chapter 3
The Neurophysiology of Orofacial Pain
Now that the anatomy of the peripheral and central nervous systems has been reviewed, the manner by which neural impulses are transferred from one neuron to another will be discussed. This chapter will outline the actual mechanisms that allow for the transfer of impulses from the peripheral sensory receptor into the central nervous system (CNS) and back out again to receptor organs from appropriate action.
The Synapse
Nerve signals are transmitted from one neuron to the next through interneuronal junctions called synapses. Synapses occur between different neurons, predominantly through contact with the dendrites. There are as many as 100,000 small knobs, called presynaptic terminals, that lie on the surfaces of the dendrites and some on the soma of the neuron. It is thought that in some parts of the brain a single neuron may have as many as 400,000 synaptic terminals.1
Impulses that cross these synapses create an action potential that is carried down to the terminal end of the axon to synapse with another neuron. Each presynaptic terminal is separated from its adjacent neuron by a small distance called the synaptic cleft. The synaptic clefts range in size from 200 to 300 Å. An understanding of the neurochemistry of the synapse is basic to controlling the neural pathway of nociception and eventually pain.
Humans have two types of synapses: the chemical synapse and the electrical synapse. Electrical synapses are found in some smooth and cardiac muscles and will not be discussed here. Almost all of the synapses in the CNS are chemical.
Within the presynaptic terminals are two important groups of structures: the synaptic vesicles and the mitochondria (Fig 3-1). The synaptic vesicles contain transmitter substances that, when released into the synaptic cleft, either excite or inhibit the postsynaptic neuron. In a similar sense, the postsynaptic neural membrane has both excitatory and inhibitory receptors. Therefore, if an excitatory transmitter is released in the presence of an excitatory receptor, the neuron is excited. If an inhibitory transmitter is released in the presence of inhibitory receptors, the neural activity is inhibited.
The second important structure in the terminal of the neuron is the mitochondron. Mitochondria provide the adenosine triphosphate (ATP) required to synthesize new transmitter substances.
At the synapse, the membrane of the postsynaptic neuron houses large numbers of receptor proteins (Fig 3-1). These receptor proteins project out into the synaptic cleft and extend into the interior of the postsynaptic neuron. The portion that protrudes into the cleft acts as a binding area for the released neurotransmitters. The portion that extends into the neuron is called the ionophore component and carries neurotransmitters into the neuron by way of channels that can influence cell activity. The characteristics of these channels will be discussed below.
The Dynamic Nerve Terminal
In the early studies of ion channels, terminal receptors, and the cell’s production of specific neurotransmitters in the presynaptic vesicles, it was believed that all these components were fixed to specific types of afferent neurons. More recent studies have demonstrated that these terminals are much more dynamic. Although each nerve is genetically predetermined to carry out specific tasks and transmit needed impulses to the CNS, the neuron is actually quite dynamic. When a postsynaptic neuron is continuously excited with a particular type of stimulation (ie, nociception) the cell itself can activate cellular genes that change its function to these demands. This induction of early gene expression causes the release of proto-oncogenes called c-fos and c-jun.2 The release of these substances by the cell alters messenger RNA (mRNA), which in turn can change the type and number of receptors that are formed on the cell membrane. As the number and type of receptors change, so also is the cell’s function changed. This process is called neuroplasticity and is nature’s way to adapt to environmental demands. Changes such as these can alter the reactivity of the synapse for minutes, days, months, or even years. The importance of this feature will be discussed in future chapters.
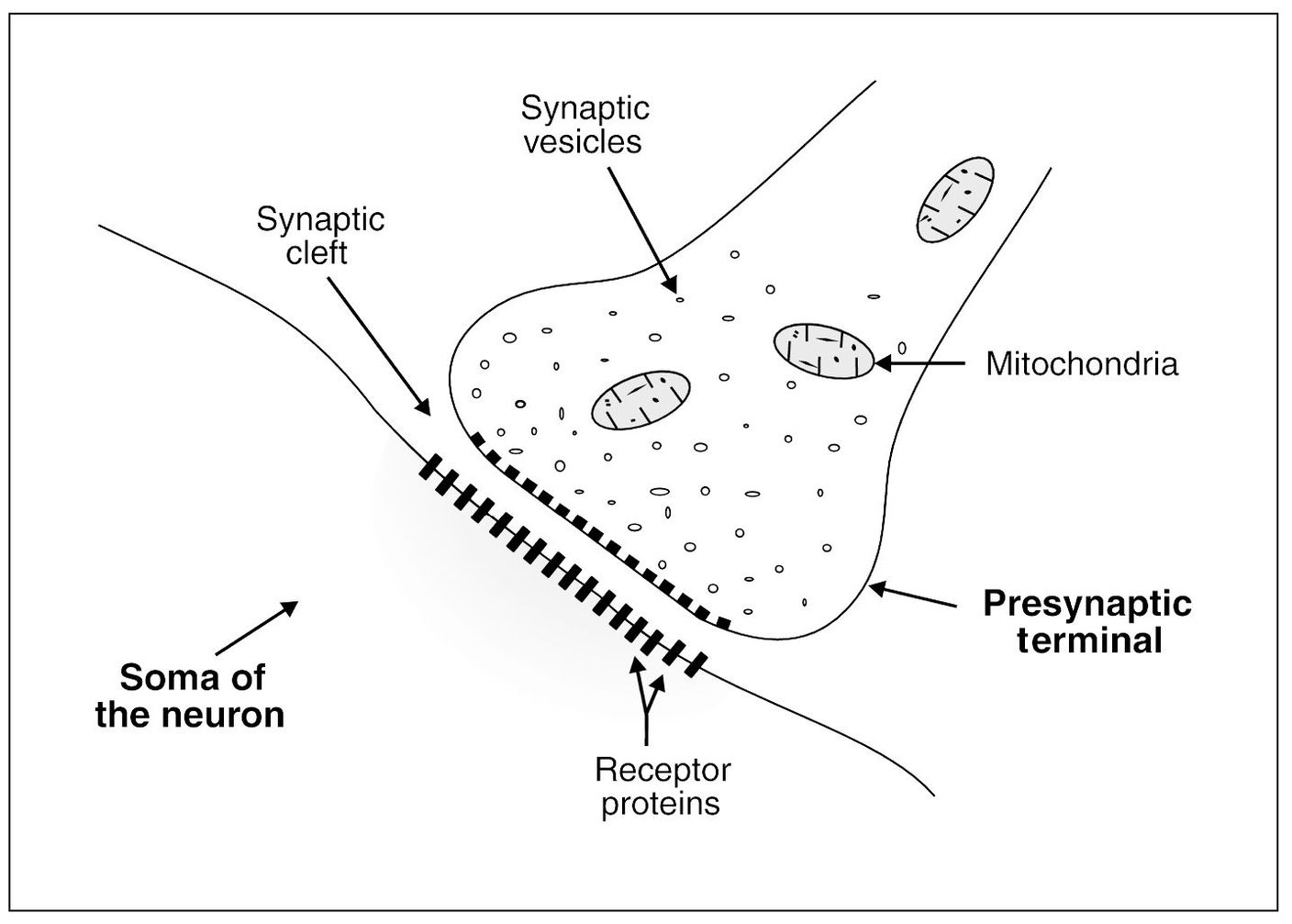
Fig 3-1 The physiologic anatomy of the synapse.
The Ion Channels
The synaptic membrane of the presynaptic terminals contains large numbers of ion channels. There are three general types of ion channels that have been extensively studied: the voltage-gated ion channel, the G-protein–linked ion channel, and the ligand-gated ion channel. Each of these types will be briefly discussed so that the general relationship of the neurochemistry of nociception can be appreciated. There are many more types of channels that have been identified, but it is not within the scope of this text to elaborate on each of these. A more complete description can be found elsewhere. 3
The Voltage-Gated Ion Channels
Voltage-gated ion channels are the basic functional component of the membrane’s ability to depolarize. As has been stated, these channels allow the passing of positive and negative ions in and out of the cell.
The sodium ion channel
When the cell membrane depolarizes to–60 to–40 mV, the sodium ion channel opens rapidly, allowing sodium to enter the cell membrane. In most neurons the sodium channel is primarily responsible for the action potential generation and conduction. There appear to be several subtypes of sodium channels. Some of the channels are blocked by tetrodotoxin (TTX) and some are not.4 The TTX-resistant sodium channel subtype (TTX-R) seems to be more common in C fibers, while the TTX-sensitive sodium channels (TTX-S) are found more often in the large A fibers. The TTX-R channels have a higher depolarization threshold for action potential generation and a faster recovery rate. This suggests that the C fibers are ideally suited to generate sustained bursts of action potentials in response to a prolonged depolarizing noxious stimulus.5,6
The potassium channel
In the presence of depolarization the potassium channel opens rapidly, allowing potassium to leave the cell. Immediately following this, the channel reverses, allowing the return of potassium back into the cell and causing the repolarization of the cell membrane. There are a variety of voltage-gated potassium channels found in sensory neurons.7 These subtypes are responsible for the shifting of potassium in and out of the cell and are therefore important in determining the action potential.
Some potassium channels are activated by calcium. These calcium-activated potassium channels seem to affect the return of the action potential, thus reducing excitability after each action potential. Therefore, these channels are important in regulating the firing pattern of the neuron.7
Neurotransmitters
The neurochemicals that are released by the presynaptic neuron into the synaptic cleft and activate ion channels are called neurotransmitters. Neurotransmitters are either small rapid-acting molecules or larger slower-acting molecules. The smaller rapid-acting transmitters cause most of the acute responses of the nervous system, such as transmission of sensory signals to and inside the brain and motor signals back to the muscles. The larger molecules are the neuropeptides and represent a different group of chemicals. These are not manufactured in the presynaptic terminal but instead in the ribosomes of the neuronal body. The neuropeptides are then transported to the synapse for release in the cleft. These neurotransmitters are much slower acting than the smaller molecules, but when released they have a much longer effect on the postsynaptic neuron. Although this chapter has mainly discussed the peripheral nerve terminal, neurotransmitters are formed and released in all nerves, both peripherally and centrally. Since it is difficult to separate neurotransmitters by location, this discussion will include the common neurotransmitters and mention their more common locations and functions.
Rapid-Acting (Small-Molecule) Neurotransmitters
Some of the more common small-molecule neurotransmitters are listed here, with their common sites of location and effects on the postsynaptic neurons:
Glutamate
Glutamate is an amino acid that is secreted by the presynaptic terminals in many of the sensory pathways, as well as in many areas of the cortex. It has been found in the spinal cord dorsal horn and is associated with noxious input.8,9 It is thought to always cause excitation.
Serotonin
Serotonin (5HT) is secreted by nuclei that originate in the median raphe of the brain-stem and project to many areas of the brain and down into the spinal dorsal horn. Serotonin is a monoamine that is also released by blood platelets. It is synthesized in the CNS from L-tryptophan, a dietary essential amino acid.10 It is released when the nucleus raphes magnus in the brainstem is stimulated by sensory input.11 Peripherally, serotonin is an algogenic agent 12 and is thought to relate especially to neurovascular pain syndromes (see chapter 16). In the CNS, serotonin is an important neurotransmitter in the endogenous antinociceptive mechanism.13 Central serotonin is thought to potentiate endorphin analgesia. 10 It reduces stimulation-evoked excitation of nociceptive dorsal horn interneurons. 14 The activation of serotoninergic pathways in the brainstem by tricyclic antidepressants yields paralleling analgesic effects along with its action on depressive states.15 Descending serotoninergic and norepinephrinergic neurons in the dorsal horn suggest that they provide global suppression or enhancement that enables nociceptive pathways to respond more effectively to incoming sensory information.16
Nitric Oxide
Nitric oxide (NO) is an important intercellular mediator and is produced by many cells that have a close physical association with sensory neurons. NO is formed from L-arginine following the activation of the enzyme nitric oxide synthase (NOS) by calcium and other cofactors.17 The physiologic actions of NO in nociceptive neurons is still unclear, but there is strong evidence that it enhances nociceptive transmission in several ways.18,19
Slow-Acting (Large-Molecule) Neurotransmitters
The large-molecule neurotransmitters are the neuropeptides. The following is a short description of a few of the important neuropeptides that serve as neurotransmitters:
Substance P
Substance P is a polypeptide composed of 11 amino acids. It is released at the central terminals of primary nociceptive neurons and acts as a transport substance, since it is found at the distal terminals as well.20 Centrally, it acts as an excitatory neurotransmitter for nociceptive impulses.21,22 It is released from spinal cord cells by the stimulation of A-delta and C-fiber afferents and excites neurons in the dorsal horn that are activated by noxious stimuli.23 Its modulating action on pain is both rapid and short-lived.24 Substance P released from unmyelinated afferents is involved in neurogenic inflammation such as cutaneous wheal formation and the hyperemia of reflex axon flare. It is known that substance P content is highest in the most severely inflamed joints. When injected into a joint, substance P increases both the inflammation and destructive changes.25
Endorphins
The endorphins are polypeptides (ie, chains of amino acids). They are identical to portions of the pituitary hormone beta-lipotropin, which consists of 91 amino acids.26,27,28 They behave like morphine and bind to morphine receptors to dull pain. Like morphine, they are displaced from these receptors by the morphine antagonist naloxone. Repeated injections of enkephalin and beta-endorphin will cause tolerance and physical dependence.29
The short-chain enkephalins appear to act chiefly in the cerebral spinal fluid. They have a short, rapid action />
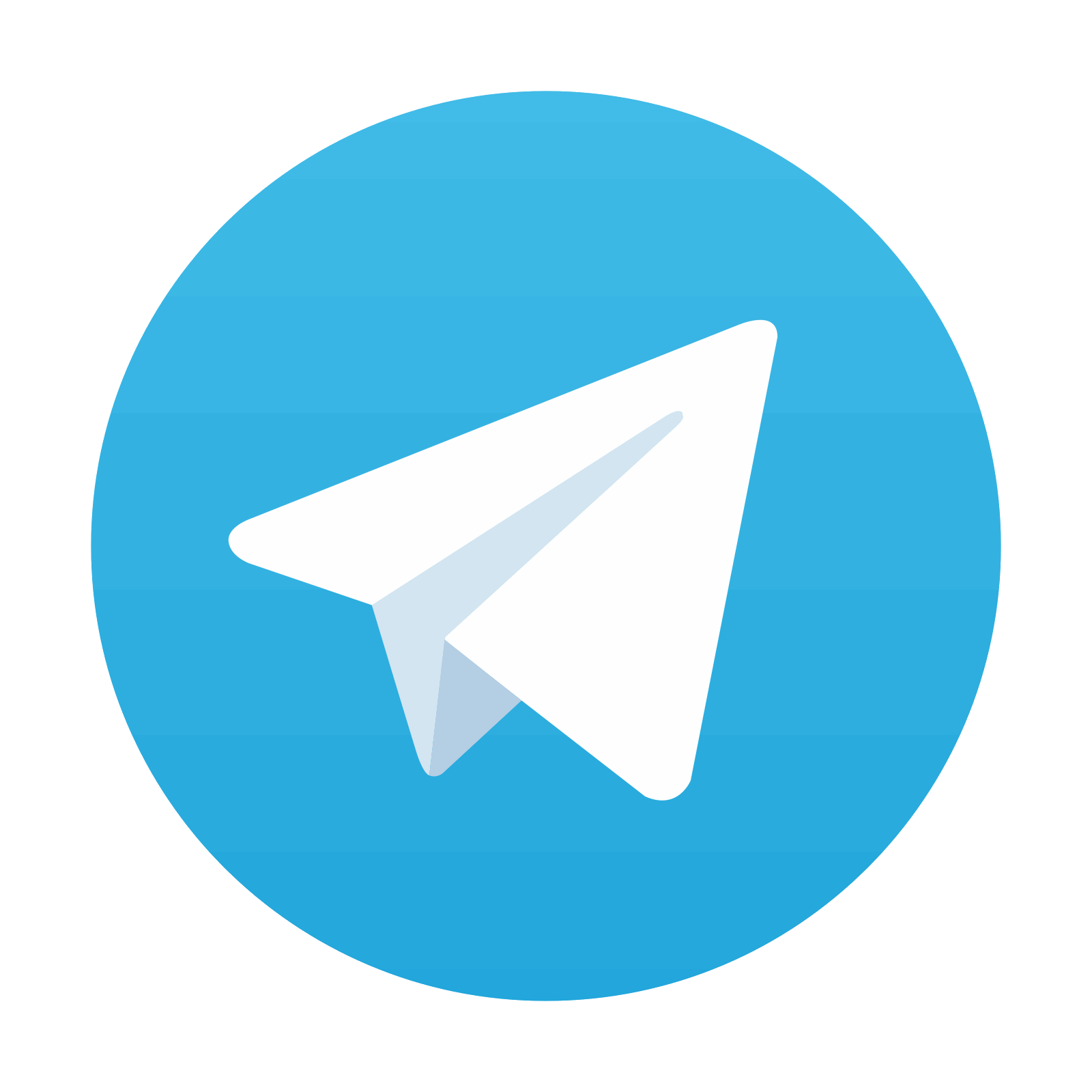
Stay updated, free dental videos. Join our Telegram channel

VIDEdental - Online dental courses
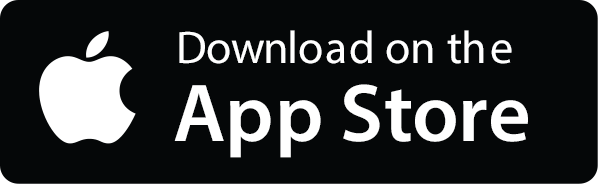
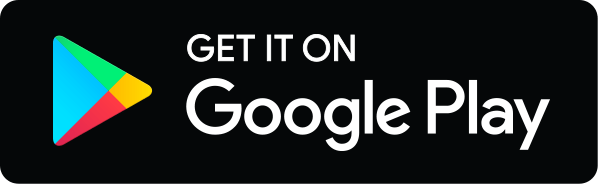