chapter 2
The Neural Anatomy of Orofacial Pain
The most essential element in the management of a patient’s pain problem is an understanding of normal function of the system. This is true in managing any condition. A clinician cannot effectively treat a disorder until he has a sound understanding of order. This basic fact needs to be appreciated by all clinicians. When a patient reports to an office with some type of dysfunction, the clinician’s therapy should be directed toward re-establishing normal function, and the clinician cannot expect to be successful if he or she lacks a sound understanding of normal function. The management of pain disorders is far too complex to develop a simple “cookbook” of therapies. The clinician must understand the unique characteristics of the system so that therapy can be designed appropriately for each patient.
As mentioned in chapter 1, the functional processing of pain can be grossly separated into four categories: transduction, transmission, modulation, and perception. Transduction is the process by which noxious stimuli lead to electrical activity in the appropriate sensory nerve endings. The body has several types of sensory organs that initiate the process of nociception.
The second process, transmission, refers to the neural events that carry the nociceptive input into the central nervous system for proper processing. The neuroanatomy of transduction and transmission will be discussed in this chapter. Modulation and perception will be discussed in chapters 3 and 4.
Neural Structures
Neuron (Nerve Cell)
A nerve is a cordlike structure that has the ability to convey electrical and chemical impulses. It consists of a connective tissue sheath, called the epineurium, that encloses bundles (fasciculi) of nerve fibers, each bundle being surrounded by its own connective tissue sheath, the perineurium. Within each bundle the nerve fibers are separated by interstitial connective tissue called the endoneurium.
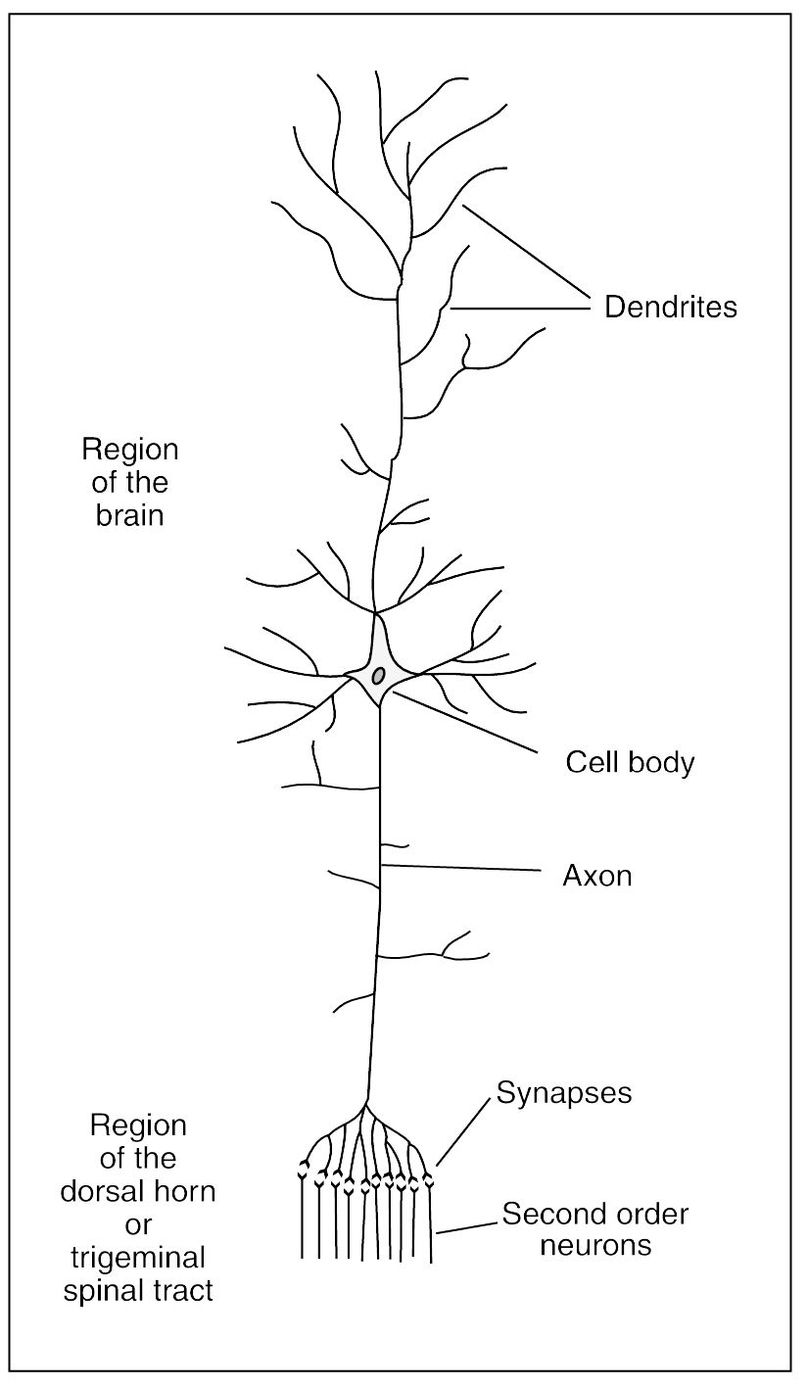
Fig 2-1 The structure of a large neuron of the brain. (Adapted from Guyton AC. Textbook of Medical Physiology, ed 8. Philadelphia: Saunders, 1991:479. Used with permission.)
An individual nerve fiber consists of a central bundle of neurofibrils in a matrix of nerve protoplasm, called the axoplasm, which is enclosed in a thin nerve tissue plasma membrane called the axolemma. Each peripheral nerve fiber is covered by a cellular nerve tissue sheath called the neurolemma (primary sheath or sheath of Schwann). Some of these fibers also have a layer of fatty nerve tissue called the myelin sheath (medullary sheath or white substance of Schwann). Fibers with myelin sheaths form the “white nerves,” and those without myelin sheaths are the “gray nerves.” Constrictions called nodes of Ranvier occur in myelinated nerves at intervals of about 1 mm. These nodes are caused by the absence of myelin material, so that only neurolemma covers the nerve fiber. Nerve fibers in the central nervous system (CNS) have no neurolemma. Those situated in the white substance are myelinated, whereas those in the gray substance are unmyelinated.
Myelination of a nerve fiber affects the resting and action potential of the neuron. The myelin acts as insulation, so that the action potential of a transferring impulse is expressed only at the node of Ranvier. Therefore the impulse travels from node to node, requiring less time to move down the nerve fiber,1 so that myelination increases the conduction velocity of the fiber. The ul-trastructure of the primary trigeminal neuron has been well described2 by use of electron microscopic techniques.
The structural unit of the nervous system is the nerve cell, or neuron. It is composed of a mass of protoplasm termed the nerve cell body (perikaryon), which contains a spherical nucleus (karyon) and gives off one or more processes. The nerve cell bodies located in the spinal cord are found in the gray substance of the CNS. Cell bodies found outside the CNS are grouped together in ganglia. The term nucleus, as applied to the gross structure of the CNS, is used to designate a group of nerve cells that bear a direct relationship to the fibers of a particular nerve. Protoplasmic processes from the nerve cell body are called dendrites and axons (Fig 2-1). A dendrite (from the Greek word dendron, meaning tree) is a branched arborizing process that conducts impulses toward the cell body. An axon (from the Greek word axon, meaning axle or axis) or axis-cylinder is the central core that forms the essential conducting part of a nerve fiber and is an extension of cytoplasm from a nerve cell.
Depending on the number of axons present, a nerve cell is unipolar, bipolar, or multipolar. Peripheral sensory neurons are unipolar. The single axon leaves the nerve cell body, located in the dorsal root ganglion, and branches into two parts: a peripheral branch that extends to terminate in a sensory receptor and a central branch that passes through the root of the nerve to terminate in the gray substance of the CNS.
Depending on their location and function, neurons are designated by different terms. An afferent neuron conducts a nervous impulse toward the CNS, whereas an efferent neuron conducts it peripherally. Interneurons , or internuncial neurons, lie wholly within the CNS. Sensory or receptor neurons, afferent in type, receive and convey impulses from receptor organs. The first sensory neuron is called the primary or first-order neuron. Second- and third-order sensory neurons are interneurons. Motor or efferent neurons convey nervous impulses to produce muscular or secretory effects. A preganglionic neuron is an autonomic efferent neuron whose nerve cell body is located in the CNS and terminates in an autonomic ganglion. A postganglionic neuron has its nerve cell body in the autonomic ganglion and terminates peripherally.
Nervous impulses are transmitted from one neuron to another only at a synaptic junction, or synapse, where the processes of two neurons are in close proximity. All afferent synapses are located within the gray substance of the CNS. It should be noted that the only synapses that normally occur outside the CNS are those of the efferent preganglionic and postganglionic autonomic fibers, and these are located in the autonomic ganglia. This indicates that there are no anatomic peripheral connections between sensory fibers. All connections are within the CNS, and the peripheral transmission of a sensory impulse from one fiber to another is abnormal. Any artificial or false peripheral synapse, called an ephapse, signifies an abnormal or pathologic change.3
Functional Neuroanatomy
Information from the tissues outside the CNS needs to be transferred into the CNS and on to the higher centers in the brain-stem and cortex for interpretation and evaluation. Once this information is evaluated, appropriate action must be taken. The higher centers then send impulses down the spinal cord and back out to the periphery to an efferent organ for the desired action. The primary afferent neuron (first-order neuron) receives a stimulus from the sensory receptor. This impulse is carried by the primary afferent neuron into the CNS by way of the dorsal root to synapse in the dorsal horn of the spinal cord with a secondary (second-order) neuron. The cell bodies of all the primary afferent neurons are located in the dorsal root ganglia. The impulse is then carried by the second-order neuron across the spinal cord to the anterolateral spinothalamic pathway, which ascends to the higher centers. There may be multiple interneurons (third-order, fourth-order, etc) involved with the transfer of this impulse to the thalamus and cortex. There are also very small interneurons located in the dorsal horn that may become involved with the impulse as it synapses with the second-order neuron. Some of these neurons may directly synapse with efferent neurons that are directed back out the CNS by way of the ventral root to stimulate an efferent organ, such as a muscle (Fig 2-2).
Some neural circuits are simple. For example, an impulse from a sensory receptor is carried into the CNS by the primary afferent neuron and synapses with an interneuron. This interneuron in turn synapses with an efferent motor neuron exiting back out the CNS to an efferent organ, such as a muscle. A circuit formed by a chain of neurons in such a way that stimulation is followed by an immediate and automatic response is called a reflex arc. As will be discussed, most reflex arcs or neural circuits are much more complex, involving several and sometimes vast numbers of interneurons, with many possible responses.
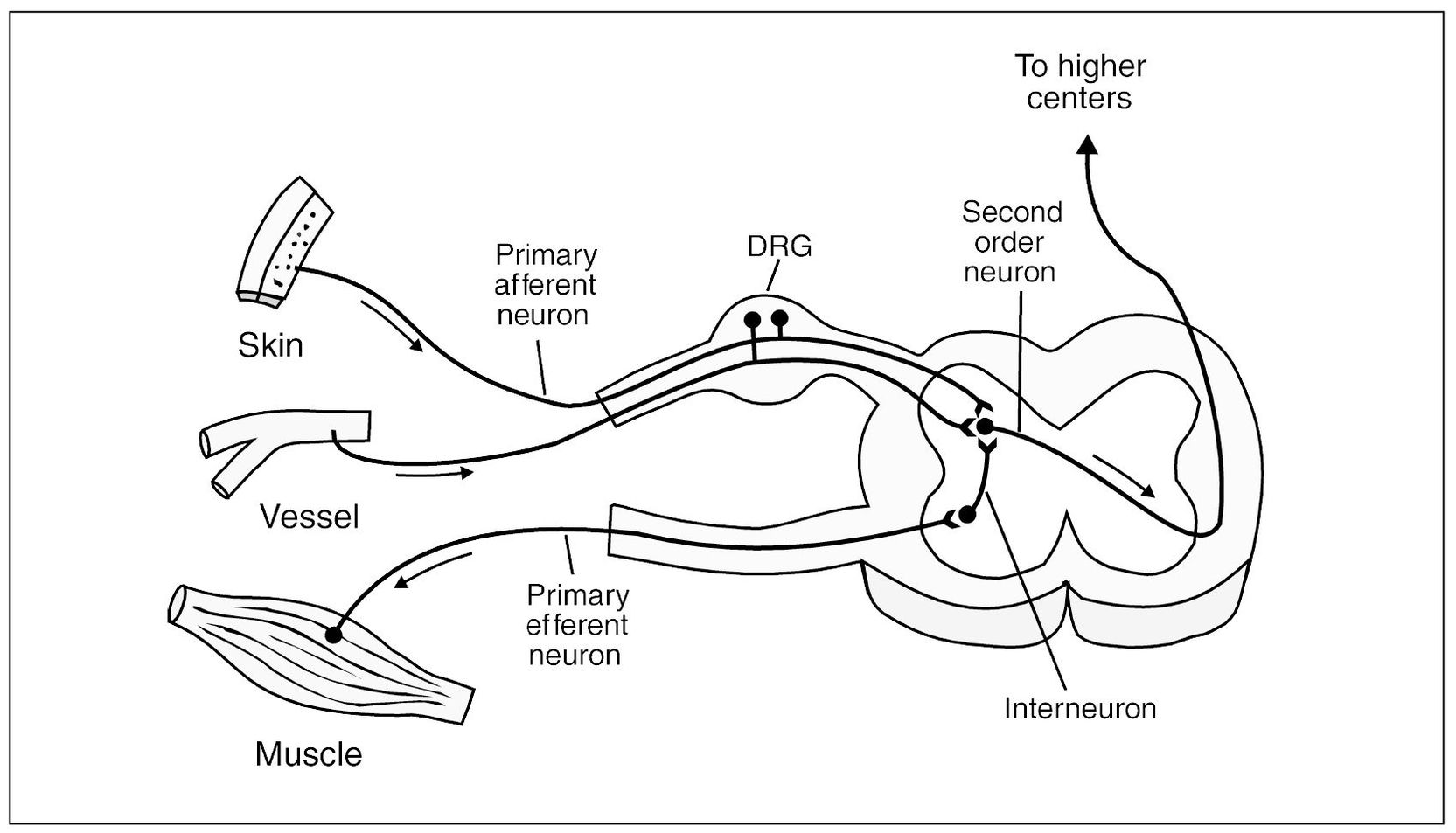
Fig 2-2 Graphic depiction of the peripheral nerve input into the spinal cord. Note that the first-order neurons (primary afferents) carry input into the dorsal horn to synapse with the second-order neurons. The second-order neuron then crosses over and ascends on to the higher centers. Small interneurons connect the primary afferent neuron with the primary motor (efferent) neuron, allowing reflex arc activity. The dorsal root ganglion (DRG) contains the cell bodies of the primary afferent neurons.
Sensory Receptors
At the distal terminals of afferent (sensory) nerves are specialized sensory receptors that respond to physical or chemical stimuli. Once these receptors have been adequately stimulated, an impulse is generated in the primary afferent neuron that is carried centrally into the CNS. Sensory receptors are specific for certain types of stimuli. They can be classified into three main groups, namely, exteroceptors, proprioceptors, and interoceptors.4
Nociceptors
As described above, free nerve endings are found in almost all tissues. These receptors are responsible for identifying tissue injury and are therefore referred to as nociceptors. The tissue area for which the nociceptor is responsible is called its receptive field. Unlike the other types of cutaneous receptors, nociceptors may respond to multiple stimulus modalities, such as mechanical, heat, cold, and chemical stimuli.5 Nociceptors are therefore considered to be polymodal. Early studies of nociceptors only investigated heat and mechanical stimuli, and therefore two types of nociceptors were identified: the C-fiber mechano/heat-sensitive nociceptors (CMH), and the A-fiber mechano/ heat-sensitive nociceptors (AMH). The CMH nociceptor is commonly found in cutaneous tissues and when stimulated in sufficient magnitude is thought to evoke a burning pain sensation. The receptive field of the CMH in humans is about 100 mm.6 However, many of these will overlap each other, giving a particular receptive field more than one nociceptor. CMH nociceptors also respond to chemical stimuli, making them polymodal.7
The AMH nociceptors are thought to evoke pricking pain, sharpness, and perhaps aching pain. As a general rule, the A-fiber nociceptors do what the C-fiber nociceptors do, but they do it more robustly. Although specific pain sensations have been described, further investigations reveal that it is not nearly this simple. Under certain conditions CMH and AMH nociceptors can provoke similar pain sensations.
It is now known that the nerve itself has its own nociceptors. The connective tissue sheath surrounding a peripheral nerve is innervated by primary afferents called the nervi nervorum. The nervi nervorum appear to enter the nerve trunk with the neurovascular bundle.8,9 Because of the nervi nervorum, peripheral nerves can be a source of pain in a manner similar to joints, muscles, and ligaments. The role of the nervi nervorum in certain pain conditions will be discussed in later chapters.
Specialized Receptors and Reflexes
The more highly specialized receptor organs are complex and admirably designed to receive a particular type of stimulus. The neuromuscular receptors, or muscle spindles, have their own sensory and motor innervation comprising a monosynaptic reflex system known as the myotatic or stretch reflex. When muscle spindles are elongated due to passive stretching of the muscle, reflex contraction occurs. This appears to function not only to oppose the forces of gravity but also during reflex and voluntary contraction of muscles, both flexor and extensor.
It should be noted that the neurotendi-nous receptors called Golgi tendon organs respond to tendon stretch and muscle contraction. When these receptors are stimulated, an inhibitory reflex occurs that limits contraction and thus protects the muscle from disruption or detachment. The reflex mechanism involved in this activity is called the nociceptive reflex and is a polysynaptic relay that involves concurrently the contraction of flexor muscles and the inhibition of extensor muscles, resulting in withdrawal of the part stimulated.10,11
When a muscle is maximally stretched, stimulation of Golgi tendon organs induces a reflex that causes contraction to cease and the muscle to relax. This reflex is called the inverse stretch reflex. It should be noted that occasional stretching of a muscle that induces this reflex activity is necessary for the muscle to maintain its normal resting length. If conditions prevent normal operation of this reflex, muscular contracture may occur, which causes the muscle to become functionally shortened.12 For example, the simple act of yawning lengthens the masticatory elevator muscles, stimulating the inverse stretch reflex, which maintains the full range of mouth opening. If the mouth were wired closed because of a mandibular fracture, the elevator muscles would functionally shorten, disallowing full mouth opening at the time of fixation removal. However, as the individual attempted to open the mouth wider, the elevator muscle would be lengthened, stimulating the Golgi tendon organ. This excites the inverse stretch reflex and eventually allows a return of the original functional length of the muscle. This phenomenon is also seen with the lateral pterygoid muscles in edentulous patients. When an individual with complete natural dentition fully inter-cuspates the teeth, the inferior lateral pterygoid muscles lengthen completely, maintaining normal functional length. However, with artificial dentures the resiliency of the interposed underlying soft tissues may not allow full contraction, and therefore muscle contracture can occur in the inferior lateral pterygoid muscles. This can result in a progressive shift of the mandible into a more anterior position.
The simplest type of receptor is the non-encapsulated branching of the axon called the free nerve ending. These terminations are usually described as naked, and they form a network that is especially dense in the cutaneous layers, mucous membranes, and periodontium. In deeper tissues the network is neither as widely spread nor as dense. Simple free nerve endings are no doubt the receptors for nociception and pain, but they are not specific for pain only.
Table 2-1 General Classification of Neurons
Nerve fiber | Diameter | Velocity | |
---|---|---|---|
Type A fibers | |||
Alpha fibers | 13 to 20 µm | 70 to 120 m/s | |
Beta fibers | 6 to 13 µm | 40 to 70 m/s | |
Gamma fibers | 3 to 8 µm | 15 to 40 m/s | |
Delta fibers | 1 to 5 µm | 5 to 15 m/s | |
Type C fibers | 0.5 to 1 µm | 0.5 to 2 m/s |
Stimulation of the free nerve ending receptors can occur as a result of mechanical stimulation (eg, pressure), thermal stimulation (eg, heat), or by chemical stimulation (eg, that produced by substances released following tissue damage).13 It should be noted that free nerve endings are not required for the reception of noxious stimulation, since the nerve fiber itself has the same propensity and the evoked response is similar to that initiated by receptors.
Associated with all vascular tissue, including the endocardium, is a remarkable network of sensory receptors derived from myelinated nerve fibers called the end-net.14 These receptors provide sensory information from the vessels.
The First-Order Neuron
Each sensory receptor is attached to an afferent neuron that carries the impulses to the CNS. Since these neurons are the first to carry the information into the CNS, they are called first-order neurons or primary afferent neurons. The axons of these first-order neurons are found to have varying thickness. It has long been known that a relationship exists between the diameter of nerve fibers and their conduction velocities. 15,16,17 The larger fibers conduct impulses more rapidly than the smaller ones. A general classification of neurons divides the larger fibers from the smaller ones; the larger fibers are called A fibers and the smaller fibers are called C fibers. The A fibers are further divided by diameter into alpha, beta, gamma, and delta. This relationship is summarized in Table 2-1.18
It is generally thought that there is a relationship between fiber size and the type of impulse transmitted. The fast conducting A-alpha, A-beta, and A-gamma fibers carry impulses that induce tactile and proprioceptive responses but not pain. It seems that pain is conducted by A-delta fibers and C fibers, but these are not specific for nociception only.
It is recognized that there are two types of cutaneous pain sensation: pricking pain, which is felt rapidly, and dull, aching, sometimes burning pain, which is slightly delayed. It is thought that these sensations are mediated by different fibers, the pricking sensation by A-delta fibers and the dull aching sensation by C fibers.19 It is known, however, that A-delta fibers also conduct touch, warmth, and cold, whereas C fibers also conduct itch, warmth, and cold.20,21,22
There are three types of afferent neurons that can provide nociceptive input to the CNS:
- Mechanothermal afferents are primarily A-delta fibers that conduct at a velocity of 12 to 15 m/s and respond to intense thermal and mechanical stimuli. They provide a high degree of discriminative information and are peculiar to primates.
- High-threshold mechanoreceptive afferents are chiefly A-delta fibers and normally respond to intense mechanical stimuli in all mammals. They can, however, be sensitized by algogenic substances or repeated noxious stimulation to respond to noxious heat as well (see chapter 4).
- Polymodal afferents are C fibers that conduct much more slowly, at a velocity of 0.5 m/s, and respond to mechanical, thermal, and chemical stimuli in all mammals. At this rate it takes an impulse 2 seconds to go from the big toe to the spinal cord.
Of these three afferent neurons, only the mechanothermal A-delta and C-fiber afferents normally respond to noxious heat.23,24
The Second-Order Neuron
The primary afferent neuron carries impulses into the CNS and synapses with the second-order neuron. This second-order neuron has been called a transmission neuron , since it transfers the impulse to the higher centers. The synapse of the primary afferent neuron and the second-order neuron occurs in the dorsal horn of the spinal cord (see Fig 2-2). The specific anatomy of the trigeminal system will be reviewed later in this chapter.
There appear to be three specific types of second-order neurons that transfer impulses to the higher centers.13,25 These neurons are named according to the type of impulses they predominantly carry. The low-threshold mechanosensitive neurons (LTM neurons) transfer information of light touch, pressure, and proprioception. The nociceptive-specific neurons (NS neurons) exclusively carry impulses related to noxious stimulation. The third type of second-order neuron is called a wide dynamic range neuron (WDR neuron). This neuron is able to respond to a wide range of stimulus intensities from nonnoxious to noxious.
Recently an additional type of second-order neuron has been identified, called a silent nociceptor. The silent nociceptor is an afferent neuron that appears to remain inactive or “silent” to any mechanical stimulation. These neurons become active with tissue injury and add to the nociceptive input entering the CNS.26
Under normal conditions it is not believed that the LTM neurons are involved in the transfer of nociception. Nociception is primarily carried by the NS and WDR neurons.
The dorsal horn of the spinal cord is subdivided into different layers, or laminae.27,28,29 These laminae are numbered according to their depth in the dorsal horn from I to VI, with the most superficial being I and the deepest being VI13 (Fig 2-3). Studies suggest that nociceptive input enters the dorsal horn by way of the NS and WDR neurons in the area of laminae I, II, and V.30,31 The LTM neurons that do not carry nociception seem to be more concentrated in laminae III and IV.
Within the dorsal horn there are interneurons that transfer impulses to other interneurons or to the ascending neurons. These neurons can be either inhibitory or excitatory . In other words, when some of the neurons are stimulated, they tend to reduce the activity of the neuron to which they synapse. These are called inhibitory neurons.
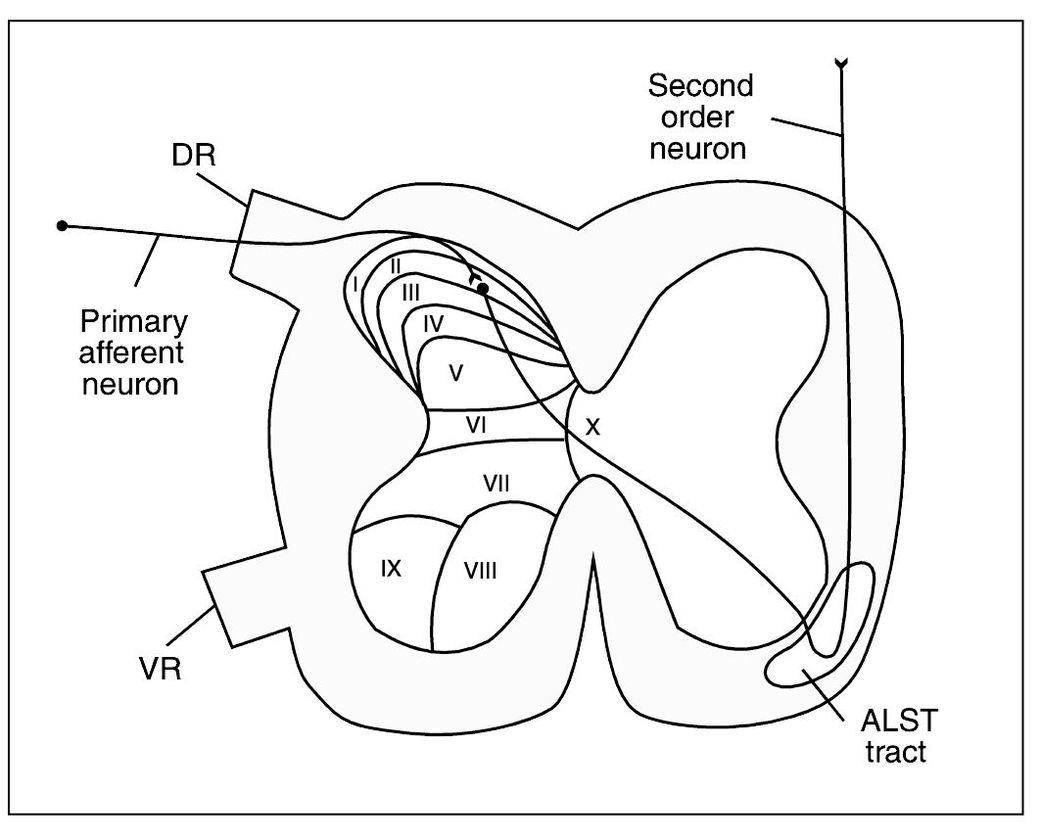
Fig 2-3 Cross section of the spinal cord showing the anatomic laminae I through X of the cord gray matter and the ascending sensory anterolateral spinothalamic tract (ALST tract) in the white columns. DR = Dorsal root; VR = ventral root.
Other interneurons, when excited, increase the activity of the neurons on which they synapse. These are called excitatory neurons. There are a significant population of these interneurons in laminae II and III, and this region is collectively called the substantia gelatinosa.
Once the impulses have been transferred from the primary afferents, most of the second-order neurons cross to the opposite side of the spinal cord and enter the anterolateral spinothalamic tract, which ascends to the higher centers. Some of the second-order neurons remain on the same side of the dorsal column and ascend by way of the lemniscal system. These neurons cross over to the opposite side at the level of the medulla. The dorsal column lemniscal system is composed of large, myelinated nerve fibers that transmit signals to the brain at velocities of 30 to 110 m/s. The anterolateral system is composed of much smaller myelinated and unmyelinated fibers that transmit signals at velocities ranging from a few meters per second up to 40 m/s.
These differences immediately characterize the types of sensory information that can be transmitted by these two systems. The dorsal column lemniscal system rapidly transmits information regarding touch, pressure, vibration, and proprioception needed for immediate response of the musculoskeletal system to environmental changes. The anterolateral system transmits impulses at a slower rate but carries a much broader spectrum of sensory information, such as pain, warmth, cold, and crude tactile sensations.32
Nociceptive input is predominantly carried by the anterolateral system, which is divided into two tracts: the neospinothalamic tract and the paleospinothalamic tract. The neospinothalamic tract carries the A-delta nociceptive inputs directly to the higher centers. The paleospinothalamic tract predominantly carries the slower C-fiber nociception and travels through many other centers before reaching the brain. The significance of these two pathways with regard to “fast” and “slow” pain will be discussed in chapter 3.
Brainstem and Brain
Once the impulses have been passed to the second-order neurons, these neurons carry them to the higher centers for interpretation and evaluation. There are numerous centers in the brainstem and brain that help give meaning to the impulses. It should also be remembered that numerous interneurons may be involved in transmitting the impulses on to higher centers. In fact, attempting to follow an impulse through the brainstem on to the cortex is no simple task. To intelligently discuss pain in this text, certain functional regions of the brainstem and brain will need to be described. The reader should be reminded that the following description will merely be an overview of what are thought to be the most important functions of each area. The complexity of the organ we call the brain is beyond the scope of this text. In fact, it may be beyond the scope of the human mind itself. In a very thought-provoking statement, Thibodeau33 wrote, “Perhaps the capacity of the human brain falls short of the ability to understand its own complexity.”
The higher centers of the CNS can be subdivided into the following four regions, from the most inferior to the most superior:
- The brainstem, which is made up of the medulla oblongata, the pons, and the midbrain (or mesencephalon)
- The cerebellum
- The diencephalon, which is made up of the thalamus and the hypothalamus
- The cerebrum, which is made up of the cerebral cortex, the basal ganglia, and the limbic structures
The figures used throughout this text are graphic representations of the brain and brainstem. They are not meant to accurately depict the anatomy of each area but instead to give the reader an idea of the functional relationship so that pain processing can be better understood (Fig 2-4
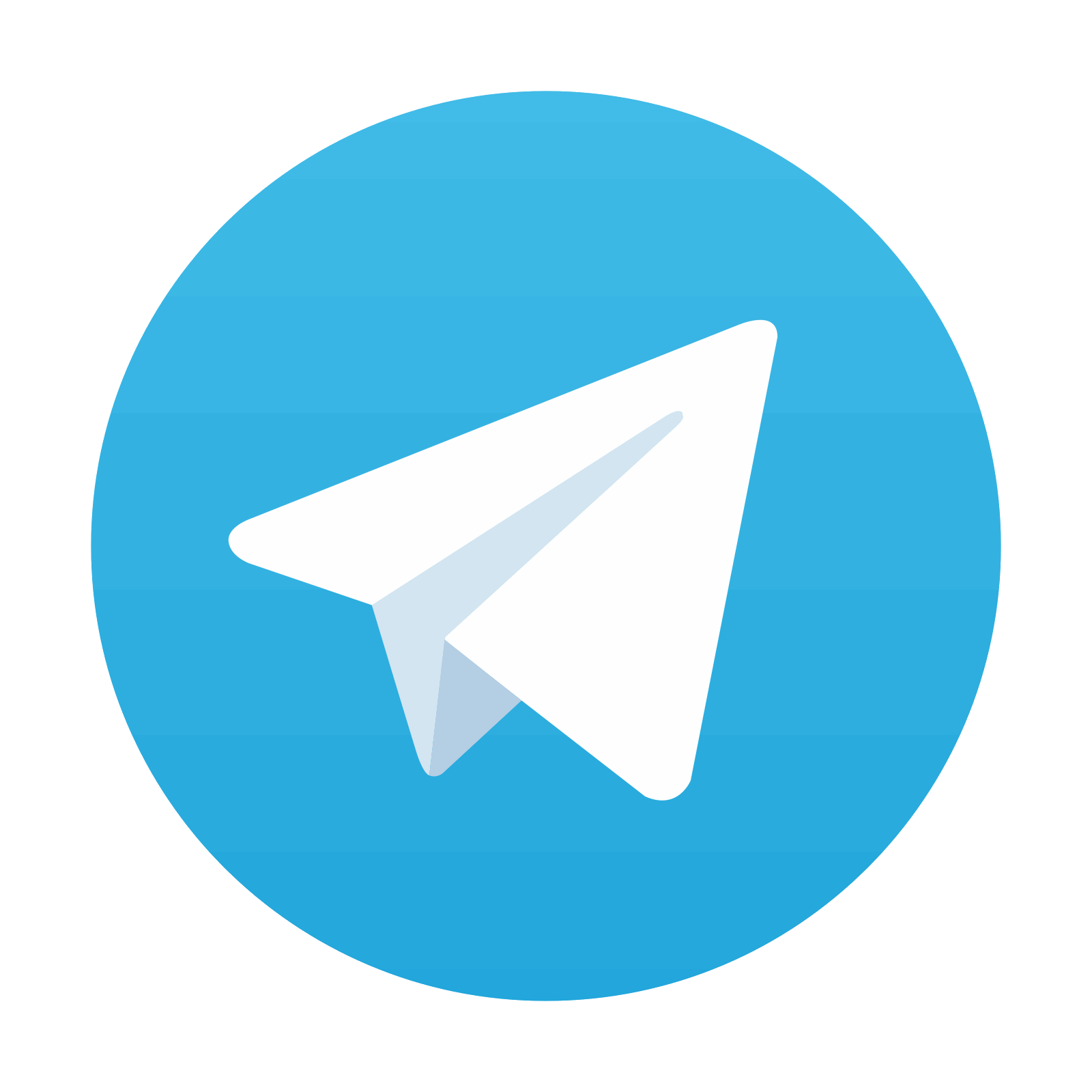
Stay updated, free dental videos. Join our Telegram channel

VIDEdental - Online dental courses
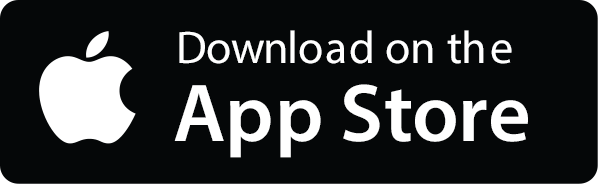
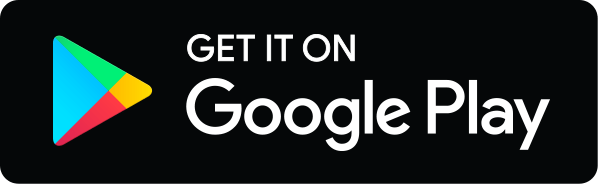