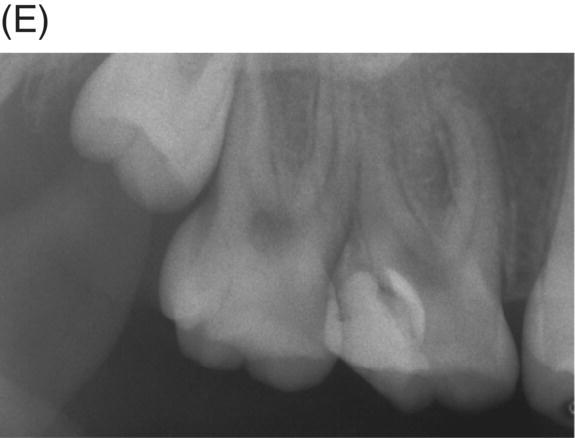
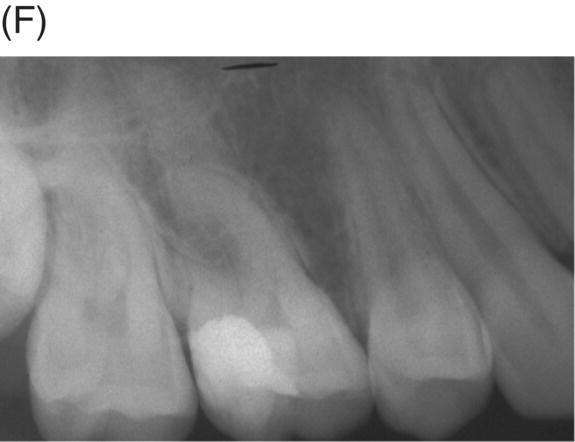
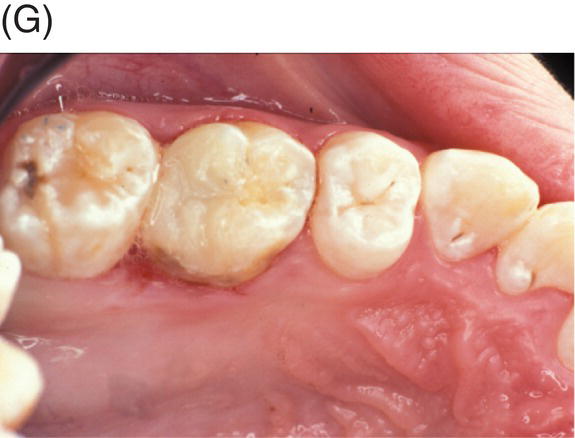
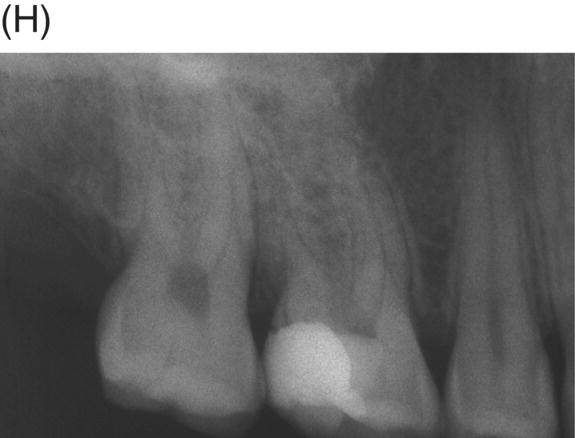
Fig. 4.5 (A) Preoperative radiograph of deep caries associated with maxillary right molar in symptomatic 15-year-old patient. (B) Clinical photograph of involved molar. (C) Caries removal using caries detector dye. (D) Clinical view of pulp exposures after 5.25% NaOCl hemostasis. (E) Radiograph of direct MTA pulp cap with wet cotton pellet and unbonded Photocore®. (F) Permanent bonded composite placed five days after MTA direct pulp cap. (G) Clinical photograph of final bonded composite restoration. (H) Fourteen-year recall radiograph without restorative intervention, patient responded normally to cold testing.
Mode of action in pulp capping and pulpotomy
MTA has been shown to promote a variety of positive cellular responses in vitro when applied directly to the dental pulp (Bonson et al. 2004; Nakayama et al. 2005; Tani-Ishii et al. 2007). It also has a substantial effect on the mitosis index of progenitor cells and stimulates hard tissue formation after direct pulp capping (Dammaschke et al. 2010b). Progenitor cells are multipotent adult stem cells that have the potential to differentiate into odontoblast-like cells after injury or damage to the primary odontoblasts (Goldberg & Smith 2004, Goldberg et al. 2008). MTA most probably stimulates mineralization by up-regulation of bone morphogenic protein (Yasuda et al. 2008). In vitro, MTA promotes the production of mineralization matrix genes, mRNA, and a protein expression of cellular markers, which play a role in the mineralization process (Thomson et al. 2003).
When MTA is placed in direct contact with pulp cells, there is a significant increase in the induction and secretion of vascular endothelial growth factor (VEGF), a platelet-derived protein growth factor critical for angiogenesis and shown to be involved in dentinogenesis (Paranjpe et al. 2010, 2011). Moreover, in comparison with untreated control groups, MTA induces a significant increase of MDPC-23-cells in the S- and the G2-phases and of OD-21-cells in the S-phase of the cell cycle in vitro. However, MTA does not influence the apoptosis of these cells. Therefore, it can be concluded that MTA promotes the proliferation, but not apoptosis of pulp cells. This may explain the regenerative processes observed after direct pulp capping with MTA in vivo (Moghaddame-Jafari et al. 2005; Caicedo et al. 2006) (Fig. 4.6).
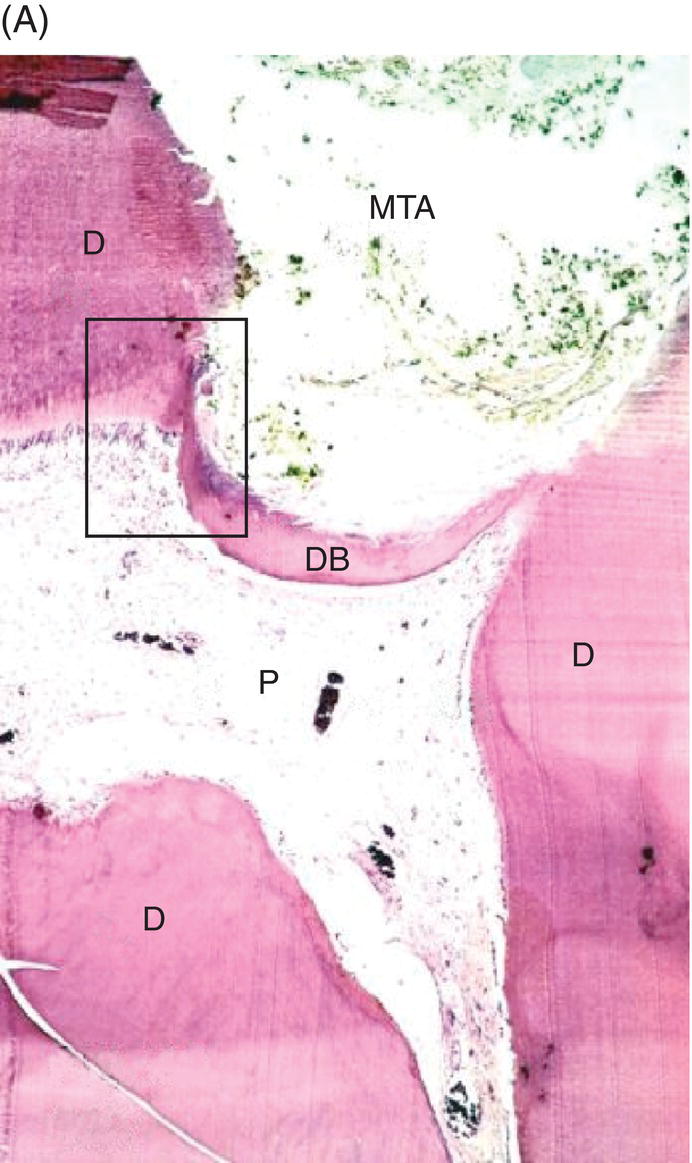
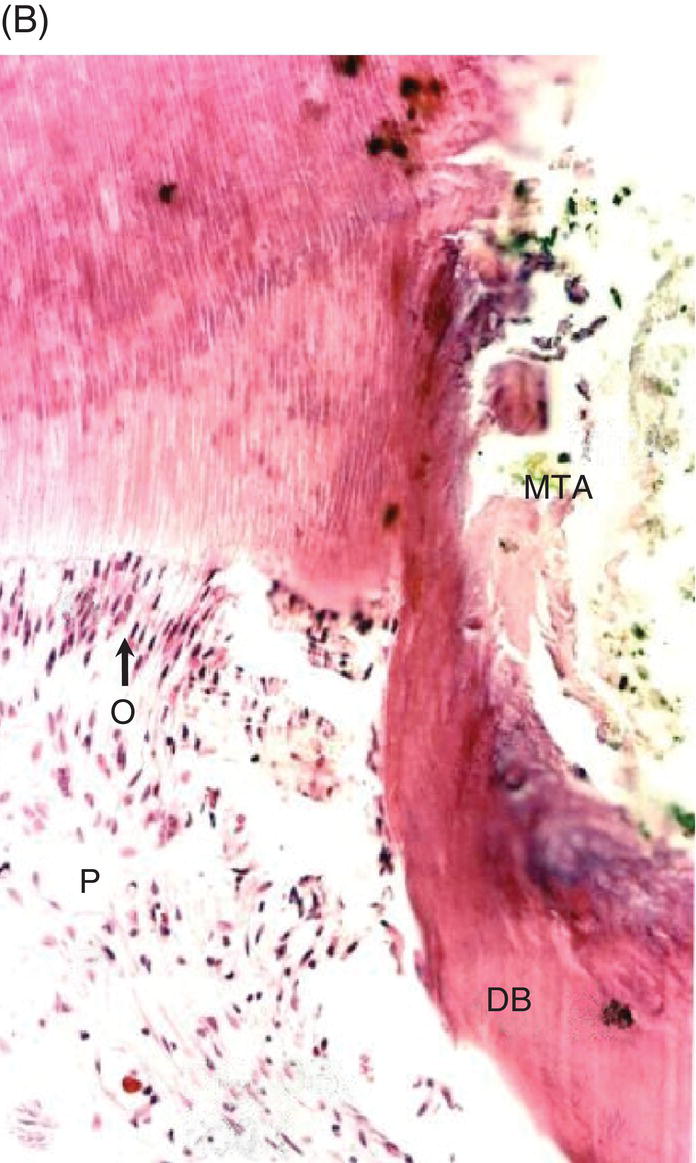
Fig. 4.6 (A) Direct pulp capping with MTA in a human primary tooth showing dentin bridge formation after five months (D: dentin; P: dental pulp; DB: dentin bridge). Magnification ×40. (B) Odontoblasts (O) proximal to MTA induced dentin (hard tissue) bridge. Magnification ×200.
Source: Caicedo 2008. Reproduced with permission of John Wiley and Sons, Inc.
Essential physiochemical properties attributed to MTA promote reparative dentinogenesis by uncoupling and activating growth factors nested in the proximal dentin (Koh et al. 1997; Tziafas et al. 2002; Okiji & Yoshiba 2009). Signaling molecules, including transforming growth factor-β (TGF-β), macrophage colony-stimulating factor (MCSF), and interleukins IL-1α and IL-β, are stimulated via the continued release of calcium ions during the setting process (Takita et al. 2006; An et al. 2012). MTA is able to improve the secretion of IL-1β significantly better compared with other capping materials like CH or hydrophilic bonding resins (Accorinte et al. 2008c; Reyes-Carmona et al. 2010; Cavalcanti et al. 2011; Galler et al. 2011). Interleukin-1 beta is a highly effective cytokine that controls the growth and differentiation of cells (Cavalcanti et al. 2011). It has been proposed that glycoproteins in the extracellular matrix can stimulate the formation of reparative or reactionary dentin (Smith et al. 1995; Goldberg & Smith 2004; Goldberg et al. 2008). In particular, tenascin and fibronectin have been identified as high molecular weight oligomeric proteins involved in odontoblastic differentiation and odontogenesis in the presence of MTA (Thesleff et al. 1995; Leites et al. 2011; Zarrabi et al. 2011). Both glycoproteins are expressed during dentinogenesis and may be critical elements in pulp cell migration and differentiation (Zarrabi et al. 2011).
Proliferation and survival of human dental stromal cells is increased when cultured on set GMTA. Gene products such as osteocalcin, dental sialoprotein, and alkaline phosphatase are also up-regulated when in contact with set MTA and promote the differentiation of odontoblast-like cells essential for reparative dentin bridge formation. After MTA pulp capping, both sialoprotein and osteopontin have been identified during initial dentinogenesis at the exposure site (Kuratate et al. 2008). It is known that the presence of bone morphogenetic proteins BMP-2, BMP-4, BMP-7, signaling molecules such as TGF-β, and heme oxygenase-1 enzyme are required for differentiation of the odontoblastic cell line (Guven et al. 2011). The up-regulation of specific cytokines by MTA promotes mineralization by forming apatite-like clusters on collagen fibrils at the MTA-dentin interface (Ham et al. 2005; Yasuda et al. 2008; Reyes-Carmona et al. 2010). The up-regulated cytokines include cyclooxygenase-2, activating protein-1, myeloperoxidase, VEGF, nuclear factor-kappa B, and inducible nitric oxide synthase. MTA has been shown to improve the secretion of IL-1β and IL-8 and does not adversely affect the generation of reactive oxygen species and cell survival (Camargo et al. 2009). However, the release of aluminum ions by MTA may have a partial inhibitory effect on pulp stromal cells (Minamikawa et al. 2011).
Comparison with calcium hydroxide
The positive effect of MTA when in contact with vital pulp tissue has been experimentally evaluated in several species and compared with CH: in humans (Aeinehchi et al. 2003; Iwamoto et al. 2006; Caicedo et al. 2006; Accorinte et al. 2008a, b; Min et al. 2008; Nair et al. 2008; Sawicki et al. 2008; Mente et al. 2010; Parolia et al. 2010), monkeys (Pitt Ford et al. 1996), dogs (Faraco Júnior & Holland 2001; Dominguez et al. 2003, Queiroz et al. 2005; Asgary et al. 2008; Costa et al. 2008), pigs (Shayegan et al. 2009), and rodents (Dammaschke et al. 2010c). These investigations show that from a histological perspective, CH and MTA generate comparable reactions in vital pulp tissue. However, in direct contact with pulp tissue, MTA shows significantly less inflammation (Aeinehchi et al. 2003; Accorinte et al. 2008b; Nair et al. 2008; Parolia et al. 2010), a lower amount of hyperemia and necrosis (Aeinehchi et al. 2003; Dammaschke et al. 2010c), and a more homogeneous dentin bridge formation exhibiting fewer tunnel defects (Nair et al. 2008). The newly-formed reparative dentin is thicker and is characterized by a more uniform odontoblast-like cellular layer observed at the bridge interface (Aeinehchi et al. 2003; Min et al. 2008; Nair et al. 2008; Parolia et al. 2010). This may explain why MTA shows better results clinically when compared with CH in direct pulp capping (Mente et al. 2010; Hilton et al. 2013).
The majority of contemporary pulp capping studies show MTA to be superior to CH (Pitt Ford et al. 1996; Faraco Júnior & Holland 2001; Aeinehchi et al. 2003; Dominguez et al. 2003; Accorinte et al. 2008c; Asgary et al. 2008; Min et al. 2008; Nair et al. 2008; Mente et al. 2010; Leye Benoist et al. 2012; Hilton et al. 2013). However, some authors have found no significant differences in pulp healing between the two substances (Queiroz et al. 2005; Iwamoto et al. 2006; Accorinte et al. 2008b; Costa et al. 2008; Sawicki et al. 2008; Shayegan et al. 2009; Dammaschke et al. 2010c; Parolia et al. 2010) (Table 4.1). These investigations demonstrate that MTA is equal or superior to CH salicylate ester cement or CH powder when used for direct pulp capping (Accorinte el al. 2008a, b; Dammaschke et al. 2010c). Although it has been shown that CH paste generates a more favorable pulpal response than CH salicylate ester cements (Phaneuf et al. 1968; Retzlaff et al. & Castaldi 1969; Stanley & Lundy 1972; Liard-Dumtschin et al. 1984; Schröder 1985; Lim & Kirk 1987; Kirk et al. 1989; Staehle 1990), both materials are prone to absorption over time and therefore more vulnerable to microleakage. Furthermore, some additives necessary for the hard setting of CH may also be toxic to pulp tissue (Liard-Dumtschin et al. 1984). Therefore, MTA has advantages when compared to CH in pulp capping procedures due to better dimensional stability, sustained alkaline pH and equal or improved bioactive properties (Fridland & Rosado 2005; Sarkar et al. 2005; Dreger et al. 2012; Cho et al. 2013).
Table 4.1 Histological results of direct pulp capping with MTA in comparison to CH (Literature review).
Authors | type of Ca(OH)2 | Species | Observation period | Result |
Pitt Ford et al. 1996 | Hard setting cement | Monkey | 5 months | MTA significantly superior |
Faraco Júnior and Holland 2001 | Hard setting cement | Dog | 2 months | MTA significantly superior |
Aeinehchi et al. 2003 | Hard setting cement | Human | 1 week – 6 months | MTA significantly superior |
Dominguez et al. 2003 | Light curing | Dog | 50 days + 150 days | MTA significantly superior |
Accorinte et al. 2008 | Hard setting cement | Human | 30 days + 60 days | MTA significantly superior |
Asgary et al. 2008 | Hard setting cement | Dog | 8 weeks | MTA significantly superior |
Min et al. 2008 | Hard setting cement | Human | 2 months | MTA significantly superior |
Nair et al. 2008 | Hard setting cement | Human | 1 week + 1 month + 3 months | MTA significantly superior |
Mente et al. 2010 | Aqueous paste | Human | 12–80 months (median 27 months) | MTA significantly superiora |
Hilton et al. 2013 |
Hard setting cement |
Human | 6–24 months (median 12.1 months) |
MTA significantly superior |
Queiroz et al. 2005 | Aqueous paste | Dog | 90 d | No significant difference |
Iwamoto et al. 2006 | Hard setting cement | Human | 136 ± 24 days | No significant difference |
Accorinte et al. 2008b | Powder | Human | 30 days + 60 days | No significant difference |
Costa et al. 2008 | Aqueous paste | Dog | 60 days | No significant difference |
Sawicki et al. 2008 | Hard setting cement | Human | 47–609 days | No significant difference |
Shayegan et al. 2009 | Hard setting cement | Pigs | 21 days | No significant difference |
Dammaschke et al. 2010 | Aqueous paste | Rat | 1, 3, 7, 70 days | No significant difference |
Parolia et al. 2010 | Hard setting cement | Human | 15 days + 45 days | No significant difference |
aThe statistical results of Mente et al. (2010) were found by Naito (2010) to be inappropriate. Hence, these results should be regarded with care.
PULPOTOMY IN PRIMARY TEETH
Pulpotomy is defined as the amputation of the affected or infected coronal exposed pulp preserving the vitality and function of the remaining radicular pulp (American Academy of Pediatric Dentistry 2011). Pulpotomy on primary teeth is indicated for pulpal exposures in which the inflammation and/or infection has been judged to be confined to the coronal pulp. With more widespread inflammation into the radicular pulp the tooth is a candidate for pulpectomy and root canal filling or extraction. Hemorrhage control is the key diagnostic determinant in the assessment of irreversibly inflamed tissue and the decision for more aggressive treatment.
In the primary dentition with a mechanical or carious exposure, all the coronal pulp tissue is amputated. Following tissue removal, the pulp chamber should be thoroughly irrigated with a sodium hypochlorite (SH) soaked cotton pellet to remove debris and inspected for any remaining pulpal filaments. Hemostasis cannot be achieved with pulp filaments remaining in the chamber and is accomplished by applying pressure on the pulp stumps with a cotton pellet dampened with SH (1.25–6.0%). If hemostasis is not obtained in 2–3 minutes, this indicates the spread of inflammation into the radicular pulp and the pulpotomy is abandoned in favor of a pulpectomy or extraction. The pulp should never be capped with hemorrhage present as this will result in failure of the procedure (Matsuo et al. 1996). Once hemostasis is attained, the radicular pulp is covered with the medicament or pulp capping material of choice. The chamber is then sealed to prevent the ingress of microorganisms. In primary molars, the placement of a stainless steel crown is the preferred final restoration (Camp & Fuks 2006; Winters et al. 2008; McDonald et al. 2011).
Numerous pharmacotherapeutic agents have been utilized for pulpotomy in the primary dentition. These include CH, formocresol (FC), glutaraldehyde (GA), ferric sulfate (FS), MTA, and collagen. Formocresol continues to be the most widely used medicament for pulpotomy procedures today. However, major concerns about toxicity, allergicity, carcinogenicity, and mutagenicity have led to much criticism and decreased usage (Duggal 2009; Lewis 2010). Electrosurgery (Oringer 1975; Ruemping et al. 1983; Shaw et al. 1987, Shulman et al. 1987) and lasers (Elliot et al. 1999; Liu et al. 1999) also have been successfully implemented for tissue removal and hemostasis.
MTA PULPOTOMY
Primary teeth
Since its introduction, MTA has been used as a dressing in pulp capping and pulpotomy procedures in both primary and permanent teeth. Comparative studies have shown MTA to be equal to (Aeinehchi et al. 2007; Moretti et al. 2008; Subramaniam et al. 2009; Ansari et al. 2010; Erdem et al. 2011) or superior to (Salako et al. 2003; Agamy et al. 2004; Farsi et al. 2005; Holan et al. 2005; Fuks & Papagiannoulis 2006; Zealand et al. 2010) other medicaments and materials used for primary tooth pulpotomies. Most of the reports are comparative in nature, employing clinical signs and symptoms with radiographic interpretation to determine success or failure.
Long-term clinical trials have also shown MTA to produce better results than FC when used for pulpotomy in primary teeth (Farsi et al. 2005; Holan et al. 2005; Zealand et al. 2010). Although radiopaque calcified tissue leading to canal obliteration can be seen in over half of the cases using both materials, MTA demonstrates dentin bridging absent with FC (Farsi et al. 2005; Caicedo el al. 2006; Zealand et al. 2010) (Fig. 4.7). Formocresol also has also been reported to show a greater amount of root resorption than MTA (Aeinehchi et al. 2007; Moretti et al. 200/>
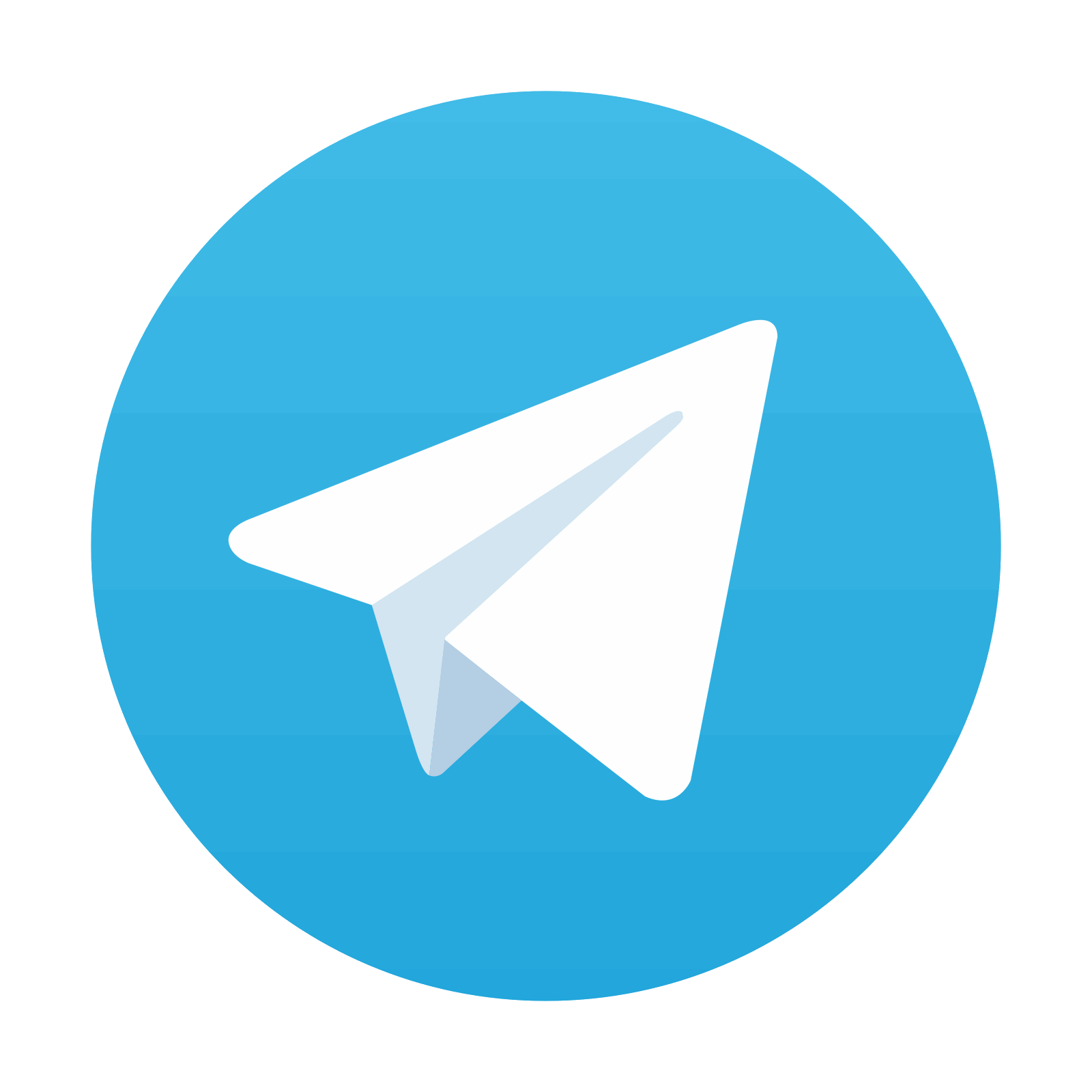
Stay updated, free dental videos. Join our Telegram channel

VIDEdental - Online dental courses
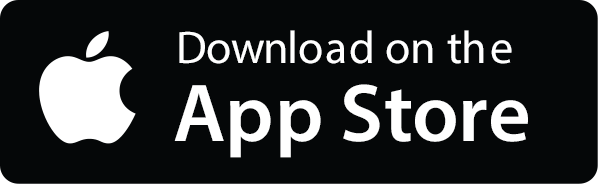
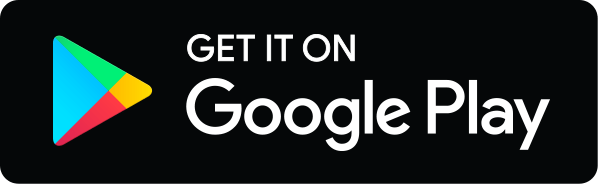